Abstract
Until now, the isolation and characterisation of bovine bone marrow-derived mesenchymal stem cells (bBM-MSCs) have not been established, which prompted us to optimise the differentiation protocol for bBM-MSCs. In this study, bBM-MSCs were freshly isolated from three six-month-old cattle and used for pluripotent or phenotypic characterisation by reverse transcription polymerase chain reaction (RT-PCR) and flow cytometer. Initially, the cells were adherent to plastic surfaces and exhibited spindle-like morphology. The cells expressed pluripotent as well as typical MSC markers. In addition, bBM-MSCs were differentiated into chondrocytes and osteocytes, but less efficiently into adipocytes. Accordingly, we conducted this study to establish the optimal adipogenic differentiation protocol under specific culture conditions. For this purpose, we formulated the basal differentiation medium using low-glucose Dulbecco's modified eagle medium (DMEM) supplemented with 10% (v/v) foetal bovine serum (FBS), 1% (v/v) penicillin/streptomycin (P/S), 1 µM dexamethasone, 0.5 mM indomethacin and 0.5 mM 3-isobutyl-l-methylxanthine, and added with three levels of insulin at 10, 15 and 20 µg/mL as insulin is the key adipogenesis inducer. The level of differentiation was evaluated by Oil Red O staining and by analysing the expression of adipocyte lineage-specific genes by a quantitative real time RT-PCR. In this study, we found that the bBM-MSCs were effectively differentiated into adipocytes as manifested by the presence of Oil Red O-stained lipid droplets. The mRNA levels of adipocyte-specific genes in the differentiated cells were highly expressed as compared with the non-differentiated bBM-MSCs. In conclusion, we successfully isolated and characterised bBM-MSCs with multipotent and differentiation potential. Additionally, enhanced in vitro adipogenic differentiation protocol for bBM-MSCs was established.
1. Introduction
Mesenchymal stem cells (MSCs) are multipotent stromal cells which can differentiate into a variety of cell types with considerable therapeutic and regenerative potential in human and veterinary science. Although MSCs have been isolated from bone marrow (BM), brain, adipose tissue and umbilical cord blood, the former was the most well-studied source of MSCs. It has been reported that BM-derived MSCs (BM-MSCs) are known to differentiate into chondrocytes, osteocytes and adipocytes, and other types of cells (Jiang et al. Citation2002a, Citation2002b). Although BM-MSCs have been isolated from various species including human and experimental animals, such as murine (Jiang et al. Citation2002b; Grove et al. Citation2004), canine (Csaki et al. Citation2007), feline (Webb et al. Citation2012), porcine (Pankajakshan et al. Citation2012), caprine (Pratheesh et al. Citation2012) and equine (Ranera et al. Citation2012), there is limited information on bovine MSCs (Bosnakovski et al. Citation2005). Thus, the present study was conducted to isolate and characterise the multipotent bovine BM-MSCs (bBM-MSCs). While the cells isolated in this study expressed the pluripotent and MSC markers at the molecular levels, adipogenic differentiation with commercial induction medium was not successful, which prompted us to further establish enhanced in vitro adipogenic differentiation protocol for bBM-MSCs. In general, a combination of dexamethasone, 3-isobutyl-1-methylxanthine (IBMX) and insulin is commonly employed to induce adipogenesis in MSCs. As insulin plays an important role in adipogenesis, we assessed three levels of insulin within its physiological dose in the differentiation medium (Gagnon & Sorisky Citation1998) for bBM-MSCs.
2. Materials and methods
2.1. Isolation and culture of bBM-MSCs
BM was aspirated from freshly slaughtered three six-month-old male Holstein breed cattle into syringes containing heparin (1000 units) and kept on ice until later use. bBM-MSCs were isolated as described elsewhere (Worster et al. Citation2001; Bosnakovski et al. Citation2005; Mauck et al. Citation2006). In brief, one volume of BM sample was mixed with two volumes of Dulbecco's modified eagle medium-low glucose (DMEM-LG, Gibco), and centrifuged for 10 min at 300 × g to obtain a cell pellet. After the removal of supernatant, the cells were resuspended in 25 mL of DMEM-LG containing 10% foetal bovine serum (FBS, Gibco) and 1% penicillin/streptomycin (P/S, Gibco), and 1 × 106 cells were then plated and cultured in T-150 culture flasks at 37°C in a humidified atmosphere of 5% CO2. The non-adherent cells were removed by changing the culture medium (DMEM + GlutaMax™-I, Gibco), supplemented 10% FBS and 1% P/S, every three days after seeding. The cells were detached using 0.25% Trypsin-ethylenediaminetetraacetic acid (EDTA) solution (Gibco) and replated into culture flasks at a 1:4 split ratio. All experimental protocols were approved by the Animal Welfare Committees of the Quarantine and Inspection Agency.
2.2. Fluorescence-activated cell sorting analysis
Fluorescence-activated cell sorting (FACS) analysis was performed to investigate the expression of typical MSC marker, CD44 or haematopoietic marker, CD45, in bBM-MSCs at third passage. bBM-MSCs were dispersed into a single cell suspension in ice cold Dulbecco's phosphate-buffered saline (DPBS, Gibco) containing 0.5% bovine serum albumin (BSA, Gibco) at a concentration of 5.0 × 105 cells/100 µl. The cells were then incubated for 20 min on ice with phycoerythrin (PE)-conjugated anti-bovine CD44 (AbD seroTec) or fluorescein isothiocyanate (FITC)-conjugated anti-bovine CD45 (AbD seroTec). Analyses were performed by flow cytometry (BD Biosciences) using the Cell Quest software (BD Biosciences).
2.3. Chondrogenic differentiation
The cells were plated in 4-well plates at 5.0 × 105 cells/drops density. After 4 hours of incubation, the culture medium was changed to chondrogenic differentiation medium (Lonza) and changed every three days. Three weeks later, the cells were stained with Alcian blue to see the presence of acid mucosubstances.
2.4. Osteogenic differentiation
The cells were plated in 4-well plates at 4.0 × 104 cells/wells density. After cell reached 80–90% confluence, the culture medium was changed to osteogenic differentiation medium consisting of DMEM-LG supplemented with 10% (v/v) FBS, 1% (v/v) P/S, 100 nM dexamethasone (Sigma), 10 mM β-glycerol phosphate (Sigma) and 0.2 mM ascorbic acid (Sigma) solution. Medium was changed every three days. Three weeks later, osteogenesis was demonstrated by the accumulation of mineralised calcium phosphate assessed by Alizarin Red stain.
2.5. Adipogenic differentiation
For adipogenesis of bBM-MSCs, we used either commercially available adipogenic induction medium (Lonza) or manually formulated induction medium. Initially, bBM-MSCs were not successfully differentiated into adipocytes when the commercial induction medium was used. Thus, we added 10 µg/mL insulin into the commercial induction medium to induce the adipogenesis of bBM-MSCs. Based on the latter observation, we formulated the optimal induction medium consisting of DMEM-LG supplemented with 10% (v/v) FBS, 1% (v/v) P/S, 1 µM dexamethasone, 0.5 mM indomethacin (Sigma) and 0.5 mM IBMX (Sigma). All basal components used in this study were commercially available. However, indicated concentrations of the components (1 µM dexamethasone, 0.5 mM indomethacin and 0.5 mM IBMX) were established in our laboratory. As insulin plays an important role in adipogenesis, we used three levels of insulin within its physiological range in the differentiation medium at 10, 15 and 20 µg/mL. At three weeks induction, neutral lipid droplets in the differentiated cells were examined by staining with Oil Red O. As a control, bBM-MSCs were incubated with the culture medium only. The expression of the adipogenic-specific genes, CCAAT/enhancer-binding protein alpha (C/EBPα), peroxisome proliferator-activated receptor gamma (PPARγ) and lipoprotein lipase (LPL), was analysed in the differentiated or undifferentiated cells.
2.6. Reverse transcription polymerase chain reaction
Total RNAs were prepared from bBM-MSCs or the differentiated cells using a Trizol Kit (Molecular Research Center, Inc.) according to the manufacturer's instructions. Oligonucleotide primers for stemness and cell surface markers in the bBM-MSCs and glyceraldehyde 3-phosphate dehydrogenase (GAPDH) internal control were presented in . PCR was conducted at 94°C for 5 min; followed by 35 cycles of 94°C for 45 s at 55–60°C for 45 s, and 72°C for 45 s; 72°C for 5 min. The amplified products were visualised by electrophoresis using a 2% agarose gel.
Table 1. Oligonucleotide primers designed for pluripotent and mesenchymal stem cell CD markers and tissue-specific differentiation markers.
The expression levels of transcripts encoding the tissue-specific markers (C/EBPα, PPARγ and LPL) and GAPDH as internal control were conducted using quantitative real-time reverse transcription polymerase chain reaction (RT-PCR). In brief, cDNA synthesis was conducted using oligonucleotide primers () according to the manufacturer's protocols (Promega). Real-time RT-PCR using Light Cycler 480® system (Roche) was performed with 2 µg of each cDNA sample in triplicate using the Light Cycler 480® SYBR green master (Roche). Relative mRNA expression levels were calibrated to GAPDH and expression levels of differentiated cells are calculated relative to their respective bBM-MSCs.
2.7. Statistical analysis
Data on relative mRNA expression levels (i.e., C/EBPα, PPARγ and LPL) in the differentiated vs. undifferentiated cells are expressed as means ± standard deviation (SD). All statistical analyses were performed using one-way analysis of variance. A P values < 0.05 (*) were considered statistically significant.
3. Results
3.1. Isolation of bBM-MSCs
The non-adherent cells were removed during the first medium change at 24 h. As expected, bBM-MSCs adhered to plastic surface and had typical spindle-like appearance ().
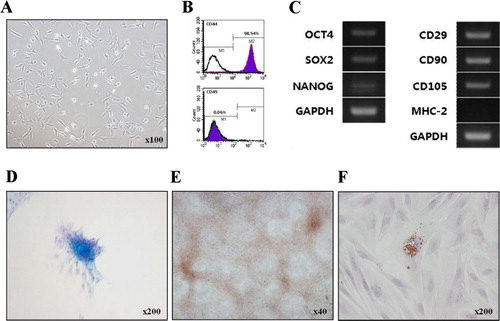
3.2. Characterisation of bBM-MSCs
FACS analysis indicated that bBM-MSCs were positive for the typical MSC marker, i.e., CD44 (98.54%) and negative for CD45 (0.06%; ). RT-PCR analysis showed that bBM-MSCs expressed MSCs-surface markers, i.e., CD29, CD90 and CD105, but did not express the immune cell marker major histocompatibility complex class II (MHC-2) (), indicating that these cells originated from mesenchymal lineage cells. In addition, we found that the bBM-MSCs expressed pluripotent markers such as OCT4, SOX2 and NANOG ().
3.3. In vitro differentiation potentials of bBM-MSCs
Using the commercially available induction medium and conditions used in this study, bBM-MSCs were able to differentiate into chondrocytes () and osteocytes () but not into adipocytes (data not shown). However, the addition of 10 µg/mL insulin into the commercially available induction medium induced adipogenesis of bBM-MSCs although Oil Red O-stained lipid droplets were relatively low (). Thus, we attempted to optimise the adipogenic induction media.
3.4. In vitro adipogenic differentiation of bBM-MSCs
We formulated the optimum culture conditions for the adipogenic induction in bBM-MSCs. The newly formulated adipogenic induction medium contained key components such as dexamethasone, IBMX and indomethacin. In addition, the induction medium was added with three levels of insulin at concentrations of 10, 15 and 20 µg/mL as insulin is the key inducer of adipogenesis. As shown in , the cells in insulin-added induction media were differentiated and contained a high number of very small lipid vacuoles that stained positively using Oil red solution. RT-PCR and qRT-PCR assays confirmed that adipocyte-specific genes, i.e., C/EBPα, PPARγ and LPL, were all expressed in the differentiated, but not in undifferentiated cells (). No adipocytes were formed when the bBM-MSCs were incubated with the growth medium only.
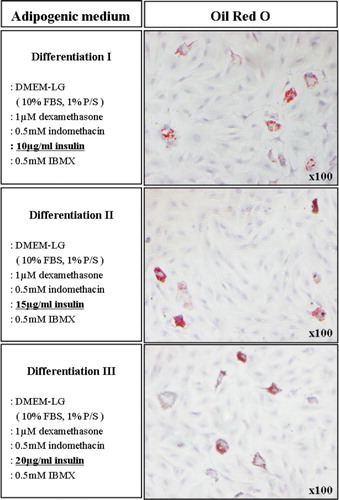
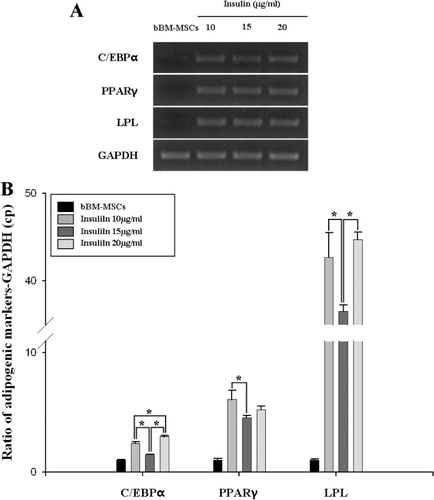
4. Discussion
The adherent cells were isolated from bBM and exhibited typical fibroblast-like morphology. FACS analysis found that the isolated bBM-MSCs were positive (98.54%) for MSC-specific marker CD44, but negative (0.06%) for haematopoietic-specific marker CD45. These cells have been found to be pluripotent (OCT4, SOX2 and NANOG) and express various CD markers (CD29, CD90 and CD105) as confirmed by RT-PCR. As expected, the immune cell marker MHC-2 was not expressed in bBM-MSCs. In mammals, OCT4, SOX2 and NANOG are three important binding transcription factors present in the undifferentiated embryonic and adult stem cells with a high proliferative capacity and known to regulate self-renewal and pluripotency (He et al. Citation2006; Zhang et al. Citation2010; Potdar & D'Souza Citation2011). In addition, bBM-MSCs could be induced to differentiate into chondrocytes, osteocyte and adipocytes. The tissue-specific differentiated cells showed positive in the light microscope by each immunogold staining. Collectively, these data clearly indicate that bBM-MSCs isolated in this study show typical MSC-like characteristics (Ducy Citation2000; Jiang et al. Citation2002a; Dominici et al. Citation2006; Pankajakshan et al. Citation2012).
Cell differentiation is mainly a regulated process that depends on various extracellular and intracellular factors of its modulation. It is well known that insulin is the key inducer of adipocyte differentiation. Insulin has a strong anti-lipolytic action, stimulates lipogenesis and triggers and promotes adipocyte differentiation in vitro (Klemm et al. Citation2001). In this study, bBM-MSCs in commercially available adipogenic induction medium did not differentiate into adipocytes at all. Thus, we attempted to add 10 µg/mL of insulin into the induction medium to facilitate the differentiation process. By doing this, we were able to see Oil Red O-stained lipid drops in the differentiated cells. Based on our observation, we decided to establish enhanced in vitro adipogenic differentiation protocol for bBM-MSCs. We formulated the in-house induction medium consisting of key components, such as dexamethasone, IBMX and indomethacin, in addition to the various physiological concentrations of insulin at 10–20 µg/mL. It was found that all bBM-MSCs in the induction medium were able to differentiate into adipocytes, suggesting that culture condition and medium composition for adipogenic differentiation are optimised.
Adipocyte differentiation is a complex process that involves expression of several adipocyte-specific genes, including C/EBPα, PPARγ, and LPL, which lead to morphological changes and lipid accumulation within the cells (Klemm et al. Citation2001; Zhang et al. Citation2009). Our results showed that C/EBPα, PPARγ and LPL were detected in the differentiated cells, but not in the intact bBM-MSCs as manifested by RT-PCR. The mRNA levels of C/EBPα, PPARγ and LPL were increased in bBM-MSCs and treated with insulin, but the expression levels were not dose-dependent. Instead, our result indicates that insulin treated with 10 µg/mL in the induction medium seems optimal based on the adipogenic differentiation and the expression levels of adipocyte-specific genes in the differentiated cells.
In conclusion, we successfully isolated and characterised bBM-MSCs with multipotent potential. Furthermore, the optimal culture condition of adipogenic differentiation in bBM-MSCs was established with insulin level at 10 µg/mL in adipogenic induction medium containing dexamethasone, IBMX and indomethacin.
Funding
This work was supported by a grant [P-AD20-2010-12-01] from the Animal and Plant Quarantine Agency, Ministry of Agriculture, Food and Rural Affairs, the Republic of Korea.
Additional information
Funding
References
- Bosnakovski D, Mizuno M, Kim G, Takagi S, Okumura M, Fujinaga T. 2005. Isolation and multilineage differentiation of bovine bone marrow mesenchymal stem cells. Cell Tissue Res. 319:243–253.
- Csaki C, Matis U, Mobasheri A, Ye H, Shakibaei M. 2007. Chondrogenesis, osteogenesis and adipogenesis of canine mesenchymal stem cells: a biochemical, morphological and ultrastructural study. Histochem Cell Biol. 128:507–520.
- Dominici M, Le Blanc K, Mueller I, Slaper-Cortenbach I, Marini F, Krause D, Deans R, Keating A, Prockop D, Horwitz E. 2006. Minimal criteria for defining multipotent mesenchymal stromal cells. The International Society for Cellular Therapy position statement. Cytotherapy. 8:315–317.
- Ducy P. 2000. Cbfa1: a molecular switch in osteoblast biology. Dev Dynam. 219:461–471.
- Gagnon A, Sorisky A. 1998. The effect of glucose concentration on insulin-induced 3T3-L1 adipose cell differentiation. Obes Res. 6:157–163.
- Grove JE, Bruscia E, Krause DS. 2004. Plasticity of bone marrow-derived stem cells. Stem Cells. 22:487–500.
- He S, Pant D, Schiffmacher A, Bischoff S, Melican D, Gavin W, Keefer C. 2006. Developmental expression of pluripotency determining factors in caprine embryos: novel pattern of NANOG protein localization in the nucleolus. Mol Reprod Dev. 73:1512–1522.
- Jiang Y, Jahagirdar BN, Reinhardt RL, Schwartz RE, Keene CD, Ortiz-Gonzalez XR, Reyes M, Lenvik T, Lund T, Blackstad M, et al. 2002a. Pluripotency of mesenchymal stem cells derived from adult marrow. Nature. 418:41–49.
- Jiang Y, Vaessen B, Lenvik T, Blackstad M, Reyes M, Verfaillie CM. 2002b. Multipotent progenitor cells can be isolated from postnatal murine bone marrow, muscle, and brain. Exp Hematol. 30:896–904.
- Klemm DJ, Leitner JW, Watson P, Nesterova A, Reusch JE, Goalstone ML, Draznin B. 2001. Insulin-induced adipocyte differentiation. Activation of CREB rescues adipogenesis from the arrest caused by inhibition of prenylation. J Biol Chem. 276:28430–28445.
- Mauck RL, Yuan X, Tuan RS. 2006. Chondrogenic differentiation and functional maturation of bovine mesenchymal stem cells in long-term agarose culture. Osteoarthr Cartilage. 14:179–189.
- Pankajakshan D, Kansal V, Agrawal DK. 2012. In vitro differentiation of bone marrow derived porcine mesenchymal stem cells to endothelial cells. J Tissue Eng Regener Med. doi:10.1002/term.1483
- Potdar PD, D'Souza SB. 2011. Isolation of Oct4+, Nanog+ and SOX2- mesenchymal cells from peripheral blood of a diabetes mellitus patient. Hum Cell. 24:51–55.
- Pratheesh MD, Gade NE, Katiyar AN, Dubey PK, Sharma B, Saikumar G, Amarpal,Sharma GT. 2012. Isolation, culture and characterization of caprine mesenchymal stem cells derived from amniotic fluid. Res Vet Sci. 94:313–319.
- Ranera B, Remacha AR, Alvarez-Arguedas S, Romero A, Vazquez FJ, Zaragoza P, Martin-Burriel I, Rodellar C. 2012. Effect of hypoxia on equine mesenchymal stem cells derived from bone marrow and adipose tissue. BMC Vet Res. 8:142.
- Webb TL, Quimby JM, Dow SW. 2012. In vitro comparison of feline bone marrow-derived and adipose tissue-derived mesenchymal stem cells. J Feline Med Surg. 14:165–178.
- Worster AA, Brower-Toland BD, Fortier LA, Bent SJ, Williams J, Nixon AJ. 2001. Chondrocytic differentiation of mesenchymal stem cells sequentially exposed to transforming growth factor-beta1 in monolayer and insulin-like growth factor-I in a three-dimensional matrix. J Orthopaedic Res. 19:738–749.
- Zhang D, Efendic S, Brismar K, Gu HF. 2010. Effects of MCF2L2, ADIPOQ and SOX2 genetic polymorphisms on the development of nephropathy in type 1 Diabetes Mellitus. BMC Med Genet. 11:116.
- Zhang HH, Huang J, Duvel K, Boback B, Wu S, Squillace RM, Wu CL, Manning BD. 2009. Insulin stimulates adipogenesis through the Akt-TSC2-mTORC1 pathway. PLoS One. 4:e6189.