Abstract
The purpose of this study was to investigate the effects of phytase on iron requirement of broiler breeder hens at the late stage of production cycle. Ninety-six Cobb (500) broiler breeder hens were weighed and individually placed in galvanized wire cages at 59 weeks of age. In order to depletion of hens iron reserved, after placement, hens were offered a semi-purified iron-deficient (37 mg iron/kg) diet for three weeks. At 62 weeks of age, hens were randomly allocated to eight dietary treatments in a factorial arrangement with four replicates of three hens in each. Factors included two levels of phytase (0 and 600 U/kg) and four levels of iron (37, 52, 67 and 82 mg/kg). Hens were fed the experimental diets from 62 to 68 weeks of age. Iron in the diet had significant effects on egg weight (P < 0.05). Increasing dietary iron content significantly increased the concentration of iron in the bone marrow, liver, spleen (P < 0.05), egg yolk and blood serum (P < 0.01). Added phytase enhanced the iron concentration of the egg yolk and serum (P < 0.01). It seems that phytase can release iron from inositol in old broiler breeder hens and increased chicken iron reserve. Two slope, broken-line analysis of hen house egg production regressed on the dietary added iron indicated that 65 mg/kg supplemental iron without phytase and 58 mg/kg supplemental iron with phytase were required for the optimal egg production of broiler hens.
1. Introduction
Iron is an essential mineral for animal growth and development because it takes part in an array of biochemical processes vital in maintaining normal cellular function. Biochemical processes involved include electron transport (cytochromes and iron–sulphur proteins), handing of molecular oxygen (peroxidase and catalase), oxygen transport and storage (haemoglobin and myoglobin) porphyrin metabolism, collagen synthesis, lymphocyte and granulocyte function and neurotransmitter anabolism and catabolism (McDowell Citation2003). Also, iron is especially essential during development, as it is required for proper myelination and also is a cofactor for enzymes in neurotransmitter synthesis (Pollitt & Leibel Citation1976; Reichmann et al. Citation1995). Few studies were performed on the iron requirements of poultry (Chio et al. Citation1976; Morck & Austic Citation1981; Aoyagi & Baker Citation1995). But none of the researches have been conducted on effect of phytase on iron requirement of poultry. On the other hands, in spite of the broiler breeder hens' importance in meat production cycle, no investigation of the iron requirement of them has been reported. Only one study has been done on the effect of iron on broiler breeder hens that investigated the effect of iron on performance and iron content of broiler breeder hens' egg (Bess et al. Citation2012). They suggested that egg production in hens fed no supplemented iron diets showed a significant reduction in comparison with hens fed supplemented iron diets. Cao et al. (Citation1996) stated that the kidney, liver and bone marrow iron concentrations increased linearly by increasing dietary iron. Ramadan et al. (Citation2010) investigated 0, 100 and 200 mg/kg iron in laying hens and suggested that egg mass for layers fed by supplemental iron either at the level of 100 mg or at the level of 200 mg/kg diet in comparison with diet without iron was improved by 15% and 4.9%, respectively. Vahl and Van ‘T Klooster (Citation1987) recommended 100 mg/kg of iron for broiler. There are many factors that can affect iron absorption; proteins, calcium, polyphenols and Phytic acid (inositol hexaphosphate) can all reduce iron bioavailability (Hurrell Citation1997). Phytate can account for up to 5% of the weight in cereal grains and legumes (Maga Citation1982). It accounts for 0.62–1.35% of dry weight in wheat, 0.84–1.01% of dry weight in oats and 0.97–1.08% of dry weight in barley (Maga Citation1982). More than 80% of the total phosphorous in corn is in the form of phytate phosphorous (De Boland et al. Citation1975). There appears to be a dose–response relationship between the level of phytate in a food and iron absorption (Hallberg & Hulthén Citation2000). It is poorly digestible for humans and monogastric animals due to a lack of effective endogenous phytase (Bitar & Reinhold Citation1972). Phytate acts as an anti-nutritional factor, exerting its effects via a reduction in the solubility, and so availability of phosphorus (P) and to a lesser extent, calcium, zinc and iron (Hallberg et al. Citation1987, Hurrell et al. Citation2003). Several studies have shown that supplementing animal diets with phytase improves mineral bioavailability (Simons et al. Citation1990; Lei et al. Citation1993; Stahl et al. Citation1999; Augspurger & Baker Citation2004; Shelton & Southern Citation2006). Compared to broiler chicks, phytase inclusion in diets for laying hens has been the subject of less research. Exogenous phytase enzymes are known to release not only phosphorus bound by the phytase molecule but also other nutrients including calcium and amino acids (Cosgrove Citation1966; Selle & Ravindran Citation2007). Recently, it has been reported that phytase enzymes may also affect iron metabolism. Liebert et al. (Citation2005) contend that the benefits of phytase supplementation of layer diets are ‘still under discussion’. These studies were conducted to determine the effect of iron deficiency in the broiler breeder hens and to determine the amount of dietary iron required with phytase and without phytase for maximal performance.
2. Materials and methods
2.1. Animals and sampling
Ninety-six Cobb 500 broiler breeder hens were weighed and individually placed in galvanized wire cages (45 cm height × 38 cm depth × 30 cm width) at 59 weeks of age. Soft plastic wires were placed on the cage floors to inhibit bird's foot damages. In order to depletion of hens iron reserved, until 62 weeks of age, hens were offered a semi-purified iron deficient (37 mg iron/kg) diet that contained 13.7% of crude protein and 2680 kcal/kg of AMEn. The composition of the basal diets is shown in . After the depletion period, hens were randomly allocated to eight dietary treatments in a factorial arrangement of two levels of phytase (0 and 600 U/kg) and four levels of iron (37, 52, 67 and 82 mg/kg) with four replicates of three hens in each. Dietary treatments were made with the addition of FeSO4.4H2O to the basal diet. The composition of the experimental diets is shown in . Hens received a constant schedule of 16 h light (beginning at 6 am). The consumed water contained approximately 41.68 µg iron/l that was measured by polarography (Model VA 797 Metrohm). In this experiment, plastic trough drinker and individual feeder were used. Birds did not have any access to the feed of each other. Egg production and egg weight were recorded daily, and the egg mass was calculated at the end of experiment (egg weight × egg production). To evaluate blood serum iron concentration, first bleeding was carried out before initiation and next was done at the end of experiment. Blood samples were collected in heparin-coated tubes from the brachial vein. Blood samples were immediately centrifuged at 3500× g for 14 min to collect blood serum. Serum samples were stored at –20°C pending for iron concentration assays. Blood serum iron was determined by calorimetric method using an automated spectrophotometric analyzer (enzyme-linked immunosorbent assay plate reader model no. 259293) instrument. Egg component, Haugh units, shell-quality test and shell-breaking strengths measurements were done at the end of experiment. Shell-breaking strengths and shell thickness were measured by using an egg shell force gauge (model no. 55R1123, Instron Corp., Canton, MA) and Karl Deutsch D-56 (wuppertal echometer 1061), respectively. At 68 weeks of age, two hens per replicate were slaughtered by a neck cutter, and carcass characteristics were measured. The liver, heart and spleen were extracted from the carcass then weighed and frozen immediately for further analysis. The right tibia was pooled and then frozen for analysis of bone marrow iron content. The iron content of liver, heart, spleen, bone marrow and egg yolk was determined by atomic absorption spectrophotometry instrument (Shelton & Southern Citation2006).
Table 1. Composition of basal diets.
Table 2. Composition of experimental diets.Footnotea
2.2. Statistical analyses
The experimental design was a completely randomized design using individual broiler breeder hens as experimental units. The obtained results from experiments were analysed by general linear model (GLM) procedure of SAS Institute (Citation2002). Significant differences among treatment means were determined using Duncan's multiple range tests. In this experiment, the broken-line (Robbins et al. Citation2006), logistic and quadratic models (Pesti et al. Citation2009) were used to determine iron requirement.
3. Results
The effects of phytase and iron on performance of hens are shown in . Iron in the diet had significant effects on egg weight (P < 0.05). No significant effect of dietary added phytase of phytase and iron were observed on egg production, egg mass and body weight. Also, no significant interactions were observed between supplemented iron and phytase for egg production, egg weight, egg mass and body weight. The effects of phytase and iron levels on carcass characteristics of hens are shown in . Analysis showed that there was no significant effect of iron on the liver, heart and spleen weight. Significant differences were observed in heart weight (P < 0.01) and spleen weight (P < 0.05) of phytase-supplemented hens, but there was no significant effect on the liver weight. Lack of significant interactions were observed between iron levels and phytase supplementation for heart, spleen and liver weight. The effects of iron and phytase on egg quality of broiler breeder hens are presented in . Haugh unit, albumen height, shell thickness, egg shell-breaking strength, shell and yolk percentage were not significantly affected by dietary of iron. Also the phytase supplementation showed no significant differences on Haugh unit, albumen height, egg shell-breaking strength, shell and yolk percentage. Significant differences were observed in shell thickness (P < 0.05) and egg shell-breaking strength (P < 0.01) of phytase supplementation. No significant interactions were observed between iron and phytase supplementation for Haugh unit, albumen height, egg shell-breaking strength and shell and yolk fractional weight. But interaction between dietary added iron and phytase on shell thickness was significant (P < 0.05; ). The effects of iron and phytase on concentration of iron in tissue of broiler breeder hens are presented in . Based on the results by increasing dietary iron levels, the concentration of iron in the bone marrow, liver, spleen (P < 0.05), egg yolk and blood serum increased (P < 0.01). Dietary iron linearly increased iron concentrations in spleen, egg yolk, liver and bone marrow. The hens received the highest amount of iron and stored more iron in their organs. Hens fed with phytase supplementation showed high iron concentration in the egg yolk and blood serum (P < 0.01). According to result of this study, the phytase levels showed no significant differences on iron concentration in the bone marrow, spleen and liver. No significant interaction between iron levels and phytase supplementation for concentration of iron in the bone marrow, liver, spleen and blood serum of hens was observed. But interaction between dietary added iron and phytase on egg yolk iron content was significant (P < 0.05). Highest and lowest amount of egg yolk iron storage was observed at 37 mg/kg dietary iron, 0 phytase and 82 mg/kg dietary iron level and 600 unit supplemented phytase, respectively (). summarized the statistics, estimated requirement values and appropriate models used for estimation. According to the results of the broken-line models, estimated iron requirement without phytase for hen house egg production, egg production percentage, egg mass, iron content of the liver, blood serum, spleen and egg yolk was 65, 65, 64, 54, 68, 63 and 68 mg/kg, respectively (–). Based on the quadratic model, estimated iron requirement without phytase for egg weight was 66 mg/kg (). The logistic model that was fitted to iron content of spleen proposed that iron requirement without phytase was equal to 53 mg/kg (). Two slope, broken-line analysis of hen house egg production regressed on the supplemental iron indicated that 58 mg/kg supplemental iron with phytase was required for the optimal egg production of broiler breeder ().
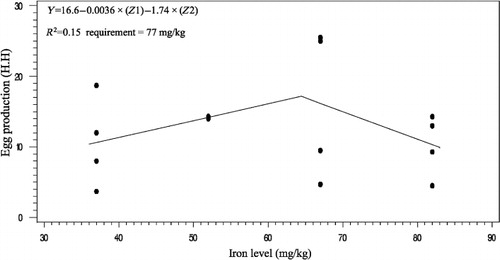
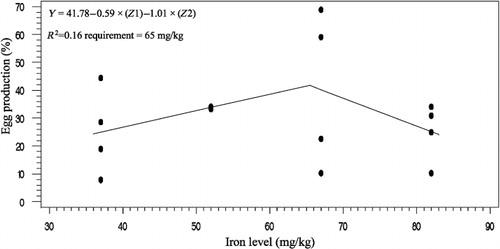
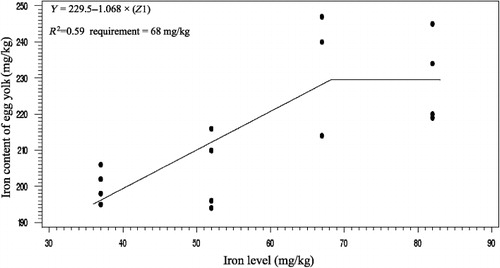
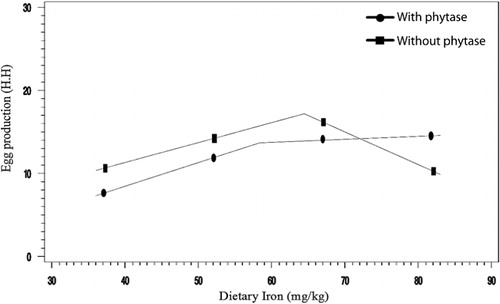
Table 7. The estimated requirement of broiler breeder hens.
4. Discussion
The results of this study showed that, increasing the amount of iron in the diet increases egg weight. The highest egg weight was observed in hens fed supplemented diet with 67 mg iron/kg. Morck and Austic (Citation1981) and Park et al. (Citation2004) reported that the iron can influence egg weight. Ramadan et al. (Citation2010) agreed with results of this experiment and stated that egg weight increases by dietary iron in the layer hens but had no effects on the egg quality. Ovotransferrin is one of the iron-containing compounds in the egg white albumin which forms 12% of the amount of the egg protein. Increased egg weight in the present study may be due to increasing the amount of ovotransferrin in the albumen of hens that received 67 mg iron/kg of iron. These results are in disagreement with the published data by Bess et al. (Citation2012). The choice of a model to evaluate data should depend on objectives of the experiment (Baker Citation1986). Pesti et al. (Citation2009) suggested that the broken-line models more closely represent theoretical ideas of the nature of nutritional responses than polynomial models. The ranges of optimal iron requirement of broiler breeder hens at late stage of production cycle are 54–72 g/kg of diet (Figure 1–4). The model: Y = 17.18–0.24 × (65–x)–0.393 × (x–65), where (65–x) is defined as zero at values of x > 65, and (x–65) is defined as zero when x < 65, fitted to the data from groups without supplementation of phytase. The model indicated that the breakpoint occurred at 65 mg/kg diet (). In White Leghorn hens, the iron requirement was 35–45 ppm for maintenance of hematocrit and 55 ppm for maximum of hatchability (Morck & Austic Citation1981). These researchers determined the iron requirement of White Leghorn hens by diet that contained levels of 15–65 mg iron/kg. Davis et al. (Citation1962) and McNaughton and Day (Citation1979) suggested that the iron requirements of broiler were 79–80 mg iron/kg. Aoyagi and Baker (Citation1995) showed that the weight gain, hematocrit, haemoglobin and iron content in blood serum increases when graded levels 0, 5, 10, 20, 30, 40 and 50 mg/kg of iron from analytical grade FeSO4.7H2O were added to iron-deficient basal diet containing 46 mg iron/kg. Also suggested that the maximum response in hematocrit, haemoglobin, weight gain, feed intake and iron content in blood serum occurred between 30 and 40 mg/kg supplemental iron. Cobb 500 and Ross 308 breeder management guide recommended 50–55 mg/kg of iron of diet for broiler breeder hens. The model: Y = 13.68–0.285 × (58–x) + 0.036 × (x–58), where (58–x) is defined as zero at values of x > 58 and (x–58) is defined as zero when x < 58, fitted to the data from groups with supplementation of phytase. The model indicated that the breakpoint occurred at 58 mg/kg diet (). Based on these data, the iron equivalency of 600 U/kg phytase is 7 mg/kg. Significant differences were observed in shell thickness and egg shell-breaking strength of phytase supplementation. Decrease in shell-breaking strengths and shell thickness was observed due to addition of phytase to the diet. Also it can be related to greater egg weight in the hens' received phytase which is in disagreement with reports by Lim et al. (Citation2003) and Casartelli et al. (Citation2005) who reported that phytase does not affect the shell-breaking strengths and shell thickness. indicated that hens received diet without phytase had more shell thickness, furthermore, addition of phytase to the basal diet decreased shell thickness. Iron is necessary for making haemoglobin which is essential for transferring oxygen in blood from the lungs to the tissues. Life of animal can be endangered by reducing available oxygen of tissues (hypoxia). Heart increases flow of blood to the lungs for oxygenation to prevent hypoxia but increasing of blood flow causes heart hypertrophy. Aoyagi and Baker (Citation1995) reported the heart hypertrophy in chicks fed by diets severely deficient in iron. The heart weight was not affected by iron levels in our study that may be due to the high age of hens. In this research, the high spleen weight and the low heart weight were observed in hens fed diet supplemented with phytase. Phytase can influence on the carcass traits because of high impact of it on the availability of minerals, fat, protein, amino acids and starch. Mohamed Arabi (Citation2013) stated that phytase did not have significant effect on heart and liver percentage. Concentrations of mineral tissue are commonly used to evaluate the mineral status of animals and humans. In this experiment, we found linear responses in the concentrations of iron in liver, spleen and bone marrow by increasing levels of iron. Our findings are similar to (Aoyagi & Baker Citation1995; Cao et al. Citation1996; Yu et al. Citation2000) results that observed that the iron concentration of tissues enhances with increasing of dietary iron. Ma et al. (Citation2012) suggested that adding 120 and 160 mg iron/kg from iron–glycine and 160 mg iron/kg from FeSO4 increases iron concentration in serum, liver, breast muscle, tibia and faeces at 21 and 42 days of broiler age. The poor iron status will lead to embryonic malformations and delayed development and also it can cause death in post-hatch. One of embryonic sign in mortality of post-hatch is heart hypertrophy (Tako & Glahn Citation2011). In this experiment, significant effect of dietary iron on iron content of egg yolk was observed, which agree with (Morck & Austic Citation1981; Park et al. Citation2004; Bess et al. Citation2012) the findings. Park et al. (Citation2004) found that iron content of eggs increased by 5% and 18% by the addition of either FeSO4 and Fe-methionine, respectively. Hens fed diets with phytase enhanced the iron concentration of the egg yolk and blood serum. This result showed that phytase can release iron from inositol in old broiler breeder hens and increases chicken iron reserve. The result showed that 600 U/kg of phytase supplementation led to an increase in iron concentration of 6% in egg yolk and 18.7% in blood serum. Ao et al. (Citation2007) stated that adding phytase to diet increase zinc concentration in blood plasma and bone marrow of broiler. Statistical methods used to determine the iron requirement can be effective on the amount of iron requirements estimation.
5. Conclusion
The present study showed that phytase increased iron bioavailability, and the supplements of iron provided as organic iron in the semi-purified diet required for hen house egg production of broiler breeder hens at late stage of production cycle were 65 and 58 mg/kg without and with phytase supplementation, respectively. Based on our data on hen house egg production, about 1.16 mg of iron was released per 100 U/kg of phytase, which means that 7 mg iron/kg will be released if 600 U/kg of phytase is used.
References
- Ao T, Pierce J, Pescatore A, Cantor A, Dawson K, Ford M, Shafer B. 2007. Effects of organic zinc and phytase supplementation in a maize–soybean meal diet on the performance and tissue zinc content of broiler chicks. Br Poult Sci. 48:690–695. 10.1080/00071660701694072
- Aoyagi S, Baker DH. 1995. Iron requirement of chicks fed a semipurified diet based on casein and soy protein concentrate. Poult Sci. 74:412–415. 10.3382/ps.0740412
- Augspurger N, Baker D. 2004. High dietary phytase levels maximize phytate-phosphorus utilization but do not affect protein utilization in chicks fed phosphorus-or amino acid-deficient diets. J Anim Sci. 82:1100–1107.
- Baker DH. 1986. Problems and pitfalls in animal experiments designed to establish dietary requirements for essential nutrients. J Nutr. 116:2339–2349.
- Bess F, Vieira SL, Favero A, Cruz RA, Nascimento PC. 2012. Dietary iron effects on broiler breeder performance and egg iron contents. Anim Feed Sci Technol. 178:67–73. 10.1016/j.anifeedsci.2012.10.002
- Bitar K, Reinhold JG. 1972. Phytase and alkaline phosphatase activities in intestinal mucosae of rat, chicken, calf, and man. Biochim Biophys Acta (BBA)-Enzymol. 268: 442–452. 10.1016/0005-2744(72)90340-3
- Cao J, Luo XG, Henry PR, Ammerman CB, Littell RC, Miles RD. 1996. Effect of dietary iron concentration, age, and length of iron feeding on feed intake and tissue iron concentration of broiler chicks for use as a bioassay of supplemental iron sources. Poult Sci. 75:495–504. 10.3382/ps.0750495
- Casartelli EM, Junqueira O, Laurentiz A, Filardi R, Lucas Júnior J, Araujo L. 2005. Effect of phytase in laying hen diets with different phosphorus sources. Rev Bras Ciênc Avícola. 7:93–98.
- Chio LF, Bunden K, Vohra P, Kratzer FH. 1976. An abnormal requirement for iron in dystrophic chickens. Poult Sci. 55:808–813. 10.3382/ps.0550808
- Cosgrove D. 1966. The chemistry and biochemistry of inositol phosphates. Rev Pure Appl Chem. 16:209–224.
- Davis PN, Norris LC, Kratzer FH. 1962. Iron deficiency studies in chicks using treated isolated soybean protein diets. J Nutr. 78:445–453.
- De Boland AR, Garner GB, O'Dell BL. 1975. Identification and properties of phytate in cereal grains and oilseed products. J Agric Food Chem. 23:1186–1189. 10.1021/jf60202a038
- Hallberg L, Hulthén L. 2000. Prediction of dietary iron absorption: an algorithm for calculating absorption and bioavailability of dietary iron. Am J Clin Nutr. 71:1147–1160.
- Hallberg L, Rossander L, Skånberg A. 1987. Phytates and the inhibitory effect of bran on iron absorption in man. Am J Clin Nutr. 45:988–996.
- Hurrell R. 1997. Optimizing iron compounds and bioavailability. Eur J Clin Nutr. 51:S4–S8.
- Hurrell RF, Reddy MB, Juillerat M-A, Cook JD. 2003. Degradation of phytic acid in cereal porridges improves iron absorption by human subjects. Am J Clin Nutr. 77:1213–1219.
- Lei X, Ku P, Miller E, Yokoyama M. 1993. Supplementing corn-soybean meal diets with microbial phytase linearly improves phytate phosphorus utilization by weanling pigs. J Anim Sci. 71:3359–3367.
- Liebert F, Htoo J, Sunder A. 2005. Performance and nutrient utilization of laying hens fed low-phosphorus corn-soybean and wheat-soybean diets supplemented with microbial phytase. Poult Sci. 84:1576–1583. 10.1093/ps/84.10.1576
- Lim H, Namkung H, Paik I. 2003. Effects of phytase supplementation on the performance, egg quality, and phosphorous excretion of laying hens fed different levels of dietary calcium and nonphytate phosphorous. Poult Sci. 82:92–99. 10.1093/ps/82.1.92
- Ma WQ, Sun H, Zhou Y, Wu J, Feng J. 2012. Effects of iron glycine chelate on growth, tissue mineral concentrations, fecal mineral excretion, and liver antioxidant enzyme activities in broilers. Biol Trace Elem Res. 149:204–211. 10.1007/s12011-012-9418-5
- Maga JA. 1982. Phytate: its chemistry, occurrence, food interactions, nutritional significance, and methods of analysis. J Agric Food Chem. 30:1–9. 10.1021/jf00109a001
- McDowell LR. 2003. Minerals in animal and human nutrition. Amsterdam: Elsevier Science BV.
- McNaughton JL, Day EJ. 1979. Effect of dietary Fe to Cu ratios on hematological and growth responses of broiler chickens. J Nutr. 109:559–564.
- Mohamed Arabi SA. 2013. Effect of dietary phytase on protein and electrolyte utilization on broiler chicks production. Sci Int. 1:15–21. 10.5567/sciintl.2013.15.21
- Morck TA, Austic RE. 1981. Iron requirements of White Leghorn hens. Poult Sci. 60:1497–1503. 10.3382/ps.0601497
- Park SW, Namkung H, Ahn HJ, Paik IK. 2004. Production of iron enriched eggs of laying hens. Australas J Anim Sci. 17:1725–1728.
- Parsons CM. 1996. Digestible amino acids for poultry and swine. Anim Feed Sci Technol. 59:147–153.
- Pesti GM, Vedenov D, Cason JA, Billard L. 2009. A comparison of methods to estimate nutritional requirements from experimental data. Br Poult Sci. 50:16–32. 10.1080/00071660802530639
- Pollitt E, Leibel RL. 1976. Iron deficiency and behavior. J Pediatr. 88:372–381. 10.1016/S0022-3476(76)80250-8
- Ramadan NA, Omar AS, Bahakaim ASA, Osman SM. 2010. Effect of using different levels of iron with zinc and copper in layer's diet on egg iron enrichment. Int J Poult Sci. 9:842–850. 10.3923/ijps.2010.842.850
- Reichmann H, Janetzky B, Riederer P. 1995. Iron-dependent enzymes in Parkinson's disease. J Neural Transm Suppl. 46:157–164.
- Robbins K, Saxton A, Southern L. 2006. Estimation of nutrient requirements using broken-line regression analysis. J Anim Sci. 84:E155–E165.
- SAS Institute. 2002. The SAS system for windows 2002 release 9.2. Cary (NC): SAS Institute Inc.
- Selle PH, Ravindran V. 2007. Microbial phytase in poultry nutrition. Anim Feed Sci Technol. 135:1–41. 10.1016/j.anifeedsci.2006.06.010
- Shelton JL, Southern LL. 2006. Effects of phytase addition with or without a trace mineral premix on growth performance, bone response variables, and tissue mineral concentrations in commercial broilers. J Appl Poult Res. 15:94–102. 10.1093/japr/15.1.94
- Simons P, Versteegh H, Jongbloed AW, Kemme P, Slump P, Bos K, Wolters M, Beudeker R, Verschoor G. 1990. Improvement of phosphorus availability by microbial phytase in broilers and pigs. Br J Nutr. 64:525–540. 10.1079/BJN19900052
- Stahl C, Han Y, Roneker K, House W, Lei X. 1999. Phytase improves iron bioavailability for hemoglobin synthesis in young pigs. J Anim Sci. 77:2135–2142.
- Tako E, Glahn RP. 2011. Iron status of the late term broiler (Gallus gallus) embryo and hatchling. Int J Poult Sci. 10:42–48. 10.3923/ijps.2011.42.48
- Vahl HA, Van T Klooster AT. 1987. Dietary iron and broiler performance. Br Poult Sci. 28:567–576. 10.1080/00071668708416992
- Yu B, Huang WJ, Chiou PW. 2000. Bioavailability of iron from amino acid complex in weaning pigs. Anim Feed Sci Technol. 86:39–52. 10.1016/S0377-8401(00)00154-1