Abstract
The objective of this study was to describe the physiological changes in serum protein profile in calves during the first month of life. Seven clinically healthy calves were used in this study. Calves received colostrum and whole milk from their dams by voluntary sucking. The first blood sampling was performed within one hour after birth before colostrum intake (day 0) and then at 1, 2, 7, 14 and 30 days of age. Serum was analysed for total protein concentrations, and for the relative and absolute values of serum protein fractions – albumin, alpha1-(α1), alpha2-(α2), beta1-(β1), beta2-(β2) and gamma-(γ) globulins. The results showed a significant effect of age on total protein concentrations and for all the analysed protein fractions. The concentrations of total proteins, β2- and γ-globulins increased significantly one day after colostrum intake (P < 0.001) and then significantly decreased gradually till the end of the first month of age. An opposite trend was observed in the relative concentrations of albumin (P < 0.001). Significant decrease of values was found for relative concentrations of α1-globulins (P < 0.001) and increase of the relative values of α2- and β1-globulins from birth till the end of the monitored period (P < 0.001). The above mentioned changes are also reflected in changes of albumin/globulin ratio (P < 0.001). The results suggest that the serum protein profile in calves is significantly influenced by colostrum intake and the age of calves. This should be taken into consideration when interpreting the serum protein profile in young animals.
1. Introduction
The neonatal period in calves as the most critical period is also known as the adaptive period, when the development of physiological functions and the adaptation processes to the extra-uterine life occur (Piccione et al. Citation2010; Skrzypczak et al. Citation2011). Calves are born with no immunity against disease, so must depend on their dam to provide passive immunity through colostrum. Colostrum and subsequently milk provide a complete diet with all the essential nutrients for the neonate during the initial phase of its life. In ruminants, colostrum is also the sole source of initial acquired immunity for the offspring. The concentration of immunoglobulins is in colostrum particularly high, with IgG being the major immunoglobulin class present in ruminant milk (Stelwagen et al. Citation2009). Colostrum is important for sufficient passive immunity and for enhancing developmental changes and improving postnatal metabolism in calves (Kühne et al. Citation2000). Consumption quantity of good quality colostrum to provide proper circulating concentrations of immunoglobulins G (IgG) prior to the cessation of macromolecular transport is essential to ensure healthy calves with immunity against disease (Nowak & Poindron Citation2006). In addition to immunoglobulins the protein fraction in bovine colostrum comprises bioactive components such as major milk proteins, hormones, growth factors and cytokines. Due to the presence of large amounts of these compounds, colostrum has a beneficial influence on newborn calves during the first days after calving. However, the concentrations of many these proteins decrease over the time after calving (Sobczuk-Szul et al. Citation2013). Thus, the chemical and immunological quality of colostrum decrease after the first milking (Moreno-Indias et al. Citation2012). The determination of serum proteins is a useful and practical means of assessing immunoglobulin absorption in the neonatal calf.
Serum protein electrophoresis is a simple, reliable and specific method for the separation of different protein fractions. Weight and charge of different protein fractions separates these into distinct bands, comprising albumin, alpha (α), beta (β) and gamma (γ) globulins (Vaden et al. Citation2009). Serum protein electrophoresis is used to evaluate, diagnose and monitor a variety of diseases, and provides important diagnostic information for further definitive studies of the patient (Alberghina et al. Citation2010). On the other hand, several physiological factors may affect serum protein levels, including the age of the evaluated animals (Weaver et al. Citation2000; Eckersall Citation2008). Several studies confirmed that changes in nutrition in young animals, body growth and development are accompanied by dynamic changes in many indices of haematological, protein, mineral, enzymatic, energy and other profiles, including serum protein profile (Egli & Blum Citation1998; Mohri et al. Citation2007). Therefore, age-related reference values should be ideal for interpreting serum protein pattern, but such data in calves rarely are available.
The objective of this study was to evaluate the changes in the serum profile and concentrations of serum protein fractions in clinically healthy calves during the first month of life. The aim of the study was to investigate and characterize the physiological changes in serum proteins in calves during the process of neonatal adaptation to postnatal life including precolostral, colostral and milk feeding period.
2. Material and methods
2.1. Animals and blood sample collection
Seven clinically healthy calves (four males and three females) of a low-land black spotted breed and its crossbreeds were included into this study. The calves were born at the Clinic for Ruminants of the University of Veterinary Medicine and Pharmacy in Kosice with an average birth weight of 36.1 ± 2.3 kg and an average body weight of 64.8 ± 5.5 kg at the end of the first month. Calves were born as singles from three cows and four heifers with pregnancies of normal length and uncomplicated births. After birth they were kept individually with their dams in pens and were stabled on straw litter. Calves were allowed to suck their dams voluntarily and the first colostrum they received within two hours after birth. Subsequently until the end of the study the calves received colostrum and whole milk by voluntary sucking of their dams. The calves had also free access to water and hay during the time under study. The health status of the calves was evaluated daily. Clinical examination included behaviour, appetite, rectal temperature, heart and respiratory rates, pulmonary sounds, nasal discharge, eye discharge, navel infection and faecal consistency. All animals were clinically healthy and in good general condition without any obvious clinical signs of diseases during the time of the study.
The first blood sampling in calves was performed before the colostrum intake (precolostral sample, day 0) within one hour after birth and then at 1, 2, 7, 14 and 30 days of age. Blood samples were collected by direct jugular vein puncture into tubes with serum cloth activator (Meus, Piove di Sacco, Italy). Blood samples were allowed to clot at room temperature and then centrifuged at 3000 × g for 30 minutes to separate serum. The harvested blood serum was dispensed into plastic tubes and stored at –20°C until analysed.
2.2. Laboratory analyses
In blood serum the concentrations of total proteins (TP, g/l) and serum protein fractions were analysed. Total protein concentrations were assessed on an automated biochemical analyser Alizé (Lisabio, France) by the biuret method using commercial diagnostic kits (Randox, UK). Serum protein fractions were separated by zone electrophoresis on a buffered agarose gel at pH 8.8 on an automated electrophoresis system Hydrasys (Sebia Corporate, France) using commercial diagnostic kits Hydragel 7 Protein (Sebia Corporate, France) according to the procedure described by the manufacturer. The electrophoretic gels were scanned, and the serum protein fractions were visualized and displayed on densitometry system Epson Perfection V700 (Epson America Inc., CA, USA) by light transmission and automatic conversion into an optical density curve presentation. Protein fractions were identified and quantified by computer software Phoresis version 5.50 (Sebia Corporate, France) and, if necessary, corrected by visual inspection of the electrophoretogram.
Serum proteins were separated into the following fractions in order of fastest to slowest mobilities: albumin (Alb), alpha1 (α1)-, alpha2 (α2)-, beta1 (β1)-, beta2 (β2)- and gamma (γ)-globulins. The relative concentrations (%) of the protein fractions were determined as the percentage of the optical absorbance, and the absolute concentrations (g/l) were calculated from the total serum protein concentrations. Albumin/globulin ratios (A/G) were computed from the electrophoretic scan.
2.3. Statistical analyses
Arithmetic means (x) and standard deviations (SD) expressed as relative and absolute concentrations for each evaluated variable and sample collection were calculated using descriptive statistical procedures. The significance of the effect of age on the concentrations of the analysed variables during the whole monitored period and between the collection points was examined by non-parametric Friedman’s rank sum test (P value Dunn’s Multiple Comparisons test using the programme GraphPad Prism V5.02 (GraphPad Software Inc., CA, USA).
3. Results
Serum protein electrophoresis identified six distinct bands, comprising albumin, two α-globulin (α1 and α2), two β-globulin (β1 and β2) and γ-globulin fractions, in calves ( and ; ). Albumin was the most prominent protein fraction and at birth constituted in average 58.1% of total serum proteins (). Its relative concentrations decreased significantly one day after colostrum intake (P < 0.001). From the second day till the end of the first month of life a gradual significant increase of the values was observed (P < 0.001). However the percentages of albumin fraction 30 days after birth did not reach the values found before the intake of colostrum. Sampling time had a significant effect on the relative concentrations of α1-globulins (P < 0.001). Among the globulins this fraction was present in precolostral serum in the highest ratio. After colostrum intake the values decreased significantly from day 1 up to day 30 of life (). An opposite trend of significantly increasing values was observed in the relative concentrations of α2-globulins (P < 0.001). The mean values at birth and one day after colostrum intake were roughly uniform. An increase of α2-globulins was found from day 2 after birth with the significantly highest mean value at the age of 30 days (). Significant age-related changes during the first month of life were found also for β1- and β2-globulins (P < 0.001). The lowest mean relative concentration of β1-globulins was found at birth. Their values increased gradually from the first day after birth till the end of the study (). The significantly highest mean relative concentration of β1-globulins was found on day 14. At birth, the relative mean concentration of β2-globulins was very low (1.2%). Their concentrations increased markedly already one day after colostrum intake (P < 0.001), with a subsequent decrease of values from the second day of life (; P < 0.05). At birth, the mean relative concentration of γ-globulins was only 1.3% and increased significantly one day after colostrum intake (P < 0.001). Since the second day after birth a gradual significant decrease of values was found till the end of the study (). The A/G ratios showed significant changes during the time of observation (P < 0.001). The highest mean value was recorded before colostrum intake (1.4) and the significantly lowest on the first day after birth (0.32). Since the second day after birth gradual increase of A/G ratio values was found ().
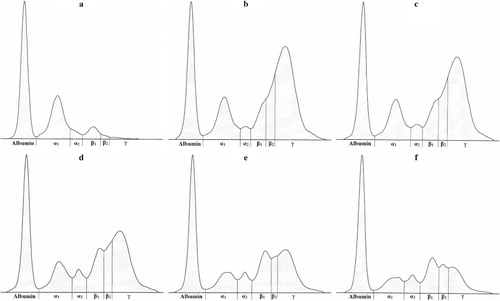
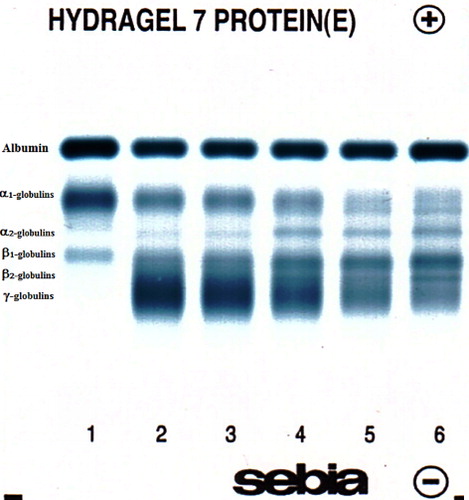
Table 1. The changes of the relative concentrations of serum protein fractions (%) and albumin/globulin ratios (A/G) in calves during the first month of life (mean ± SD).
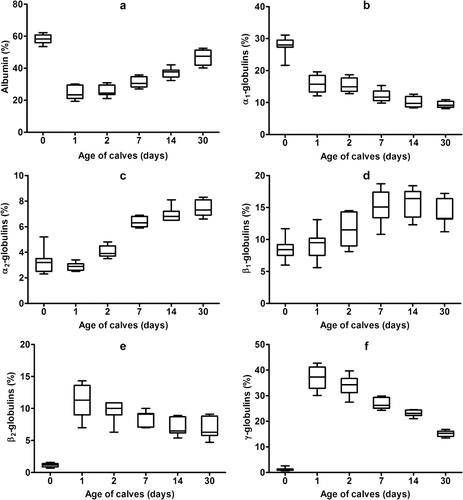
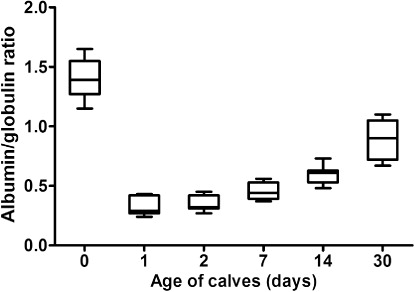
The concentrations of total proteins in relation to the age of the calves showed significant changes during the first month of life (, P < 0.001). The lowest mean concentration was observed at birth (41.1 g/l), which was followed by a marked significant increase one day after colostrum intake (mean 85.2 g/l; P < 0.001). Thereafter, the values significantly decreased gradually until the end of the evaluated period (; P < 0.05). Significant changes during the evaluated time were found in the absolute concentrations of albumin (P < 0.001). A slight non-significant decrease of values one day after birth was followed by subsequent gradual significant increase of values till the end of the study (P < 0.05 and P < 0.01). An opposite trend was observed in the absolute concentrations of α1-globulins, with a temporary non-significant increase of values one and two days after birth and subsequent significant gradual decrease till the end of the study (P < 0.001). The lowest mean value of α2-globulins was found before colostrum intake. Since the first day of life, a gradual significant increase of α2-globulin values was observed till the 14th day of life (P < 0.001). Significant age course changes were found also in the absolute concentrations of β1-globulins (P < 0.001). The significantly lowest mean value of β1-globulins was recorded at birth before colostrum intake. From the day 1 after birth, a gradual increase of their values was recorded, with the significantly highest mean concentration on the seventh day of life (P < 0.001). The significantly lowest mean value of β2-globulins was found at birth (0.5 g/l). One day after colostrum intake, their concentrations increased markedly, with a subsequent significant decrease till the end of the first month of life (P < 0.01). Similar trends of changes were also observed in the concentrations of γ-globulins (P < 0.001). Before colostrum intake the mean absolute concentration of γ-globulins was only 0.5 g/l. The significantly highest values were recorded one day after the animals were fed colostrum (mean 32.1 g/l; P < 0.001). Since the second day significant decrease of γ-globulins was found till the end of the study.
Table 2. The changes of the concentrations of serum total proteins (TP) and protein fractions (g/l) in calves during the first month of life (mean ± SD).
4. Discussion and conclusion
New tools, such as proteomics, enable the comprehensive analysis of proteins present in any biological compartments, e.g. blood plasma or milk also in ruminants (Argüello Citation2011). However, the studies focused on the analysis of blood proteins in calves during the early postnatal period are rather sparse, while the most intensive adaptational changes of the newborn calves occur during this period. The numerous functional, morphological and metabolic alterations are reflected also by biochemical changes, including changes in blood serum proteins (Kühne et al. Citation2000). Various studies described the protein electrophoretic pattern in bovine blood serum (Žvorc et al. Citation2000; Alberghina et al. Citation2011). However, these investigations were carried out on adult animals, and there are only few data concerning the serum protein electrophoretic pattern of neonatal calves and without analysing precolostral blood samples. Thus, there is a lack of data related to the physiological changes in protein profile using protein electrophoretic fractions as well occurring shortly after the first colostrum intake.
The results of this study showed a significant effect of colostrum intake and age on the concentrations of total serum proteins and the evaluated protein fractions. The most significant changes occurred between the time before colostrum intake (day 0) and 24 hours after the first colostrum feeding (day 1). The low concentration of total serum proteins observed in calves at birth was followed by their rapid and marked increase after colostrum intake. The mean serum concentration of total proteins increased significantly by more than twice from 41.1 g/l on day 0 to 85.2 g/l on day 1. After this time the values decreased notably till the end of the study. Similar results were recorded in lambs by Loste et al. (Citation2008). Hammon et al. (Citation2002) reported that at birth, the serum protein concentrations of most animals are quite low due to the minimal quantities of immunoglobulins and the increase in its values during the first 24 hours of life reflects the intestinal absorption of proteins (particularly immunoglobulins) from colostrum due to the enhanced intestinal permeability. Piccione et al. (Citation2009) found also in calves the highest total protein concentrations on the first day of life. On the other hand, our results showed from the second day of life gradually decreasing values of total proteins until the end of the first month. At the end of the study the mean serum protein concentrations were lower almost by 20 g/l. The reason for this effect was probably the degradation of colostral immunoglobulins (Mohri et al. Citation2007).
Various studies showed in calves progressive increase of albumin concentrations from birth till the age of 80th day (Knowles et al. Citation2000; Ježek et al. Citation2006; Piccione et al. Citation2009). According to Davis et al. (Citation1998), the increase of albumin together with the age is a physiological phenomenon and higher concentrations of albumin in young and growing animals help to maintain the metabolic balance. Our results showed in calves initially a decrease of the concentrations of albumin first two days after birth. This was followed by gradual increase of the values till the end of the first month of life. The highest relative values were observed after birth. This was followed by more than 50% decrease one day after colostrum intake and an increase of values from day 2 after birth. According to Thomas (Citation2000), this pattern is caused by changing globulin concentrations during the development (higher relative concentrations of albumin and lower percentages of γ-globulins in very young animals). The changes in albumin concentrations reflect the albumin’s medium half-life that ranges from 14 to 16 days in ruminants, after which period the liver is responsible for albumin synthesis (Lassen Citation2004).
Significant age-related variations were seen for the concentrations of α1-globulins with the highest relative values at birth before colostrum intake. This component of globulins comprised almost 30% of total proteins. One day after colostrum feeding the mean relative concentration decreased by almost 50% and subsequent decrease of the values was observed till the end of the first month of life. Similar findings were reported by Piccione et al. (Citation2009). Szewczuk et al. (Citation2011) found in calves higher concentrations of α-globulins on day 5 compared with values measured on day 30 of life. Many of the acute phase proteins migrate in the α-globulin region (haptoglobin, ceruloplasmin, α1-acid glycoprotein, α1-antitrypsin), so that increases in this fraction may be associated with inflammatory status and stimulation to various stressors (Kaneko Citation1997). However, higher concentrations of α1-globulins in calves shortly after birth are not necessarily a sign of the activation of inflammatory processes nor a sign of a disease. Kaneko (Citation1997) reported that blood sera of newborn and young animals contain large amounts of α-globulins due to higher concentrations of some of the proteins from this fraction, which have to protect young animals from immunologic attacks. According to Bishop et al. (Citation2010), α1-fetoprotein represents one of these proteins, which is synthesized in the developing foetus and then by the parenchymal cells of the liver. Its serum concentrations decrease progressively after birth and normal adult values are usually achieved at the age of 8–12 months (Mizejewski Citation2001). Therefore, higher relative concentrations of α1-globulins in calves after birth recorded in our study may reflect the accumulation of α1-fetoprotein in the blood serum of young animals. An opposite trend was recorded in the changes of the concentrations of α2-globulin. This fraction includes some acute phase proteins, including α2-macroglobulin and serum amyloid A. According to Lomborg et al. (Citation2008), not only inflammatory stimuli, but also environmental conditions represent an important trigger for the shift in acute phase proteins, and some increases in the concentrations of α-globulins may be present in conditions not related to inflammatory diseases. Therefore, increasing values of α2-globulins in calves with age obtained in our study may be associated with the normal process of growth and may be related to the exposure of animals to changing environmental conditions and nutritional factors.
Similarly, the β1-globulins showed a progressive increase of values from the first day of life till the end of the study. These findings may be related to the increase of some proteins, especially complement, from this fraction which like the acute phase proteins is involved in the environmental stress response (Bernabucci et al. Citation2009). Moreover, the increase of β1-globulins with age may be attributed to an increase of exposure to ambiental agents in animals with advancing age (França et al. Citation2011). The lowest mean concentrations among the globulins were found in β2- and γ-globulins (1.2% and 1.3%, respectively). One day after colostrum feeding there were marked changes in their values with almost 10- and 30-fold increase of relative concentrations of β2- and γ-globulins, respectively. After this time till the end of the study there was a trend of continuing decrease of their values. Beta2-lipoprotein, transferrin or lactoferrin and, occasionally, immunoglobulin molecules of the IgM and IgA type migrate in the fraction of β2-globulins. Lactoferrin is present in the blood serum in relatively low concentrations, but higher concentrations were determined in bovine milk (Adlerova et al. Citation2008; Giacinti et al. Citation2013). Moreover, lactoferrin concentrations are very high in colostrum and newborn animals (Sobczuk-Szul et al. Citation2013). Therefore, the rapid increase of the concentrations of β2-globulins in calves one day after birth may be caused by the absorption of lactoferrin from colostrum. The proteins that compose the γ-globulin fraction are principally the immunoglobulins. Presented study indicated significant effect of colostrum intake and age also on this fraction. The changes in the serum protein electrophoretic pattern after birth reflect the absorption of immunoglobulins with colostral uptake during the first 24 hours after birth. In calves, precolostral serum normally contains no γ-globulins, but within a few hours after ingestion of colostrum, γ-globulins appear in serum and the absorption continues according to various authors for up to 36–48 hours after birth, after which gut permeability ceases (Weaver et al. Citation2000; Castro-Alonso et al. Citation2008). Immunoglobulins absorbed from colostrum provide the humoral immunity for the newborn animals until they can produce immunoglobulins on their own in sufficient amounts to provide protection against infectious agents (Meyer & Harvey Citation2004; Blum Citation2006). Piccione et al. (Citation2009) recorded in calves a decrease of γ-globulins from day 1 till day 15, with a subsequent small increase of values from day 20, which can be related to the development of the lymphoid function. Our results showed in calves a longer lasting and progressive decrease of γ-globulin concentrations from day 2 after birth till the end of the first month of age, probably attributed to the degradation of colostrum-derived immunoglobulins and only a slow gradual initiation of the production of own immunoglobulins by the maturing immune system. Presented results suggest a marked shift in the concentrations of albumin and globulins in calves during the first month of life, presumably caused by changing globulin pattern during the growth and development. These changes reflect also the marked variations found in the A/G ratios. According to Alberghina et al. (Citation2010), the A/G ratio must be interpreted cautiously with attention paid to which part of the ratio has changed because it allows systematic classification and identification of dysproteinemias.
In conclusion, our results showed that the serum protein electrophoretic patterns are markedly and significantly influenced by the age, growth and development of the calves. Significant changes were found in all evaluated variables during the whole study period. They reflect the response of the calves to colostrum intake after birth and changes in nutrition and homeostasis during the neonatal period. These changes suggests that the age of evaluated animals should be taken into consideration when interpreting serum protein profile. Thus, presented results contribute to the knowledge about the electrophoretic pattern of serum proteins profile in young calves and to the widening of the physiological values starting with precolostral period shortly after birth. The changes in serum protein electrophoretic profile may indicate not only various diseases, but modifications of the proteinogram in calves during the first 30 days of life may be associated with physiological changes and adaptation processes. The results confirm the need for age specific reference values also for these variables when considering precise interpretation of laboratory results with respect to dysproteinemias. The obtained data would be useful for clinicians in the evaluation of dysproteinemias and various pathological conditions, including neonatal calf disease providing a basis for further specific laboratory investigations.
Disclosure statement
No potential conflict of interest was reported by the authors.
Disclosure statement
This work was supported by VEGA Scientific Grants [grant number 1/0447/14], [grant number 1/0154/15] from the Ministry of Education.
References
- Adlerova L, Bartoskova A, Faldyna M. 2008. Lactoferrin: a review. Vet Med Czech. 53:457–468.
- Alberghina D, Casella S, Vazzana I, Ferrantelli V, Giannetto C, Piccione G. 2010. Analysis of serum proteins in clinically healthy goats (Capra hircus) using agarose gel electrophoresis. Vet Clin Pathol. 39:317–321.10.1111/j.1939-165X.2010.00226.x
- Alberghina D, Giannetto C, Vazzana I, Ferrantelli V, Piccione G. 2011. Reference intervals for total protein concentration, serum protein fractions, and albumin/globulin ratios in clinically healthy dairy cows. J Vet Diagn Invest. 23:111–114.10.1177/104063871102300119
- Argüello A. 2011. Trends in goat research, a review. J Appl Anim Res. 39:429–434.
- Bernabucci U, Lacetera N, Danieli PP, Bani P. 2009. Influence of different periods of exposure to hot environment on rumen function and diet digestibility in sheep. Int J Biometeorol. 53:387–395.
- Bishop ML, Fody EP, Schoeff LE, editors. 2010. Clinical chemistry: techniques, principles, correlations. Philadelphia (PA): Lippincott Williams & Wilkins; p. 205–245.
- Blum JW. 2006. Nutritional physiology of neonatal calves. J Anim Physiol Anim Nutr. 90:1–11.10.1111/j.1439-0396.2005.00614.x
- Castro-Alonso A, Castro N, Capote J, Morales-delaNuez A, Moreno-Indias I, Sánchez-Macias D, Herraez P, Argüello A. 2008. Short communication: apoptosis regulates passive immune transfer in newborn kids. J Dairy Sci. 91:2086–2088.10.3168/jds.2007-0814
- Davis TA, Burrin DG, Fiorotto ML, Reeds PJ, Jahoor F. 1998. Roles of insulin and amino acids in the regulation of protein synthesis in the neonate. J Nutr. 128:347–350.
- Eckersall PD. 2008. Proteins, proteomics, and the dysproteinemias. In: Kaneko JJ, Harvey JW, Bruss ML, editors. Clinical biochemistry of domestic animals. 6th ed. London: Elsevier Academic Press; p. 117–155.
- Egli CP, Blum JW. 1998. Clinical, haematological, metabolic and endocrine traits during the first three months of life of suckling Simentaler calves held in a cow-calf operation. J Vet Med A. 45:99–118.10.1111/j.1439-0442.1998.tb00806.x
- França RT, Costa MM, Martins DB, Pagnoncelli M, Leal ML, Mazzanti CM, Palma HE, Kunert CP, Paim FC, dos Anjos Lopes ST. 2011. Protein profile of buffaloes of different ages. Acta Sci Vet. 39:995–999.
- Giacinti G, Basirico L, Ronchi B, Bemabucci U. 2013. Lactoferrin concentration in buffalo milk. Italian J Anim Sci. 12:139–143.10.4081/ijas.2013.e23
- Hammon HM, Schiessler G, Nussbaum A, Blum JW. 2002. Feed intake patterns, growth performance and metabolic and endocrine traits in calves fed unlimited amounts of colostrum and milk by automate starting in the neonatal period. J Dairy Sci. 85:3352–3362.10.3168/jds.S0022-0302(02)74423-8
- Ježek J, Klopčič M, Klinkon M. 2006. Influence of age on biochemical parameters in calves. Bull Vet Inst Pulawy. 50:211–214.
- Kaneko JJ. 1997. Serum proteins and the dysproteinemias. In: Kaneko JJ, editor. Clinical biochemistry of domestic animals. 5th ed. London: Academic Press; p. 117–138.
- Knowles TG, Edwards JE, Bazeley KJ, Brown SN, Butterworth A, Warriss PD. 2000. Changes in the blood biochemical and haematological profile of neonatal calves with age. Vet Rec. 147:593–598.10.1136/vr.147.21.593
- Kühne S, Hammon HM, Bruckmaier RM, Morel C, Zbinden Y, Blum J. 2000. Growth performance, metabolic and endocrine traits and absorptive capacity in neonatal calves fed either colostrum or milk replacer at two levels. J Anim Sci. 78:609–620.
- Lassen ED. 2004. Laboratory evaluation of plasma and serum proteins. In: Thrall MA, editor. Veterinary hematology and clinical chemistry. Philadelphia (PA): Lippincott Williams & Wilkins; p. 401–415.
- Lomborg SR, Nielsen LR, Heegaard PMH, Jacobsen S. 2008. Acute phase proteins in cattle after exposure to complex stress. Vet Res Commun. 32:575–582.10.1007/s11259-008-9057-7
- Loste A, Ramos JJ, Fernández A, Ferrer LM, Lacasta D, Verde MT, Marca MC, Ortín A. 2008. Effect of colostrum treated by heat on immunological parameters in newborn lambs. Livest Sci. 117:176–183.10.1016/j.livsci.2007.12.012
- Meyer DJ, Harvey JW, editors. 2004. Veterinary laboratory medicine: interpretation and diagnosis. 3rd ed. St. Louis: Saunders; p. 156–168.
- Mizejewski GJ. 2001. Alpha-fetoprotein structure and function: relevance of isoforms, epitopes, and comformational variants. Exp Biol Med. 226:377–408.
- Mohri M, Sharifi K, Eidi S. 2007. Hematology and serum biochemistry of Holstein dairy calves: Age related changes and comparison with blood composition in adults. Res Vet Sci. 83:30–39.10.1016/j.rvsc.2006.10.017
- Moreno-Indias I, Sánchez-Macías D, Castro N, Morales-delaNuez A, Hernández-Castellano LE, Capote J, Argüello A. 2012. Chemical composition and immune status of dairy goat colostrum fractions during the first 10 h after partum. Small Ruminant Res. 103:220–224.10.1016/j.smallrumres.2011.09.015
- Nowak R, Poindron P. 2006. From birth to colostrum: early steps leading to lamb survival. Reprod Nutr Dev. 46:431–446.10.1051/rnd:2006023
- Piccione G, Casella S, Giannetto C, Vazzana I, Niutta PP, Giudice A. 2009. Influence of age on profile of serum proteins in the calf. Acta Vet. 59:413–422.10.2298/AVB0904413P
- Piccione G, Casella S, Pennisi P, Giannetto C, Costa A, Caola G. 2010. Monitoring of physiological and blood parameters during perinatal and neonatal period in calves. Arq Bras Med Vet Zootec. 62:1–12.10.1590/S0102-09352010000100001
- Skrzypczak WF, Ozgo M, Lepczynski A, Herosimczyk A. 2011. Defining the blood plasma protein repertoire on seven day old dairy calves – a preliminary study. J Physiol Pharmacol. 62:313–319.
- Sobczuk-Szul M, Wielgosz-Groth Z, Wroňski M, Rzemieniewski A. 2013. Changes in the bioactive protein concentrations in the bovine colostrum of Jersey and Polish Holstein-Friesian cows. Turk J Vet Anim Sci. 37:43–49.
- Stelwagen K, Carpenter E, Haigh B, Hodgkinson A, Wheeler TT. 2009. Immune components of bovine colostrum and milk. J Anim Sci. 87(Suppl. 1):3–9.
- Szewczuk M, Czerniawska-Piątkowska E, Palewski S. 2011. The effect of colostral supplement on the serum protein fractions, health status and growth of calves. Archiv Tierzucht 54:115–126.
- Thomas JS. 2000. Overview of plasma proteins and protein electrophoresis. In: Feldman BF, Zinki JG, Jain JC, editors. Schalm’s veterinary haematology. 5th ed. New York: Lippincott Williams & Wilkins; p. 891–904.
- Vaden SL, Knoll JS, Smith SWK, Tilley LP. 2009. Protein electrophoresis. In: Vaden SL, Knoll JS, Smith SWK, Tilley LP, editors. Blackwell’s five minute veterinary consult: laboratory tests and diagnostic procedures. Ames (IA): Wiley-Blackwell; p. 501–503.
- Weaver DM, Tyler JW, VanMetre DC, Hostetler DE, Barrington GM. 2000. Passive transfer of colostral immunoglobulins in calves. J Vet Intern Med. 14:569–577.10.1111/j.1939-1676.2000.tb02278.x
- Žvorc Z, Matijatko V, Beer B, Foršek J, Bedrica L, Kučer N. 2000. Blood serum proteinograms in pregnant and non-pregnant cows. Vet Archiv. 70:21–30.