Abstract
To explore the part of molecular mechanism of rhodopsin family genes in the olive flounder (Paralichths olivaceus), we observed the change patterns of the photoreceptors, rhodopsin (RH) and extra-ocular rhodopsin (Exo-RH) genes. We isolated cDNAs of RH and Exo-RH genes from the retina and pineal gland, respectively, of the olive flounder and investigated the differential expression of these genes in these organs during a 28-h time period under the 12-h light:12-h dark (LD) photocycle, constant dark (DD) and constant light (LL). The expression of RH gene in retina showed clear circadian pattern under LD, with the highest level at Zeitgeber time (ZT) 4 and ZT28 and lowest at ZT16. The expression level of Exo-RH gene in pineal organ under LD showed opposite pattern to that of RH, with higher expression during the dark period, and the peak was at ZT20. This expression pattern was similar between in vivo and in vitro (incubated) pineal organs. Under DD and LL conditions, the expression patterns of RH and Exo-RH genes were similar to those under LD photocycle, but the circadian change patterns were less clear. The expression levels of Exo-RH were about 100-fold (LD), 30,000-fold (DD) and 10-fold (LL) lower in pineal organ treated with high concentration of melatonin compared to the untreated pineal organ. The melatonin concentration in plasma had similar circadian pattern with that of the expression of Exo-RH gene, with the peak at ZT20 for fish kept under all LD, DD and LL conditions. Our results suggest that circadian rhythm in the olive flounder is controlled and maintained by the environmental photocycle through the mediation of RH and Exo-RH, and the melatonin concentration in blood plasma.
Introduction
Fish live in aquatic environments with varying light intensity, and they often possess visual pigments with the absorption spectrum adapting to their habitats (Yokoyama et al. Citation1999). Fish that migrate from the pelagic zone to the benthic habitat, or vice versa, during ontogeny change their visual receptors (Helvik et al. Citation2001). The retina at the larval stages consists of only single cone cells; later during metamorphosis double cones and rods develop (Helvik et al. Citation2001). Because fish use environmental light signals for multiple physiological functions such as vision, photoentrainment of circadian rhythms, regulation of body colour and detection of seasonal changes in the photoperiod (Cole and Youson Citation1982), they are useful model species to study the adaption of animals to the environmental light conditions.
Among the factors that influence circadian rhythms (physiological and behavioural changes within a period of 24 h), light is the most important one (Simonneaux and Ribelayga Citation2003; Pierce et al. Citation2008). The biological circadian rhythm usually has a diurnal rhythm that is controlled by the external illumination time, often with seasonal periodicity (Bolliet et al. Citation2001; Pierce et al. Citation2008). The oscillation of the circadian rhythm is driven by an intra-cellular molecular clock and is self-sustaining, i.e. occurring even in the absence of environmental cues (Deguchi Citation1981; King and Takahashi Citation2000; Pierce et al. Citation2008).
Light also controls the expression of the gene encoding rhodopsin (RH), a dim-light photoreceptor belonging to the G protein-coupled receptor (GPCRs) family (Khorana et al. Citation2002). RH is located in rod cells and transduces extracellular light signals into the cells (Yokoyama Citation2000; Terakita Citation2005). It consists of a protein, opsin and a retinal chromophore and is the photoreceptor responding to external light signals in the retina (Deguchi Citation1981; Khorana et al. Citation2002; Minamoto and Shimizu Citation2003). RH has been identified in various animals including fish (Terakita Citation2005). Most fish possess only one RH, with the maximum absorption corresponding to the photic environment (Minamoto and Shimizu Citation2003). There are two ways to tune the absorption spectrum of visual pigments: one is to change the chromophore and the other is by changing the primary amino acid structure of opsin proteins (Munz and McFarland Citation1977). It is reported that only a few important amino acid substitutions can cause a large shift of absorption maximum in vertebrates (Nakayama and Khorana Citation1991; Yokoyama and Radlwimmer Citation1998).
Also, it has been generally considered that one fish has one type of RH, although some fish such as the coelacanth (Yokoyama et al. Citation1999), the ayu (Plecoglossus altivelis) (Hope et al. Citation1998), the puffer fish (Takifugu rubripes) and the Atlantic salmon (Salmo salar) (Philp et al. Citation2000) have multiple RHs.
Recently, extra-ocular rhodopsin (Exo-RH), with a similar amino acid sequence to RH and belonging to RH group in the molecular phylogenetic analysis, was found in some teleosts (Mano et al. Citation1999; Philp et al. Citation2000). These new rhodopsins are expressed in fish pineal glands and considered to be related with non-visual photoreception as well as vertebrate ancient (VA) opsin (Soni and Foster Citation1997; Kojima et al. Citation2000), although their exact physiological functions remain unknown.
Exo-RH, a pineal opsin protein, is expressed solely in the pineal gland (Mano et al. Citation1999; Philp et al. Citation2000), such as the zebrafish (Danio rerio) (Pierce et al. Citation2008), the puffer fish and the Atlantic salmon (Philp et al. Citation2000). Phylogenetic analysis indicates that this opsin belongs to the RH group. Exo-RH has been identified in some vertebrates, including the lamprey, teleosts, amphibians and mammals (Yokoyama and Zhang Citation1997; Mano et al. Citation1999; Philp et al. Citation2000; Zhang et al. Citation2003). Exo-RH has been hypothesised to play an important role in the photoentrainment of the circadian clock (Zatz and Mullen Citation1988; Cahill Citation1996).
A photoreceptive molecule in the pineal gland mediates photoentrainment of the endogenous circadian clock which drives rhythmic production of melatonin (Deguchi Citation1979, Citation1981). The pineal gland also synthesises and secretes melatonin, a nocturnal hormone produced during the scotophase which regulates circadian and seasonal rhythms, including sleeping/waking cycles of vertebrates (Eskin Citation1979).
The circadian rhythm has been studied in various organisms such as fruit flies (Stanewsky et al. Citation1998); they investigated that cryptochrome, period and timeless genes expression for circadian rhythm; and plants (Samach and Coupland Citation2000); the genes related with circadian rhythm might regulate flowering by controlling their expression by the circadian clock; however, only limited studies on this topic have also been done in fish (e.g. golden rabbitfish and zebrafish) (Mano et al. Citation1999; Park et al. Citation2007; Pierce et al. Citation2008), they investigated that changes of circadian rhythm genes, melatonin receptor subtypes and Exo-RH for searching the part of circadian rhythm in fish.
In this study, we explored changes in the mRNA expression of RH and Exo-RH for examining the part of the photic regulation molecular mechanism in the olive flounder, an economically important fish which migrates to benthic environments after inhabiting the pelagic zone during the fry stage. We cloned the full-length cDNAs of RH and Exo-RH genes from the retina and pineal gland of the olive flounder, respectively, and determined the expression level of RH gene in the retina and Exo-RH gene in pineal glands (in vivo and in vitro) during a 28-h time period under the 12-h light:12-h dark (LD) photocycle, constant dark (DD) and constant light (LL) conditions. We found that the expression level of RH in retina was higher during the photophase, while the Exo-RH gene expression level in the pineal gland was higher during the scotophase.
Materials and methods
Experimental fish and conditions
Olive flounders (n=90; length, 15±0.5 cm; weight, 27.5±2.5 g) were obtained from a commercial fish farm. Flounders were transferred into three 300-l circulation filter tanks and acclimated for 2 weeks under LD (lights on 0700–1900 hours, 200 lx during the photophase in the water phase) condition. During the experiments, flounders were placed under LD, DD and LL conditions. They were fed commercial feed twice daily (0900 and 1700 hours). Experimental fish were anesthetised with 200 mg l−1 tricaine methanesulphonate (MS-222; Sigma, St. Louis, MO, USA) before blood was collected from the caudal vein using a 3-ml syringe coated with heparin. Plasma samples were separated by centrifugation (4°C, 10,000×g, 5 min) and stored at −80°C until analysis. The fish were euthanised by spinal transection at 4-h intervals, and the retina and pineal gland were dissected and fixed immediately in Trizol solution (Gibco/BRL, Gaithersburg, MD, USA).
Total RNA extraction, cDNA synthesis and RH/Exo-RH cDNA isolation
Total RNA was extracted from the retina and pineal gland, respectively, using the TRIzol kit 167 (Gibco/BRL, Gaithersburg, MD, USA). The first-strand cDNA was synthesised using M-MLV 168 reverse transcriptase (Bioneer, Daejon, Korea) according to the manufacturer's instructions.
Gene-specific primers were designed for RH and Exo-RH (see ) using highly conserved regions from the Atlantic halibut (Hippoglossus hippoglossus) (GenBank accession no.: AAM17918), common sole (Solea solea) (CAA77254), fire clownfish (Amphiprion melanopus) (ADI59664) and T. rubripes (AF201472). Polymerase chain reaction (PCR) amplification was performed using 2× Taq Premix I (Solgent, Daejeon, Korea) under the following conditions: 95°C for 2 min; 40 cycles of 95°C for 20 s, 56°C for 40 s and 72°C for 1 min; and a final step of 7 min at 72°C.
Table 1. Primers used in this study.
The PCR products were purified and ligated into the pGEM-T Easy Vector (Promega, Madison, WI, USA), and transformed into Escherichia coli DH5a competent cells (RBC Life Sciences, Seoul, Korea). Plasmid DNA was extracted and sequenced using ABI DNA Sequencer (Applied Biosystems, Foster City, CA, USA).
Rapid amplification of cDNA 3′- and 5′-ends (3′- and 5′-RACE) of RH and Exo-RH
For the RH and Exo-RH RACE reactions, 2.5 g total RNA extracted from the retina and pineal gland, respectively, was used to synthesise the first-strand 5′- and 3’-RACE cDNAs using a CapFishing™ Full-length cDNA Premix Kit (Seegene, Seoul, Korea) following the manufacturer's protocol.
RH- and Exo-RH-specific primers were designed () based on the partial sequences obtained above and paired with 5′-RACE target primer (5′-GTC TAC CAG GCA TTC GCT TCA T-3′) or 3′-RACE target primer (5′-CTG TGA ATG CTG CGA CTA CGA T-3′). The 5′-and 3′-RACE cDNAs were used as the template for the 5′- and 3′-end RACE reactions, respectively. PCR was carried out as follows: 94°C for 5 min; 35 cycles of 94°C for 40 s, 62°C for 40 s and 72°C for 1 min; and a final step of 5 min at 72°C. PCR amplicons were cloned and sequenced as described earlier.
Phylogenetic analysis
RH and Exo-RH phylogenetic analysis was conducted using RH and Exo-RH amino acid sequences from the following species that were aligned using BioEdit software (Hall Citation1999): Atlantic halibut RH (GenBank accession no. AAM17918), common sole RH (GenBank accession no. CAA77254), flathead mullet (Mugil cephalus) RH (GenBank accession no. CAA77250), winter flounder (Pseudopleuronectes americanus) RH (GenBank accession no. AAT72123), fire clownfish Exo-RH (GenBank accession no. ADI59664), Fugu rubripes Exo-RH (GenBank accession no. AF201472), ayu Exo-RH (GenBank accession no. BAC56700) and Atlantic salmon Exo-RH (GenBank accession no. AAF44619). The phylogenetic tree was constructed using the neighbour-joining method with the Mega 3.1 software package (Center for Evolutionary Functional Genomics, Tempe, AZ, USA).
Tissue distribution of RH and Exo-RH mRNA
To examine the tissue distribution of RH and Exo-RH mRNA, total RNA was extracted from the nervous and peripheral tissues. The brain, pineal gland, pituitary, retina, gill, liver and kidney were removed and immediately stored at −80°C. Total RNA was extracted from the tissues using a TRIzol kit (Gibco/BRL, Gaithersburg, MD, USA). Reverse transcription (RT) was performed using M-MLV reverse transcriptase (Bioneer, Daejon, Korea) according to the manufacturer's instructions. PCR amplification was performed with the following primer sets: RH forward primer (5′-AGG AGT CCT TAT GAG TAC CC-3′), RH reverse primer (5′-CTT GAC AGC ACA GAG CAG-3′), Exo-RH forward primer (5′-TTG ATC ACC CGC AGT ACT AC-3′) and Exo-RH reverse primer (5′-AGG AGA TCA CCA TGA CGA TG-3′). PCR were performed with 2× Taq Premix I (Solgent, Daejon, Korea) under the following conditions: 95°C for 2 min; 40 cycles of 95°C for 20 s, 56°C for 40 s and 72°C for 1 min; followed by a final step of 7 min at 72°C. Amplification of β-actin mRNA was also conducted to verify the quality of the RT products using a primer set for olive flounder β-actin cDNA: β-actin forward primer (5′-TCG AGC ACG GTA TTG TGA CC-3′) and β-actin reverse primer (5′-ACG GAA CCT CTC ATT GCC GA-3′). The amplified PCR products were electrophoresed on 1% agarose gels, stained with ethidium bromide and visualised by illumination with UV light.
Determination of transcript levels of RH and Exo-RH using real-time transcription quantitative PCR (RT-qPCR)
Duplicate retina and pineal organ samples were collected at 4-h intervals during a 28-h period. The first-strand cDNA was prepared as described earlier and used as the template for RT-qPCR.
RT-qPCR was performed with gene-specific primers designed based on the RH and Exo-RH cDNA sequences of the olive flounder () using a BIO-RAD iCycler iQ Multicolor Real-time PCR Detection System (Bio-Rad, California, USA) and iQ™ SYBR Green Supermix (Bio-Rad), according to the manufacturer's instructions under the following condition: 95°C for 5 min followed by 40 cycles of 95°C for 20 s and 55°C for 20 s.
To confirm that the cDNAs for the diel samples were free from contaminating genomic DNAs, a negative control of RNA was prepared for use in RT-qPCR in the same way as cDNAs, but omitting reverse transcriptase. To avoid the potential error of RT-qPCR caused by poor RNA quality, β-actin (HQ386788) and glyceraldehyde-3-phosphate dehydrogenase (GAPDH) (AB029337) were used as the internal controls. The expression level of the genes in each cDNA sample was analysed based on the corresponding threshold cycle (Ct) values of gene in RT-qPCR. The Ct level was defined as the PCR cycle at which the fluorescence signal crossed the threshold line in the exponential phase of the amplification curve. The expression level of RH and Exo-RH was normalised to the average of the Ct of β-actin and GAPDH. The calibrated ΔCt value (ΔΔCt) for each sample and internal controls (β-actin and GAPDH) were calculated [ΔΔCt = 2ˆ−(ΔCtsample − ΔCtinternal control)]. The data were analysed using the delta − delta method (Livak and Schmittgen Citation2001).
In vitro culture of the pineal gland and melatonin treatment
After the fish were anesthetised, the pineal gland was dissected and placed in ice-cold medium (pH 7.5) composed of 150 mM NaCl, 3.5 mM KCl, 1.0 mM CaCl2·H2O, 1.0 mM MgCl2·6H2O, 0.7 mM NaH2PO4·2H2O, 7.0 mM NaHCO3, 2.8 mM glucose, 1.0 mM MgCl2, 10 mM HEPES and 0.88 g l−1 Eagle's MEM (Sigma) containing antibiotics (0.06 g l−1 penicillin and 0.1 g l−1 streptomycin; Penicillin-Streptomycin, Gibco, USA). The pineal gland was transferred to a 24-well culture plate containing 1 ml of medium and incubated at 20±1°C in an incubator under light/dark (LD) (LD12:12, light switched on at 0700 hours), DD and LL conditions. The light intensity at the surface of the culture plate was approximately 700 lx during the photophase. The pineal gland and medium were sampled at 4-h intervals (n=5 − 6) from ZT4 to ZT28, and each sample was centrifuged (20°C, 10,000×g, 15 s) to separate the pineal gland and medium. Total RNA was then extracted from the pineal gland. The pineal gland culture medium (supernatant) was stored for measuring melatonin, and the tissue was stored at −80°C until RNA extraction.
Melatonin (Sigma) dissolved in ethanol was added to the culture medium in the ratio 1/1000e (v/v), and the indicated concentrations of melatonin (10 M) were obtained. Melatonin treatments were given at 0700 hours (ZT0). Cells were treated for 4 or 28 h without renewing the medium. Each sample was centrifuged (20°C, 10,000×g, 15 s), and the supernatant was removed and stored at −80°C until RNA extraction.
Melatonin determination in plasma and the pineal gland culture medium by ELISA
Immunoenzymoassay with a commercial enzyme-linked immunosorbent assay (ELISA) kit (IBL, Hamburg, Germany) was used to determine the melatonin concentration in the plasma. Plasma and the pineal gland culture medium samples were purified through extraction columns immediately after defrosting in the centrifuge. Then, 50 l of each sample was added to different wells of an ELISA plate pre-coated with the capture antibody, anti-rabbit IgG (goat, polyclonal). The samples were incubated with melatonin-biotin and antiserum solutions for 15 h at 4°C. The wells were then washed with the assay buffer (phosphate buffer with Tween-20 and stabiliser), and the plate was incubated with the enzyme-labelled solution (anti-biotin-alkaline phosphatase in Tris buffer with stabilisers) for 2 h at room temperature with constant shaking. After the plate was washed, it was incubated with p-nitro-phenyl phosphate solution for 30 min, and 50 l of stop solution (1 N NaOH with 0.25 M ethylene diamine tetraacetic acid) was then added to it. Absorbance was read at 405 nm. According to the manufacturer of the ELISA kit, the intra- and inter-assay coefficients of variation were 3.9 − 6.9% in the range of 28.8–266 pg ml−1 and 6.2–15.9% in the range of 3.5 − 281 pg ml−1, respectively.
Statistical analysis
All data were analysed using the SPSS statistical package (version 10.0; SPSS Inc., Chicago, USA). One-way ANOVA followed by Tukey's post hoc test was used to assess statistically significant differences among the different time points of diurnal and circadian variation. A value of p < 0.05 was considered statistically significant. If necessary, values were transformed (logarithmic or square root transformations) to fulfil the conditions of the parametric analysis of variance ANOVA (normal distribution and homogeneity of variances). A value of p<0.05 was considered statistically significant.
Results
Full-length cDNA sequences of RH and Exo-RH
The expected amplicons for both RH and Exo-RH were obtained by RT-PCR with gene-specific forward and reverse primers and were cloned and sequenced. A 572- and 397-bp sequence were proved to be part of the RH and Exo-RH genes, respectively. On comparing the sequence of olive flounder, RH and Exo-RH with those deduced from the RH and Exo-RH cDNAs of other teleost species. Using the RACE method, the full-length cDNA sequences for both the RH (1059) bp [GenBank accession no.: HQ413772]) and Exo-RH (1059) bp [HM107825]) of the olive flounder were obtained, both of which were predicted to encode a protein of 352 amino acid (aa) residues. Olive flounder RH and Exo-RH showed high aa sequence similarity to other teleosts, Atlantic halibut, common sole, flathead mullet, winter flounder, fire clownfish, F. rubripes, ayu and Atlantic salmon (93–95% for RH and 87–96% for Exo-RH). Also, they shared common 70% aa sequence similarity with each other ( and ), as a member of the RH family (a family including RH and Exo-RH) has a similar aa structure. The aa residues that are important for the function of RH were found to be conserved in both RH and Exo-RH. Examples are: the lysine residue (□) in the putative seventh transmembrane domain that attaches to the chromophore by Schiff base linkage (Wang et al. Citation1980); the counterion glutamic acid residue (Glu-113) in the predicted transmembrane domain III (Sakmar et al. Citation1989); the cysteine residues Cys-110 and Cys-187, which may form a disulphide bridge; and the palmitoylation sites Asn-302 and Pro-303 (Asn-303 and Pro-304 in Exo-RH), which are thought to be important in anchoring RH to the cell membrane by palmitic acid esterification (Ovchinnikov et al. Citation1988) ( and ). For Exo-RH, the transmembrane embedded residues of the α-helices were predicted according to Baldwin's model (Baldwin et al. Citation1997).
Figure 1. Alignment of the amino acid sequences of olive flounder RH isolated in this study with those from the GenBank/EMBL/DDBJ databases. The GenBank accession numbers for the sequences used in the alignment are as follows: olive flounder (ofRH, HQ413772), Atlantic halibut (ahRH, AAM17918), common sole (csRH, CAA77254), flathead mullet (fmRH, CAA77250) and winter flounder (wfRH, AAT72123). The seven transmembrane domains are indicated by boxes. Amino acid residues involved in Schiff base formation (Phe, □) and their counterions (Thr, ▪), those involved in disulphide bond formation (Cys, *), those involved in glycosylation (glutamate residue; Gly, Δ) and those involved in palmitoylation (Asn and Pro, •) are indicated. Amino acids involved in the spectral tuning of rhodopsin are also indicated (Ala, ○).
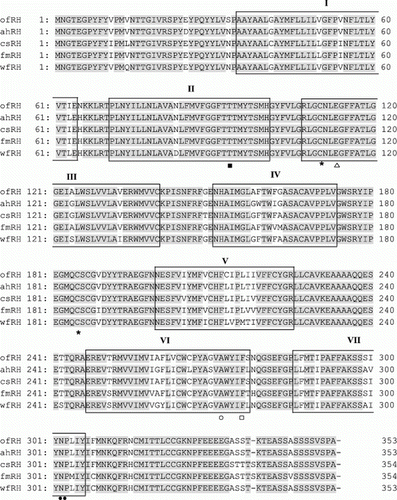
Figure 2. Alignment of the amino acid sequences from olive flounder Exo-RH isolated in this study with those from the GenBank/EMBL/DDBJ databases. The GenBank accession numbers for the sequences used in the alignment are as follows: olive flounder (ofExo-RH, ADI59669), fire clownfish (fcExo-RH, ADI59664), Fugu rubripes (frExo-RH, AF201472), ayu (ayExo-RH, BAC56700) and Atlantic salmon (asExo-RH, AAF44619). Putative transmembrane domains I–VII are indicated by horizontal lines. The conserved functional opsin features include glycosylation sites (Asn, Δ), a disulphide bridge (Cys, *), a chromophore attachment site (Lys, □), a Schiff base counterion (Glu, ▪); and a palmitoylation site (Asn and Pro, •).
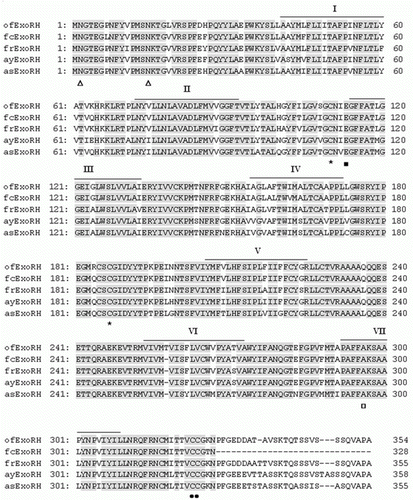
Phylogenetic analysis
The phylogenetic tree obtained by Clustal analysis of the sequences described in this section is shown in . Olive flounder RH and Exo-RH are grouped with other retinal RH and Exo-RH molecules, and separated into a retinal RH group and an Exo-RH group. In this tree, olive flounder RH was clustered in the retinal RH group, and olive flounder Exo-RH was clustered in the extra-ocular RH group.
Figure 3. Phylogenetic tree based on an amino acid alignment for RH and Exo-RH sequences in teleost fish. Bootstrap values (%) are indicated (1000 replicates). The score between two protein sequences, which is a measure of their relative phylogenetic relationship, is represented by the horizontal distance in this tree, i.e. the shorter the distance, the more related they are. GenBank accession numbers for the sequences are: Atlantic halibut RH (ahRH, AAM17918); common sole RH (csRH, CAA77254); flathead mullet RH (fmRH, CAA77250); winter flounder RH (wfRH, AAT72123); fire clownfish Exo-RH (fcExo-RH, ADI59664); Fugu rubripes Exo-RH (frExo-RH, AF201472); ayu Exo-RH (ayExo-RH, BAC56700); and Atlantic salmon Exo-RH (asExo-RH, AAF44619).
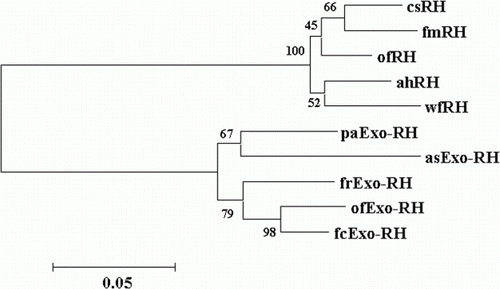
Tissue distribution of RH and Exo-RH mRNA
We examined the tissue distribution of RH and Exo-RH mRNA by RT-PCR (). RH was predominantly expressed in the retina and slightly expressed in the peripheral tissues. Exo-RH was significantly expressed in the pineal gland and also expressed at a high level in the retina.
Diurnal and circadian variation in the expression of RH mRNA in the retina
We examined the diurnal variation in the expression of RH mRNA in the retina by QPCR (). RH mRNA was expressed at significantly higher levels during the photophase than the scotophase, and its expression peaked between ZT28 and ZT4. At the start of the scotophase, the RH expression levels were significantly low, and even lower levels were observed at midnight (ZT16) (a). Further, we found that at ZT16, RH mRNA expression was lower under DD than LD, but the amplitude was attenuated (b). Also, under LL condition, the expression was rhythmic as under LD and DD, but expression levels were significantly higher than LD and DD conditions about 1.2 to 1.5 times, and the amplitude was attenuated (c).
Figure 5. Diurnal variations in the levels of RH mRNA in the retina of the olive flounder as measured by quantitative real-time PCR. The fish were reared under a 12:12 light:dark (LD) cycle (a), constant dark (DD) (b) and constant light (LL) (c). Total retinal RNA (2.5 g) was reverse transcribed and amplified. The results are expressed as normalised expression levels with respect to the β-actin and GAPDH levels in the same sample. The white bar represents the photophase and the black bar, the scotophase. Different letters indicate that values are statistically different in Zeitgeber time (ZT) and Circadian time (CT) (p < 0.05). All values represent means±SD (n = 5).
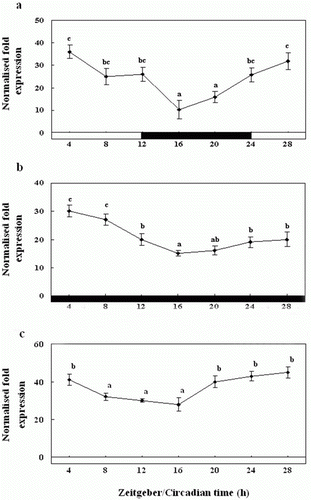
Diurnal and circadian variation in the expression of Exo-RH mRNA in the pineal gland examined in vivo
To examine the circadian rhythm in the flounder, we examined the diurnal variation in Exo-RH mRNA expression in the pineal gland by QPCR (). Exo-RH expression levels were found to be high at the start of the scotophase (ZT12), and significantly higher levels were observed during the scotophase than the photophase (a). Diurnal changes in Exo-RH expression were observed with a single peak at ZT20, and then the expression decreased at end of the scotophase (ZT24). Further, under DD condition, Exo-RH expression was rhythmic as under LD, with peaks at ZT20, but the amplitude was lower (b). Also, under LL condition, the expression was rhythmic as under LD and DD, but expression levels were significantly lower than LD and DD about 12,500 times, with peaks at ZT16, and the amplitude was lower (c).
Figure 6. Diurnal variations in the levels of Exo-RH mRNA in the pineal gland in vivo as measured by quantitative real-time PCR. The fish were reared under a 12:12 light:dark (LD) cycle (a), constant dark (DD) (b) and constant light (LL) (c). Each mean value and error bar indicates the pineal gland from 10 fish. Total pineal gland RNA (2.5 g) was reverse transcribed and amplified. The results are expressed as the normalised expression levels with respect to the levels of β-actin and GAPDH in the same sample. The white bar represents the photophase and the black bar, the scotophase. Different letters indicate that values are statistically different in Zeitgeber time (ZT) and Circadian time (CT) (p < 0.05). All values represent means±SD (n = 5).
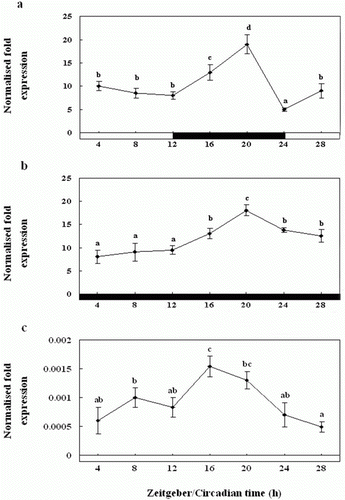
Diurnal and circadian variation in the expression of Exo-RH mRNA in the pineal gland examined in vitro
In the cultured pineal gland, Exo-RH mRNA expression was found to be high at midnight (ZT16), and the levels were significantly higher during the scotophase than the photophase (a). The expression showed diurnal changes with one peak at ZT20 and then decreased at the end of the scotophase (ZT24). Further, under DD condition, Exo-RH expression in the cultured gland was as rhythmic as that under LD, with peaks at ZT20, but the amplitude was lower (b). Also, under LL condition, the expression was rhythmic as under LD and DD, but expression levels were significantly lower than LD and DD about 8000–12,000 times, with peaks at ZT16, and the amplitude was lower (c).
Figure 7. Diurnal variations in the levels of Exo-RH mRNA in the cultured pineal gland in vitro as measured by quantitative real-time PCR. The pineal gland was maintained under a 12:12 light:dark (LD) cycle (a), constant dark (DD) (b) and constant light (LL) (c). Each mean value and error bar indicates the pineal gland from 10 fish. Total pineal gland RNA (2.5 g) was reverse transcribed and amplified. The results are expressed as the normalised expression levels with respect to the levels of β-actin and GAPDH in the same sample. The white bar represents the photophase and the black bar, the scotophase. Different letters indicate that values are statistically different in Zeitgeber time (ZT) and Circadian time (CT) (p < 0.05). All values represent means±SD (n = 5).
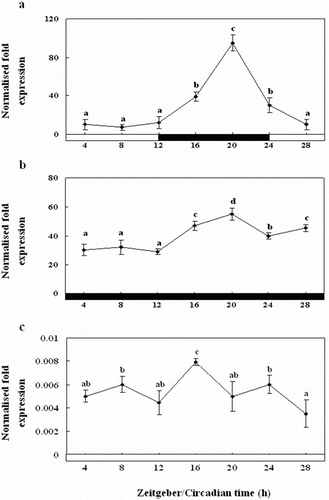
Diurnal and circadian variation in the expression of Exo-RH mRNA in the melatonin-treated pineal gland examined in vitro
Exo-RH mRNA expression was high at midnight (ZT20) in the cultured melatonin-treated pineal gland, and the levels were significantly higher during the scotophase than the photophase (a). The expression showed diurnal changes with one peak at ZT20 and then decreased at the end of the scotophase (ZT24). Similar results as Exo-RH mRNA expression in the non-treated pineal gland were observed with regard to the DD condition, and showed one peak at ZT16 (b). Further, under LD and DD, the Exo-RH expression levels were significantly lower in the melatonin-treated gland than in the non-treated one. Also, under LL condition, the expression was rhythmic as under LD and DD, but expression levels were significantly lower than LD and DD about 1.25–25 times, with peaks at ZT20, and the amplitude was lower (c).
Figure 8. Diurnal variations in the levels of Exo-RH mRNA in the cultured melatonin-treated in vitro, as measured by quantitative real-time PCR. The pineal gland was maintained under a 12:12 light:dark (LD) cycle (a), constant dark (DD) (b) and constant light (LL) (c). Each mean value and error bar indicates the pineal gland from 10 fish. Total pineal gland RNA (2.5 g) was reverse transcribed and amplified. The results are expressed as the normalised expression levels with respect to the levels of β-actin and GAPDH in the same sample. The white bar represents the photophase and the black bar, the scotophase. Different letters indicate that values are statistically different in Zeitgeber time (ZT) and Circadian time (CT) (p < 0.05). All values represent means±SD (n=5).
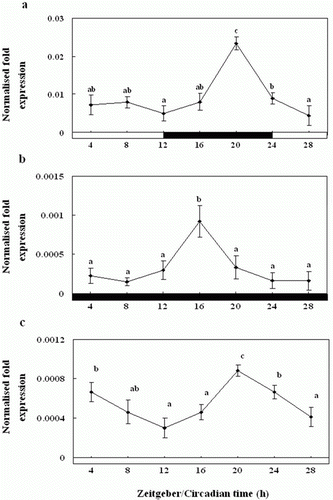
Diurnal and circadian variation in the pineal gland culture medium melatonin levels
We examined the melatonin levels in the pineal gland culture medium exposed to the normal 24-h LD cycle. The pineal gland culture medium melatonin concentrations were significantly higher during the scotophase (12.5±0.6 pg ml−1) than the photophase (3.5±0.5 pg ml−1) (a). Further, the pineal gland culture medium melatonin levels under the DD condition were similar to those under the 24 h LD cycle, although they were of a lower amplitude; they peaked significantly at ZT20 (12.0±1.0 pg ml−1) (b).
Figure 9. Enzyme-linked immunosorbent assay of the melatonin levels of the pineal gland culture medium during the daily light:dark (LD) cycle (12:12) (a), constant dark (DD) (b) and constant light (LL) (c). The white bar represents the photophase and the black bar, the scotophase. Different letters indicate that values are statistically different in Zeitgeber time (ZT) and Circadian time (CT) (p < 0.05). All values represent means±SD (n = 5).
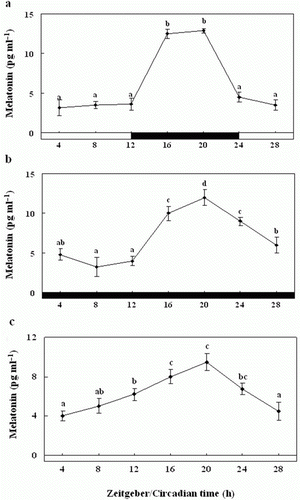
Also, under LL condition, the levels were rhythmic as under LD and DD, but levels were significantly lower than LD and DD about 1.33 times, with peaks at ZT16 (8±0.7 pg ml−1) and ZT20 (9.5±0.9 pg ml−1), and the amplitude was lower (c).
Diurnal and circadian variation in plasma
melatonin levels
We examined the plasma melatonin levels in the olive flounders exposed to the normal 24-h LD cycle. Plasma melatonin concentrations were significantly higher during the scotophase (16.1±1.9 pg ml−1) than the photophase (5.2±2.1 pg ml−1) (a). Further, the plasma melatonin levels under the DD condition were similar to those under the 24 h LD cycle, although they were of a lower amplitude; they peaked significantly at ZT20 (10.0±1.1 pg ml−1) (b). Also, under LL condition, the levels were rhythmic as under LD and DD, but levels were significantly lower than LD and DD about 1.5 − 2.5 times, with peaks at ZT20 (6.5±0.3 pg ml−1) and ZT24 (6.7±0.4 pg ml−1), and the amplitude was lower (c).
Figure 10. Enzyme-linked immunosorbent assay of plasma melatonin levels of olive flounders during the daily light:dark (LD) cycle (12:12) (a), constant dark (DD) (b) and constant light (LL) (c). The white bar represents the photophase and the black bar, the scotophase. Different letters indicate that values are statistically different in Zeitgeber time (ZT) and Circadian time (CT) (p < 0.05). All values represent means±SD (n = 5).
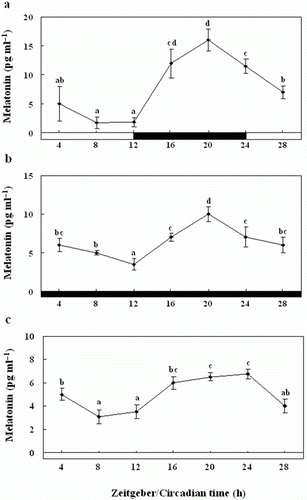
Discussion
We investigated the changes in the mRNA expression of RH and Exo-RH for examining the part of the photic regulation molecular mechanism in the olive flounder using the photoreceptors, RH and Exo-RH. Also, the relationship between the detection of external light and circadian rhythm by cloning full-length RH and Exo-RH cDNA and examining the changes in the expression of the RH family genes encoding these pigments. Importantly, we investigated the expression of Exo-RH mRNA in cultured pineal glands to determine whether it is specifically expressed in the pineal gland during the circadian cycle.
An extra-retinal opsin photoreceptor has been identified in the pineal gland of some vertebrates (Hope et al. Citation1998; Philp et al. Citation2000), but its physiological function has not been studied.
Molecular phylogenetic analysis indicated that olive flounder RH, which is expressed in the retina, and Exo-RH, which is expressed in the pineal gland, clustered with the vertebrate RH and Exo-RH groups, respectively (). However, Exo-RH, phylogenetically separated from the RH groups as a member of the RH family (a family including RH and Exo-RH), has a similar aa structure to RH. This tree agrees with the findings of Minamoto and Shimizu (Citation2003), who showed that RH and Exo-RH, separated from the RH groups were phylogenetically very similar, according to phylogenetic analysis of ayu RH and Exo-RH. In addition, Zhang et al. (Citation2003) reported that Exo-RH had evolved from RH, as determined by the divergence of functional regulatory elements that resulted in its specific expression in the pineal gland.
In this study, we observed the differential expression of RH and Exo-RH mRNA in the nervous and peripheral tissues of olive flounder by RT-PCR analysis: RH is expressed predominantly in the retina, while Exo-RH is strongly expressed in the pineal gland. However, these genes were slightly expressed in the peripheral tissues, it indicated that RH and Exo-RH were specific to retina and pineal gland, respectively (). According to these results, we examined whether RH accepts the photic information in the retina and RH is retinal visual pigments responsible for light capture and initiation of the photo-transduction cascade in rod cells, and if Exo-RH plays a central role in the processing of photic information transduced by RH to control the circadian rhythm in the olive flounder. In addition, Exo-RH is also significantly expressed in the retina, and given the structural homology of Exo-RH and RH, it may function as a photoreceptor (Mano et al. Citation1999; Philp et al. Citation2000; Zhang et al. Citation2003). Considering these results, we suggest that RH and Exo-RH accept the photic information and control the circadian rhythm.
We hypothesise that both RH and Exo-RH receive photic information and control circadian rhythm. Thus, we investigated the relationship between the detection of external light and circadian rhythm by examining changes in the expression of RH family genes, e.g. RH and Exo-RH. Exo-RH can function as an extra-retinal photoreceptor, e.g. by resetting the circadian rhythm during the photoperiod, and RH controls circadian rhythm under LD condition (Schantz et al. Citation1999). When the olive flounder was exposed to LD in this study, RH was expressed at a significantly higher level during the photophase and was expressed at a lower level during the scotophase (a). Under the DD and LL conditions, RH mRNA expression exhibits circadian rhythmicity as under LD condition (b, c). These results suggest that the retina may contain a functional light-entrainable circadian oscillator that synchronises RH expression levels in a circadian rhythm. In addition, light down-regulates RH expression, attenuating transcription and amplitude, but not the period (Im et al. Citation2007). RH is known to be a dim-light photoreceptor that is predominantly expressed during the night than during the day, when there is light (Kim et al. Citation2007). Unfortunately, previous studies in fish, like the cuttlefish (Sepia officinalis), European eel (Anguilla anguilla L.) and skate (Raja erinacea and Raja ocellata), were general studies that merely cloned RH cDNA (O'Brien et al. Citation1997; Bellingham et al. Citation1998; Hope et al. Citation1998), and few studies have investigated the expression levels of RH mRNA during diurnal rhythm (Yokoyama Citation2000; Im et al. Citation2007).
To examine the roles played by Exo-RH as a putative photoreceptor of the pineal gland and as a controller of circadian rhythm, we assessed its expression levels in the pineal gland during diurnal rhythm. Exo-RH expression was significantly increased at the start of the scotophase, ZT12 and peaked during the night at ZT20 (a). These observations are in agreement with the findings of Pierce et al. (Citation2008), who observed a peak of Exo-RH mRNA expression in the zebrafish pineal gland during the scotophase. These results are also supported by another study which indicated that Exo-RH is related to melatonin, a key hormone for the control of circadian rhythm (Zatz and Mullen Citation1988). Collectively, our results indicate that Exo-RH plays a central role in the control of circadian rhythm at night.
In this study, we compared the expression of Exo-RH mRNA between the pineal gland and retina and observed that Exo-RH is strongly expressed in the former. This result is in agreement with the findings of Zatz and Mullen (Citation1988) and Cahill (Citation1996), who reported that Exo-RH and RH have a similar function, but Exo-RH controls the biological clock and melatonin secretion during the photoperiod, whereas retinal RH is expressed in dim light.
We also investigated the expression of Exo-RH in cultured pineal glands in relation to the diurnal rhythm. Exo-RH mRNA expression was high at the start of the scotophase, at ZT12, and then peaked during the night, at ZT20, similar to the in vivo results. Mano et al. (Citation1999) examined that expression of Exo-RH in the pineal gland, and Cahill (1996) reported that Exo-RH may play an important role for photoentrainment of the circadian clock and/or for acute regulation of melatonin production, whereas retinal RH serves for twilight vision. Therefore, we hypothesised that Exo-RH mRNA is expressed at night along with melatonin to control circadian rhythm. Cahill (Citation1996) reported that the biological clock of the pineal gland responds to light and controls the secretion and suppression of melatonin depending on the presence or absence of light, whereby the expression of genes related to the control of circadian rhythm is controlled.
We also examined Exo-RH mRNA expression in pineal glands (in vivo and in vitro) under DD condition and found that its expression is rhythmic, as under LD condition, with peaks at ZT20. We observed the difference of expression levels between in vivo and in vitro (In vitro group, expression levels were significantly higher about 3–5 times than in vivo group), we hypothesised that Exo-RH mainly expresses in pineal gland and there are very close relationship between Exo-RH and pineal gland. Furthermore, under DD and LL conditions, amplitude was lower but it was rhythmic as under LD. It appears to be regulated in a circadian manner (Takemura et al. Citation2006), since all conditions showed similar variation in Exo-RH mRNA expression. It is likely that Exo-RH mRNA expression in all conditions is under circadian regulation (Park et al. Citation2007). Also, Exo-RH expression levels under LL condition were significantly lower than LD and DD conditions, this result is in agreement with the findings of Iigo et al. (Citation1995) and Park et al. (Citation2007), who reported that the rhythm of melatonin-binding sites in the brain diminished after exposure to LL condition in goldfish, and MT1 mRNA expression levels under LL condition were significantly lower than control in the golden rabbitfish (Siganus guttatus), respectively. Therefore, we hypothesised that Exo-RH is related to melatonin, a key hormone for the control of circadian rhythm in night (Zatz and Mullen Citation1988), so Exo-RH mRNA expression levels were very low under LL condition.
Also, Exo-RH mRNA expression in the cultured melatonin-treated pineal gland under DD condition was rhythmic, as under LD condition, with a peak at ZT20, but the expression level was significantly lower than in the cultured pineal gland not treated with melatonin. In addition, Exo-RH mRNA expression under LL condition was rhythmic, as under LD and DD conditions, with a peak at ZT20 and expression level was similar with DD condition. Skene et al. (Citation1996) reported that melatonin feeds back to regulate the primary circadian clock located in the SCN and thus serves as a strong entraining factor. Therefore, we treated the pineal glands with exogenous melatonin to examine the feedback function of decreasing Exo-RH expression levels through results of LL and DD conditions.
Plasma melatonin levels showed rhythmic changes under DD and LL conditions like they did under LD condition, with a peak at ZT20. Furthermore, the melatonin levels of the pineal gland culture medium showed the results similar with plasma melatonin levels. Therefore, we examined that the melatonin is directly secreted from pineal gland. This result is in agreement with the findings of Vera et al. (Citation2010), who measured the levels of melatonin in the cultured pineal gland of the Atlantic cod and Atlantic salmon. They observed significantly higher melatonin levels in the night, indicating the control of circadian rhythm by the nocturnal production of melatonin. The teleost pineal gland contains an intrinsic light-sensitive circadian oscillator that regulates daily rhythms of genes, related with circadian rhythm, expression and melatonin production (Cahill Citation1996; Begay et al. Citation1998; Gothilf et al. Citation1999; Ziv et al. Citation2005). Takemura et al. (Citation2004) found that the exposure of the golden rabbitfish to the ‘brightness’ of midnight during the full and new moon periods resulted in a rapid decrease in the melatonin concentration in the blood circulation. Similarly, the exposure of the cultured pineal gland of the golden rabbitfish to the full moon or new moon periods suppressed melatonin synthesis (Takemura et al. Citation2006). Therefore, it is likely that Exo-RH mRNA expression in the pineal gland is under circadian regulation both in vivo and in vitro. In addition, the level of Exo-RH mRNA expression under DD and LL conditions was significantly lower than under LD condition, indicating that light is an important factor affecting circadian rhythm, and melatonin actions by photoperiod in natural habitats as well as by endogenous clocks. Although there may be differences in circadian rhythm between fish and other vertebrates, these findings may be analogous to the mechanism in several other vertebrates, where attenuation and/or displacement under prolonged constant conditions are typical attributes of many clock-regulated rhythms in which light acts as the main environmental input to adjust rhythmic patterns on a daily basis (Karaganis et al. Citation2009). Further, these results are in agreement with the RH expression levels observed under DD and LL conditions in this study, on the basis of which we suggest that while the circadian rhythm pattern in fish is maintained under LD, DD and LL conditions, thereby hampering the transcription of genes involved in circadian rhythm regulation, like Exo-RH. Therefore, light is an important factor that controls circadian rhythm. These results coincide with those of Ikegami et al. (Citation2009) and Park et al. (Citation2007).
In conclusion, our result suggests that RH and Exo-RH potentially mediates the effects of environmental photocycle on pineal circadian rhythms and melatonin synthesis. Also, Exo-RH, as a member of the RH family, is expressed depending on the light-dependent increase in RH expression. We hypothesise that Exo-RH controls circadian rhythm by increasing its levels in the pineal gland, an important organ for the generation and maintenance of circadian rhythm. Furthermore, additional studies will be necessary to understand the molecular base of photic regulation in fish.
Acknowledgements
This study was supported by the Technology Development Program for Fisheries, Ministry for Food, Agriculture, Forestry and Fisheries, Korea, and by the ITRC support program supervised by the National IT Industry Promotion Agency (NIPA-2012-C1090-1221-0015).
References
- Baldwin , JM , Schertler , GF and Unger , VM . 1997 . An alpha-carbon template for the transmembrane helices in the rhodopsin family of G-protein-coupled receptors . Journal of Molecular Biology , 272 : 144 – 164 .
- Begay , V , Falcon , J , Cahill , GM , Klein , DC and Coon , SL . 1998 . Transcripts encoding two melatonin synthesis enzymes in the teleost pineal organ: circadian regulation in pike and zebrafish, but not in trout . Endocrinology , 139 : 905 – 912 .
- Bellingham , J , Morris , AG and Hunt , DM . 1998 . The rhodopsin gene of the cuttlefish Sepia officinalis: sequence and spectral tuning . The Journal of Experimental Biology , 201 : 2299 – 2306 .
- Bolliet , V , Aranda , A and Boujard , T . 2001 . Demand-feeding rhythm in rainbow trout and European catfish: synchronisation by photoperiod and food availability . Physiology & Behavior , 73 : 625 – 633 .
- Cahill , GM . 1996 . Circadian regulation of melatonin production in cultured zebrafish pineal and retina . Brain Research , 708 : 177 – 181 .
- Cole , WC and Youson , JH . 1982 . Morphology of the pineal complex of the anadromous sea lamprey, Petromyzon marinus L . American Journal of Anatomy , 165 : 131 – 163 .
- Deguchi , T . 1979 . Circadian rhythm of serotonin N-acetyltransferase activity in organ culture of chicken pineal gland . Science , 203 : 1245 – 1247 .
- Deguchi , T . 1981 . Rhodopsin-like photosensitivity of isolated chicken pineal gland . Nature , 290 : 706 – 707 .
- Eskin , A . 1979 . Identification and physiology of circadian pacemakers . Federation of American Societies for Experimental Biology , 38 : 2570 – 2572 .
- Gothilf , Y , Coon , SL , Toyama , R , Chitnis , A , Namboodiri , MA and Klein , DC . 1999 . Zebrafish serotonin N-acetyltransferase-2: marker for development of pineal photoreceptors and circadian clock function . Endocrinology , 140 : 4895 – 4903 .
- Hall , TA . 1999 . BioEdit: a user-friendly biological sequence alignment editor and analysis program for Windows 95/98/NT . Nucleic Acids Symposium Series , 41 : 95 – 98 .
- Helvik , JV , Drivenes , Ø , Naess , TH , Fjose , A and Seo , HC . 2001 . Molecular cloning and characterization of five opsin genes from the marine flatfish Atlantic halibut (Hippoglossus hippoglossus) . Visual Neuroscience , 18 : 767 – 780 .
- Hope , AJ , Partridge , JC and Hayes , PK . 1998 . Switch in rod opsin gene expression in the European eel, Anguilla anguilla (L.) . Proceeding of the Royal Society of London B , 265 : 869 – 874 .
- Iigo , M , Furukawa , K , Hattori , A , Hara , M , Ohtani-Kaneko , R , Suzuki , T , Tabata , M and Aida , K . 1995 . Effects of pinealectomy and constant light exposure on day-night changes of melatonin binding sites in the goldfish brain . Neuroscience Letters , 197 : 61 – 64 .
- Ikegami , T , Motohashi , E , Doi , H , Hattori , A and Ando , H . 2009 . Synchronized diurnal and circadian expressions of four subtypes of melatonin receptor genes in the diencephalon of a puffer fish with lunar-related spawning cycles . Neuroscience Letters , 462 : 58 – 63 .
- Im , LHJ , Isoldi , MC , Scarparo , AC , Visconti , MA and Castrucci , AM . 2007 . Rhythmic expression, light entrainment and alpha-MSH modulation of rhodopsin mRNA in a teleost pigment cell line . Comparative Biochemistry and Physiology Part A , 147 : 691 – 696 .
- Karaganis , SP , Bartell , VR , Shende , VR , Moore , AF and Cassone , VM . 2009 . Modulation of metabolic and clock gene mRNA rhythms by pineal and retinal circadian oscillators . General and Comparative Endocrinology , 161 : 179 – 192 .
- Khorana , HG , Reeves , PJ and Kim , JM . 2002 . Structure and mechanism in G protein-coupled receptors . American Pharmaceutical Review , 9 : 287 – 294 .
- Kim , JM , Kim , SW and Kim , SK . 2007 . Molecular cloning and characterization of the rod opsin gene in olive flounder Paralichthys olivaceus . Journal of Fisheries Science and Technology , 10 : 8 – 15 .
- King , DP and Takahashi , JS . 2000 . Molecular genetics of circadian rhythms in mammals . Annual Review Neuroscience , 23 : 713 – 742 .
- Kojima , D , Mano , H and Fukada , Y . 2000 . Vertebrate ancientlong opsin: a green-sensitive photoreceptive molecule present in zebrafish deep brain and retinal horizontal cells . The Journal of Neuroscience , 20 : 2845 – 2851 .
- Livak , KJ and Schmittgen , TD . 2001 . Analysis of relative gene expression data using realtime quantitative PCR and the 2−ΔΔC T method . Methods , 25 : 402 – 408 .
- Mano , H , Kojima , D and Fukada , Y . 1999 . Exo-rhodopsin: a novel rhodopsin expressed in the zebrafish pineal gland . Molecular Brain Research , 73 : 110 – 118 .
- Minamoto , T and Shimizu , I . 2003 . Molecular cloning and characterization of rhodopsin in a teleost (Plecoglossus altivelis, Osmeridae) . Comparative Biochemistry and Physiology Part B , 134 : 559 – 570 .
- Munz , FW and McFarland , WN . 1977 . “ Evolutionary adaptations of fishes to the photic environment ” . In The visual system in vertebrates , Edited by: Crescitell , F . 193 – 274 . New York : Springer .
- Nakayama , TA and Khorana , HG . 1991 . Mapping of the amino acids in membrane-embedded helices that interact with the retinal chromophore in bovine rhodopsin . The Journal of Biological Chemistry , 266 : 4269 – 4275 .
- O'Brien , J , Ripps , H and Al-Ubaidi , MR . 1997 . Molecular cloning of a rod opsin cDNA from the skate retina . Gene , 193 : 141 – 150 .
- Ovchinnikov , YA , Abdulaev , NG and Bogachuk , AS . 1988 . Two adjacent cysteine residues in the C-terminal cytoplasmic fragment of bovine rhodopsin are palmitylated . FEBS Letters , 230 : 1 – 5 .
- Park , YJ , Park , JG , Hiyakawa , N , Lee , YD , Kim , SJ and Takemura , A . 2007 . Diurnal and circadian regulation of a melatonin receptor, MT1, in the golden rabbitfish, Siganus guttatus . General and Comparative Endocrinology , 150 : 253 – 262 .
- Philp , AR , Bellingham , J , Garcia-Fernandez , JM and Forster , RG . 2000 . A novel rod like opsin isolated from the extra-retinal photoreceptors of teleost fish . FEBS Letters , 468 : 181 – 188 .
- Pierce , LX , Noche , RR , Ponomareva , O , Chang , C and Liang , JO . 2008 . Novel function for Period 3 and Exo-rhodopsin in rhythmic transcription and melatonin biosynthesis within the zebrafish pineal organ . Brain Research , 1223 : 11 – 24 .
- Sakmar , TP , Franke , RR and Khorana , HG . 1989 . Glutamic acid-113 serves as the retinylidene Schiff base counterion in bovine rhodopsin . Proceeding of the National Academy of Sciences of the United States of America , 86 : 8309 – 8313 .
- Samach , A and Coupland , G . 2000 . Time measurement and the control of flowering in plants . BioEssays , 22 : 38 – 47 .
- Schantz , M , Lucas , RJ and Foster , RG . 1999 . Circadian oscillation of photopigment transcript levels in the mouse retina . Molecular Brain Research , 72 : 108 – 114 .
- Simonneaux , V and Ribelayga , C . 2003 . Generation of the melatonin endocrine message in mammals: a review of the complex regulation of melatonin synthesis by norepinephrine, neuropeptides and other pineal transmitters . Pharmacological Reviews , 55 : 325 – 395 .
- Skene , DJ , Deason , S and Arendt , J . 1996 . Use of melatonin in circadian rhythm disorders and following phase shifts . Acta Neurobiologiae Experimentalis (Warsz) , 56 : 359 – 362 .
- Soni , BG and Foster , RG . 1997 . A novel and ancient vertebrate opsin . FEBS Letters , 406 : 279 – 283 .
- Stanewsky , R , Kaneko , M , Emery , P , Beretta , B , Wager-Smith , K , Kay , SA , Rosbash , M and Hall , JC . 1998 . The cryb mutation identifies cryptochrome as a circadian photoreceptor in Drosophila . Cell , 95 : 681 – 692 .
- Takemura , A , Susilo , ES , Rahman , MD and Morita , M . 2004 . Perception and possible utilization of moonlight intensity for reproductive activities in a lunar-synchronized spawner, the golden rabbitfish . Journal of Experimental Zoology A , 301 : 844 – 851 .
- Takemura , A , Ueda , S , Hiyakawa , N and Nikaido , Y . 2006 . A direct influence of moonlight intensity on changes in melatonin production by cultured pineal glands of the golden rabbitfish, Siganus guttatus . Journal of Pineal Research , 40 : 236 – 241 .
- Terakita , A . 2005 . The opsins . Genome Biology , 6 : 213
- Vera , LM , Davie , A , Taylor , JF and Migaud , H . 2010 . Differential light intensity & spectral sensitivities of Atlantic salmon, European sea bass and Atlantic cod pineal glands ex vivo . General and Comparative Endocrinology , 165 : 25 – 33 .
- Wang , JK , McDowell , JH and Hargrave , PA . 1980 . Site of attachment of 11-cis-retinal in bovine rhodopsin . Molecular Biology and Evolution , 12 : 53 – 61 .
- Yokoyama , S . 2000 . Molecular evolution of vertebrate visual pigments . Progress in Retinal and Eye Research , 19 : 385 – 419 .
- Yokoyama , S and Radlwimmer , FB . 1998 . The ‘five-sites’ rule and the evolution of red and green color vision in mammals . Molecular Biology and Evolution , 15 : 560 – 567 .
- Yokoyama , S and Zhang , H . 1997 . Cloning and characterization of the pineal gland-specific opsin gene of marine lamprey (Petromyzon marinus) . Gene , 202 : 89 – 93 .
- Yokoyama , S , Zhang , H , Radlwimmer , FB and Blow , NS . 1999 . Adaptive evolution of color vision of the Comoran coelacanth (Latimeria chalumnae) . Proceeding of the National Academy of Sciences of the United States of America , 96 : 6279 – 6284 .
- Zatz , M and Mullen , DA . 1988 . Two mechanisms of photoendocrine transduction in cultured chick pineal cells: pertussis toxin blocks the acute but not the phase-shifting effects of light on the melatonin rhythm . Brain Research , 453 : 63 – 71 .
- Zhang , T , Tan , YH , Fu , J , Lui , D , Ning , Y , Jirik , FR , Brenner , S and Venkatesh , B . 2003 . The regulation of retina specific expression of rhodopsin gene in vertebrates . Gene , 313 : 189 – 200 .
- Ziv , L , Levkovitz , S , Toyama , R , Falcon , J and Gothilf , Y . 2005 . Functional development of the zebrafish pineal gland: light-induced expression of period2 is required for onset of the circadian clock . Journal of Neuroendocrinology , 17 : 314 – 320 .