ABSTRACT
The objective of this study was to investigate the morphological characteristics and expression of the α-smooth muscle actin (ASMA) immunohistochemistry during development of the small intestine in broiler chickens. Twenty-four healthy broiler chickens (ROSS 308) in 4 different age groups (6-, 14-, 28- and 52-day-old) were used in this experiment. A slight increase was observed in the weight and length of the duodenum, jejunum and ileum from days 6 to 28, peaked on day 52. The weight and length of jejunum on days 28 and 52, respectively, were significantly higher in female chickens compared with males (P < .05). An increase in height and width of propria and muscular layers of villi were obtained for the three segments during the experimental period. Villi height and width of jejunum in female broilers at day 28 were significantly larger than males (P < .05). These results also revealed that all the smooth muscle layers were immunoreactive positive for ASMA; but this reactivity differed between the muscle layers. The minimal expression of ASMA was observed at days 6 and 14 of age in circular and longitudinal muscular layers. At day 28, there was a more distinct expression of ASMA in circular and longitudinal muscular layers compared with days 6 and 14. Expression of ASMA in both muscle layers at day 52 was intensely immunopositive. Contractile function during the evolution of each organ and muscle layers could be dependent on different patterns of expression of ASMA in the smooth muscle cells.
1. Introduction
The knowledge about the morphology and histology structures of the normal alimentary canal would help to diagnose and treatment of certain illnesses in animals. Intestine has a prominent physiological role because it is the initial site for digestion and absorption of nutrients (Weisbrodt Citation1987; Guyton Citation1991; Pekas & Wray Citation1991). The gastrointestinal (GI) tract is located between the outside world and the extra-cellular fluid of the body; therefore acting as a permeability barrier. Evidences have shown that large particulates can be transferred from the lumen of the gut across the intestinal epithelium and enter into systemic circulation (Lefevre et al. Citation1985; Jani et al. Citation1992). However, this site can alter during the developmental processes and these alterations are concurrent with changing age in animals. There is a relationship between morphology of the digestive system and age that could be found in the lamina propria of the intestinal mucosa among crypts base and lamina muscularis mucosa (Kanamori et al. Citation1996; Eurell & Frappier Citation2013).
The usual motility of the digestive tract depends on the regular function of the neuromuscular system; therefore, the loss of function causes a change in the intestine motility and abnormal intestine transit (Berseth Citation1996). Actin has contractile and cytoskeletal functions in various types of cells. α-Smooth muscle actin (ASMA) has been one of the six distinct actin isoforms that were previously described (Hartshorne Citation1987). The enteric smooth muscle cells in the chicken contain ASMA molecules even in the gizzard, but the distribution of ASMA varied between the smooth muscle layers and the different regions of the intestine (Yamamoto et al. Citation1996). Rubenstein (Citation1981) identified a small amount of ASMA in smooth muscle cells in the gizzard by electrophoresis.
The present study describes the morphological properties of different regions of broiler small intestine in four age groups and in both sexes. Also, the localization of immunoreactivity for ASMA was examined in the chicken's small intestine to explain the distribution pattern of ASMA molecules.
2. Materials and methods
2.1. Birds and housing
Twenty-four healthy broiler chickens (Ross 308) were used in this study. Birds were in an age range of 6-, 14-, 28- and 52-day-old, with similar environmental and nutritional conditions which were purchased and transferred to the histology division.
2.2. Tissue preparation and sampling
Before sampling, the birds’ body weight (BW) was measured at 6-, 14-, 28- and 52-day-old and then were killed with an overdose intra-peritoneal injection of sodium pentobarbital (5014;mg/ml). The abdominal cavity was immediately exposed and the entire small intestinal tract was dissected. The lumen of the intestinal tracts was flushed with 0.2% formalin fixative. Segments were cut from the duodenum, jejunum and ileum. The samples were then washed in several stages with fresh phosphate buffer (pH 7.2) to eliminate any supplementary particulate material.
Before sectioning the tissue, the arrangement of villi within the different regions of the intestine was examined using a 2× microscope. Examinations showed no difference among the surface architecture of villus between the total bird species. Afterward, all the intestine segments from total birds were cut into three smaller segments with approximately equal length including distal, middle and proximal parts. The samples were placed in a 10% buffered neutral formaldehyde solution (pH 7.2–7.4) and were gradually dehydrated with increasing concentrations of ethyl alcohol (70–100%). The dehydrated specimens were embedded in paraffin, sectioned at 5 µm and stained by using haematoxylin and eosin (Gracia et al. Citation2009).
Figure 1. (a and b) Immunohistochemical stains for α-smooth muscle actin at 6 and 14 days respectively; both demonstrating minimal expression within the bulk of the circular and longitudinal muscles. All of the figures are orientated such that the muscular layers are on the right side.
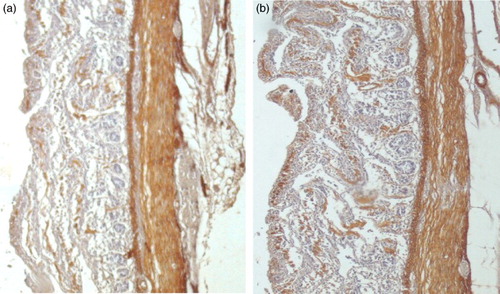
Figure 2. (c and d) Immunohistochemical stains for α-smooth muscle actin at 28 and 52 days respectively; both demonstrating intense expression within the bulk of the circular and longitudinal muscles. All of the figures are orientated such that the muscular layers are on the right side.
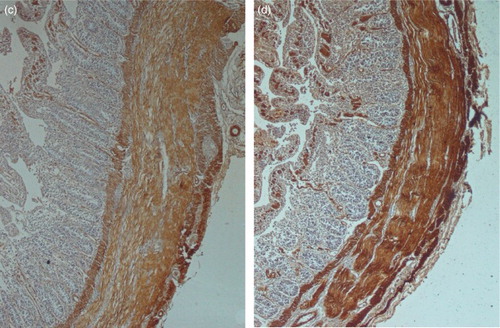
The sections were analysed under a light microscope (Nikon) and the height and width of the villi were measured using an eyepiece micrometer. Villus height was obtained from the top of the villus to the crypt–villus junction, whereas crypt depth was defined as the depth of the invagination between villi (Hu & Guo Citation2007).
2.3. Immunohistochemistry staining
The sections were deparaffinized, hydrated and incubated for 20 min in 0.3% H2O2 in methanol (Gamba et al. Citation2004). The slides were treated with primary antibody: anti ASMA (Dako, Denmark) at 1/100 dilution overnight. Endogenous peroxidase was inhibited using 0.5% hydrogen peroxide in methanol. The sections were then rinsed in buffer solution at pH 7.6. For ASMA antigen, retrieval was performed by placing the sections in a citrate buffer (0.01 M citric acid with 80 g of sodium hydroxide/litre, adjusted to pH 6.0). Sections of ASMA were heated in an oven and immunoreactivity was demonstrated using DAB + (Dako, Denmark). The slides were counterstained with haematoxylin, dehydrated, mounted and then was evaluated by a light microscope and photographed by a stereomicroscope.
3. Results
3.1. Biometric (gross) results
Weights and lengths of the different segments of the small intestine in broilers are shown in and , respectively. Weight and length of the duodenum, jejunum and ileum increased (P < .05) in both sexes as chickens grew from days 6 to 52 ( and ). The jejunum weight and length increased more rapidly than both duodenum and ileum; but overall the increase in weight and length of the duodenum, jejunum and ileum was greater from days 28 to 52 than from days 6 to 28. Weight and length of jejunum at day 28 were significantly increased in female broilers compared with males (P < .05).
Table 1. Weights of various parts of small intestine in chicken.
Table 2. Length of various parts of small intestine in chicken.
The highest weight correlation coefficients were obtained for jejunum (R2 = 0.98) and the lowest was for ileum (R2 = 0.76) (). Weight of all the different segments of intestine in broilers was positively correlated with BW; therefore the derived equation can be used to estimate the weight of different portions of intestine. The BW as the single independent parameter showed a coefficient of determination (R2), underlining the importance of the biometric background for the weight of all the different segments of the intestine ().
Table 3. Regression equations in various parts of intestine parameters in the chicken.
showed the height and width of villi, propria and muscular layer of the chickens duodenum, jejunum and ileum from days 6 to 52.
Table 4. Height and width of villi, propria and muscular layer of various parts of small intestine in chicken.
In both sexes, morphometric results showed that as the age increased (), all the desired parameters in duodenum, jejunum and ileum were increased. Height and width of villi, propria and muscular layer of the jejunum showed the highest value among the other three segments of small intestine. The growth of morphometric parameters for the three segments was more rapid from days 28 to 52 than from days 6 to 28. There was a significant difference in the villi height and width of jejunum between female broilers as compared with males at day 28 (P < .05).
3.2. Immunohistochemistry results
The small intestine specimens from days 6 to 52 showed the difference patterns of expression of ASMA immunoreactivity by using immunohistochemistry (IHC) into smooth muscular cells. Results IHC on days 6 and 14 revealed minimal ASMA expression within muscularis mucosa, circular and longitudinal muscle layers and muscularis propria, with the exception of vessel walls. Results showed that there was relatively intense expression of ASMA in vessel walls in all age groups ()). On 28 and 52 days of age, there was intense expression of ASMA throughout muscularis mucosa, the circular muscle and vessel walls. However, longitudinal muscular layer showed the maximum expression of ASMA than other muscular layers on days 28 and 52 of age. However in the small intestine, the muscularis mucosa and the circular and longitudinal muscle layer were positive for expression of ASMA ()).
3.3. Statistical analysis
All data were analysed using SPSS version 13 for windows. Morphometric findings were compared by one-way analysis of variance, and Fisher‘s least significant difference test was used as a post hoc test. A P < .05 was considered statistically significant. Linear regression was used to estimate the coefficients of the linear equation involving different independent variables that best predicted the value of the dependent variable.
4. Discussion
4.1. Gross and micrometric
Weight and size of different parts of the alimentary tract increases concurrently with an increase in BW during early post hatching than other organs and tissues in chickens (Lilja Citation1981), quails (Lilja et al. Citation1985), turkeys (Sell et al. Citation1991) and ducks (King et al. Citation2000). In the present study, weights and lengths of the duodenum, jejunum and ileum increased more rapidly from days 28 to 52 and the slowest increase was from 6 to 28 days. Liu et al. (Citation2010) showed that in an age range from 0 to 70 days, the mass of the duodenum, jejunum and ileum increased more rapidly from 1 to 28 days in geese. In other investigation, alterations in the small intestine length of the leghorn chicken during development were evaluated from 1 to 84 days by Mitjans et al. (Citation1997). They showed that intestinal length increased in the first weeks of life. Data obtained from the results of these researchers disagree with those reported in our study. Physiological evolution varies between different species of birds and is directly related to nutrient digestion and absorption in the small intestine (Aptekmann et al. Citation2001). It thought that these dramatic developmental changes in the avian gut can be related to changes in nutrient sources from a simplified regime to an exogenous feed ration (Uni et al. Citation1999).
The results of the present study showed that a more distinct increase was observed in the jejunum and ileum than the duodenum during the first two weeks of age. During the immediate postnatal period, the GI tract undergoes profound growth and morphological changes and functional maturation. Numerous studies displayed differences within the digestive canal in birds at various periods. These changes are observable both in gross and micrometrical values in the alimentary canal, which are parallel to the animal's growth and development (Lilja Citation1981; Baranyiova et al. Citation1983; Przystalski Citation1986; Dziala Citation2005). Uni et al. (Citation1999) showed that the mass was increased more in the duodenum and jejunum from 0 to 12 days post hatch in poultry. Other researchers also revealed that intestine length increased more rapidly in the jejunum and ileum during this age period (Gamba et al. Citation2004). Increasing the absorption of entrance food is coordinated by an increase in mass and lengths of the different parts of the small intestine. Results of this investigation showed that the maximum amount of macroscopic characteristics occur in the jejunum segment in the alimentary canal. Therefore, it is suggested that high weight is due to the presence of yolk sac in the jejunum. The yolk sac prepares necessary nutrients for early rapid growth during various ages (Maiorka et al. Citation2003). In another study, Jackson (Citation1992) showed that the intestine length progresses along with BW in 13 species of seabirds.
According to the results of this study, the villi height and width, propria and muscular layer in all segments of the small intestine increased with age and these findings are similar to those of previous studies (Wang & Peng Citation2008; Liu et al. Citation2010). In all age groups, the minimum height and width of villi, propria and muscular layer of the small intestine in both sexes were observed in the ileum, while the maximum values were observed in the jejunum segment. Comparison of the intestinal parameters in both the sexes clearly indicated that in all ages, values of the micrometric indices were greater in female than male. It is supposed that increased morphometric values in villi are paralleled by an increased digestive capacity and increase in the absorbing capability of the intestine due to increased absorptive surface area, expression of brush border enzymes and nutrient transport systems (Björck et al. Citation1994; Noy et al. Citation2001; Tako et al. Citation2004). In addition, intestinal villi shortening in height and width had impaired nutrient absorption. Unlike mammals, cell divisions in the birds’ intestine are not limited to crypts, they also occur along the villi (Uni et al. Citation1998). Long villi and shallow crypts are typical intestinal morphometry in birds (Uni et al. Citation1998, Citation2000), although agents such as drugs and/or pathogens can cause alterations in intestinal morphometry (Pluske et al. Citation1997).
4.2. Immunohistochemistry
This study showed that there was minimal ASMA expression at 6 and 14 days within the muscular layers and muscularis mucosa of the small intestinal. At 28 and 52 days, intense expression of ASMA occurred throughout muscularis layers; however the vessel walls showed more intense expression of ASMA in muscularis layers. It has been evidenced that the ‘extra’ actin in arteries is due to a high content of ASMA. This difference is due to the production of the high ratio of alpha to gamma-actin in arteries. The muscles that are usually relaxed (as in the muscular layer of the oesophagus and intestine) consist mainly of γ-actin. In contrast, the muscles that contract are usually (arteries and lower oesophageal sphincter) contain larger amounts of ASMA (Fatigati & Murphy Citation1984). Gamba et al. (Citation2004) showed that there is minimal ASMA expression within the inner circular muscular layer of the small intestinal and muscularis propria.
It seems that the immunoreactivity for ASMA in the inner part of the circular muscle layer may relate to special functional properties during contraction, such as acting as pacemaker cells (Duke Citation1982). Immunoreactivity in the proventriculus muscularis mucosa showed that ASMA was present in all smooth muscle cells except in its outer part (Yamamoto et al. Citation1996). In the birds’ gizzard, other results were obtained about the ASMA cells. As several studies showed, there are large amounts of gamma-smooth muscle actin and β-cytoplasmic actin in smooth muscle cells and there are little amounts of ASMA in electrophoretic findings (Vandekerckhove & Weber Citation1978; Rubenstein Citation1981). In contrast, IHC studies have shown no immunoreactivity for ASMA in the gizzard except for the blood vessels (Skalli et al. Citation1986).
The small intestine peristalsis movement in chicken is neurogenic (Hodgkiss Citation1984), but in the oesophagus and large intestine it is myogenic (Bartlet Citation1973; Mizuta et al. Citation1980; Hodgkiss Citation1984). It was suggested that diversity in the contraction regulation may relate to the individual expression patterns of ASMA in the smooth muscle cells of each region of the avian intestinal tracts. Capacity of stainability in longitudinal muscular layers in this study revealed distinct variations among other muscular layers of the alimentary canal. It seems that longitudinal muscle layers have a dissimilar function during contraction throughout the chicken intestinal tract.
However, as determined by IHC, results of the present study showed that immunoreactivity of ASMA varied in different ages during development in the small intestinal tract and the muscle layers.
Acknowledgments
We thank Sir Hatampour, Dr Baradaran and Miss Naghshineh for immunohistochemistry staining.
Disclosure statement
No potential conflict of interest was reported by the authors.
Additional information
Funding
References
- Aptekmann KP, Artoni SM, Stefanini MA, Orsi, MA. 2001. Morphometric analysis of the intestine of domestic quails (Coturnix coturnix japonica) treated with different levels of dietary calcium. Anat Histol Embryol. 30:277–280.
- Baranyiova E, Holub A, Ponizilova E. 1983. Changes in the mass and chemical composition of the gastrointestinal tract and liver of ducks in the first two months after hatching. Acta Vet Brno. 52:39–47.
- Bartlet A. 1973. Myogenic peristalsis in isolated preparations of chicken oesophagus. Brit J Pharmacol. 48:36–47.
- Berseth CL. 1996. Gastrointestinal motility in the neonate. Clin Perinatol. 23:179–190.
- Björck I, Granfeldt Y, Liljeberg H, Tovar J, Asp N-G. 1994. Food properties affecting the digestion and absorption of carbohydrates. Am J Clin Nutr. 59:699S–705S.
- Duke GE. 1982. Gastrointestinal motility and its regulation. Poultry Sci. 61:1245–1256.
- Dziala SE. 2005. Morphological and morphometric characteristics of the glandnlar stomach and gizzard in the longtail duck (Clangula hyemalis). Zool Pol. 50:49–61.
- Eurell JA, Frappier BL (2013). Dellmann's textbook of veterinary histology. 5th ed. New York: Wiley-Blackwell.
- Fatigati V, Murphy R. 1984. Actin and tropomyosin variants in smooth muscles. dependence on tissue type. J Biol Chem. 259:14383–14388.
- Gamba E, Carr N, Bateman, A. 2004. Deficient α-smooth muscle actin expression as a cause of intestinal pseudo-obstruction: fact or fiction? J Clin Pathol. 57:1168–1171.
- Gracia MI, Lázaro R, Latorre MA, Medel P, Araníbar MJ, Jiménez-Moreno E, Mateos GG. 2009. Influence of enzyme supplementation of diets and cooking–flaking of maize on digestive traits and growth performance of broilers from 1 to 21 days of age. Anim Feed Sci Tech. 150:303–315.
- Guyton AC. 1991. General principles of gastrointestinal function mortality, nervous control, and blood circulation. In: John EH, editor. Textbook of medical physiology. Philadelphia: WB Saunders; p. 71–94.
- Hartshorne DJ. 1987. Biochemistry of the contractile process in smooth muscle. In: Johnson LR, ed. Physiology of the gastrointestinal tract. 2nd ed. New York, NY: Raven Press; p. 423–482.
- Hodgkiss J. 1984. Peristalsis and antiperistalsis in the chicken caecum are myogenic. Quart J Exp Physiol. 69:161–170.
- Hu Z, Guo Y. 2007. Effects of dietary sodium butyrate supplementation on the intestinal morphological structure, absorptive function and gut flora in chickens. Anim Feed Sci Tech. 132:240–249.
- Jackson S. 1992. Do seabird gut sizes and mean retention times reflect adaptation to diet and foraging method? Physiol Zool. 65:674–697.
- Jani PU, McCarthy DE, Florence AT. 1992. Nanosphere and microsphere uptake via Peyer's patches: observation of the rate of uptake in the rat after a single oral dose. Int J Pharmaceut. 86:239–246.
- Kanamori Y, Ishimaru K, Nanno M, Maki K, Ikuta K, Nariuchi H, Ishikawa H. 1996. Identification of novel lymphoid tissues in murine intestinal mucosa where clusters of c-kit + IL-7R+ Thy1+ lympho-hemopoietic progenitors develop. J Exp Med. 184:1449–1459.
- King DE, Asem EK, Adeola O. 2000. Ontogenetic development of intestinal digestive functions in White Pekin ducks. J Nutr. 130:57–62.
- Lefevre M, Joel D, Schidlovsky G. 1985. Retention of ingested latex particles in Peyer's patches of germfree and conventional mice. Proc Soc Exp Biol Med. 179:522–528.
- Lilja C. 1981. Postnatal growth and organ development in the goose (Anser anser). Growth. 45:329–341.
- Lilja C, Sperber I, Marks H. 1985. Postnatal growth and organ development in Japanese quail selected for high growth rate. Growth. 49:51–62.
- Liu BY, Wang ZY, Yang HM, Wang XB, Hu P, Lu J. 2010. Developmental morphology of the small intestine in Yangzhou goslings. African J Biot. 9:7392–7400.
- Maiorka A, Santin E, Dahlke F, Boleli I, Furlan R, Macari M. 2003. Posthatching water and feed deprivation affect the gastrointestinal tract and intestinal mucosa development of broiler chicks. J Appl Poultry Res. 12:483–492.
- Mitjans M, Barniol G, Ferrer R. 1997. Mucosal surface area in chicken small intestine during development. Cell Tissue Res. 290:71–78.
- Mizuta S, Ohashi H, Okado E, Takewaki T. 1980 . A pharmacological study on peristalsis in the isolated rectum of the chicken. Research Bulletin of the Faculty of Agriculture, Gifu University; p. 151–161.
- Noy Y, Geyra A, Sklan D. 2001. The effect of early feeding on growth and small intestinal development in the posthatch poult. Poultry Sci. 80:912–919.
- Pekas JC, Wray JE. 1991. Principal gastrointestinal variables associated with metabolic heat production in pigs: statistical cluster analyses. J Nutr. 121:231–239.
- Pluske JR, Hampson DJ, Williams IH. 1997. Factors influencing the structure and function of the small intestine in the weaned pig: a review. Livest Prod Sci. 51:215–236.
- Przystalski A. 1986. The structure and dimensions of the alimentary canal in postembryonic development of the great tit (Parus major L.). Zool Pol. 33:5–21.
- Rubenstein PA. 1981. Differential behavior of gizzard isoactins. Arch Biochem Biophy. 210:598–608.
- Sell J, Angel C, Piquer F, Mallarino E, Al-Batshan H. 1991. Developmental patterns of selected characteristics of the gastrointestinal tract of young turkeys. Poultry Sci. 70:1200–1205.
- Skalli O, Ropraz P, Trzeciak A, Benzonana G, Gillessen D, Gabbiani G. 1986. A monoclonal antibody against alpha-smooth muscle actin: a new probe for smooth muscle differentiation. J Cell Biol. 103:2787–2796.
- Tako E, Ferket P, Uni Z. 2004. Effects of in ovo feeding of carbohydrates and beta-hydroxy-beta-methylbutyrate on the development of chicken intestine. Poultry Sci. 83:2023–2028.
- Uni Z, Ganot S, Sklan D. 1998. Posthatch development of mucosal function in the broiler small intestine. Poultry Sci. 77:75–82.
- Uni Z, Noy Y, Sklan D. 1999. Posthatch development of small intestinal function in the poult. Poultry Sci. 78:215–222.
- Uni Z, Zaiger G, Gal-Garber O, Pines M, Rozenboim I, Reifen R. 2000. Vitamin A deficiency interferes with proliferation and maturation of cells in the chicken small intestine. Brit Poultry Sci. 41:410–415.
- Vandekerckhove J, Weber K. 1978. At least six different actins are expressed in a higher mammal: an analysis based on the amino acid sequence of the amino-terminal tryptic peptide. J Mol Biol. 126:783–802.
- Wang JX, Peng KM. 2008. Developmental morphology of the small intestine of African ostrich chicks. Poultry Sci. 87:2629–2635.
- Weisbrodt NW. 1987. Motility of the small intestine. Physiol Gastr Tract. 1:631–663.
- Yamamoto Y, Kubota T, Atoji Y, Suzuki Y. 1996. Distribution of alpha-vascular smooth muscle actin in the smooth muscle cells of the gastrointestinal tract of the chicken. J Anat. 189:623–630.