ABSTRACT
This study was carried out to determination of in vitro binding capacity of wheat bran, rice bran, and perlite for Mn, Zn, Cu, and Fe. For this reason, wheat bran and rice bran were chemically analysed for moisture, protein, ash, ether extract, total carbohydrate, neutral detergent fibre, acid detergent fibre, acid detergent lignin, crude fibre, cellulose, hemicelluloses, phytic acid, Non-Fiber Carbohydrate, total dietary fibre, soluble dietary fibre, insoluble dietary fibre, DF + lignin, and endogenous minerals. Mineral binding capacity of wheat bran, rice bran, and perlite for Mn, Zn, Fe, and Cu under sequential simulated physiological conditions of the stomach, small intestine, and colon was investigated and compared. Rice bran showed the highest mineral-binding capacity in small intestine for Mn, Zn, and Cu. Among the minerals, Mn exhibited the lowest percentage of binding under the simulated physiological conditions of the small intestine and the highest percentage of removing occurred in the stomach for all of the treatments. Cereal bran's had an affinity for Cu > Zn > Fe > Mn. The amounts of minerals bound varied among samples. Rice bran showed the highest and perlite had lowest mineral-binding capacity in small intestine for Mn, Zn, and Cu.
1. Introduction
Recommendation for the increase of dietary fibre (DF) intake has raised questions about their possible negative effects on mineral bioavailability (Idouraine et al. Citation1995; Idouraine, Khan, Kohlhepp, et al. Citation1996). Although this issue has raised interest among many scientists for the last two decades, conflicting results from numerous in vitro (Idouraine et al. Citation1995; Luccia & Kunkel Citation2002; Persson et al. Citation1987) and in vivo (Coudray et al. Citation1997, Citation2003; Moak et al. Citation1987; Shah et al. Citation1990) studies have made it difficult to draw a clear conclusion on this controversial subject.
By reviewing the adverse effects of DF on mineral bioavailability in previous literatures (Harland Citation1989; Kelsay Citation1986; Munoz & Harland Citation1993), three postulations on how DF might limit mineral bioavailability have been suggested. They are (a) shortening the transit time of nutrients when DF moves along the small intestine and thus reducing the time required for mineral absorption; (b) directly or indirectly impairing the transportation of minerals when DF move across the intestinal mucosa cells; and (c) electrostatistic binding and/or trapping of minerals within DF particles, leading to the formation of stable, unabsorbable mineral–fibre complexes and thus reducing the pool of ionized minerals for absorption. Because the last population is believed to be the main factor determining the undesirable effect of DF on the mineral bioavailability (Laszlo Citation1989).
When DF is ingested, it generally passes through three major gastrointestinal sections including the stomach, small intestine, and colon (Ji Citation1998). These three gastrointestinal sections possess unique physiological conditions (such as regional pH, ionic strength, and average transit time), which had been found to significantly affect the in vitro mineral-binding capacity of DF in numerous previous studies (Camire & Clydesdale Citation1981; Elhardallou & Walker Citation1999; Laszlo Citation1989; Platt & Clydesdale Citation1987). Neutral detergent fibre (NDF) consists of cellulose, lignin, and hemicellulose and is often called cell walls. Because NDF represents the total fibre in a feed. Acid detergent fibre (ADF) consists of cellulose, lignin, lignified nitrogen compounds (heat-damaged protein), and insoluble ash. ADF does not represent the total fibre content in feed, as it does not account for hemicellulose. It is a relatively quick method for measuring fibre, often substituting for CF.
Non-fibre carbohydrate is made up of different amounts of starch, simple sugars, betaglucans, galactans, and pectins (soluble fibre) that make up the highly digestible carbohydrate fraction in feeds. Non-Fiber Carbohydrate (NFC) is calculated by the difference [100 – (%NDF + %CP + %fat + ash)]. The term non-structural carbohydrate is often used interchangeably with NFC but is analytically determined and may be slightly different from Non-Fiber Carbohydrate (NFC). The aim of the study was to determine how DF has the ability to bind cations in vitro. However, many components in the diet and the physiological conditions like colonic bacteria in the gastrointestinal tract will potentially interfere with these binding characteristic.
2. Material and methods
2.1. Materials
Organic sources of whole fibres were wheat and rice bran (local produce) and perlite (local source) was the inorganic source in this study.
2.2. Analytical methods
The moisture content of the samples was determined by heating circa 1 g in an oven, provided with a fan, for 16 h at 105 ± 2°C and 2 h at 130 ± 2°C. The ash content was determined in a muffle furnace by slowly heating 0.5–2.0 g samples (in porcelain crucibles) up to 500°C for 16 h. Their protein content was estimated from the quantitative analysis of nitrogen using the Kjeldahl method (N × 6.25) (Egan et al. Citation1981). It was determined by a KjeltecTM (Foss, 8400). NDF, ADF, acid detergent lignin (ADL), and crude fibre were determined according to the Van Soest (Citation1963) method using FibertecTM (Foss, 2010). Crude fat was determined according to AOAC (Citation1990). Phytic acid content was determined according to De Boland et al. (Citation1975). Also, DF contents of wheat and rice bran were determined by using Megazyme assay kits (Megazyme International Ireland Ltd, Wicklow, Ireland) according to Approved Methods of the American Association of Cereal Chemists (AACC Citation2000).
2.3. In vitro mineral-binding capacity
The in vitro mineral-binding capacity of the wheat bran, rice bran, and perlite on Cu, Zn, Fe, and Mn was investigated under sequential simulated physiological conditions of the gastrointestinal tract according to the modified method of Idouraine, Khan, and Weber (Citation1996). About 25 g of each sample was gently shaken in a 1% HCl solution (pH: 1.5; ionic strength: 75 mM KCl; 1:20 w/v) in a 600 mL beaker for a period of 1 h at 37°C in order to assess its mineral-binding capacity in the stomach. The slurry was then filtered through a Whatman 541 ashless filter paper, and the residue was washed several times with ultrapure water until the filtrate tested neutral (pH 7) (samples treated under gastric conditions). All samples treated under gastric conditions were then freeze-dried, and those samples were kept for analysis of minerals. For determining the mineral-binding capacity under simulated physiological conditions of the small intestine, 4 g of each sample was separately mixed in 40 mL of mineral solution (1000 mg/L containing 39% Mn (MnSO4·H2O), 38% Zn (ZnSO4·7H2O), 19% Fe (FeSO4·7H2O), and 4% Cu (CuSO4·5H2O). Then the volume of the mixture was made up to 400 mL by adding 2.0 mM MES (Merck, Germany) buffer solution (pH 6.8; ionic strength=100 mMKCl) and incubated at 37°C for 3 h with moderate agitation. The slurry was then centrifuged (Hettich Zentrifugen D-78532) at 2500g for 15 min under room temperature. The supernatant was discarded, and the residue was washed several times with ultrapure water (sample treated under small intestine conditions). Subsequently, each sample treated under small intestinal conditions was then freeze-dried, and those samples were retained for mineral analysis. Finally, to assess the mineral-binding capacity under a mimicked physiological conditions of the colon with slightly acidic pH, portions of the mineral-bound samples (2 g) treated with simulated small intestinal physiological conditions were further incubated with 2.0 mM MES buffer solution (pH 5.8; ionic strength = 100 mMKCl; 1:100, w/v) in a 250 mL Erlenmeyer flask for 3 h at 37°C. The resulting mixture was centrifuged at room temperature (2500g, 15 min), and the residue was washed several times with ultrapure water until the pH value was approximately 7 (samples treated under colonic conditions). All samples treated under colonic conditions were then freeze-dried, and those samples were retained for mineral analysis.
2.4. Mineral content determination
About 0.5 g of the original (endogenous) samples treated under gastric conditions, small intestinal conditions, and colonic conditions were wet-ashed sequentially with nitric acid (65%) and hydrogen peroxide (30%) followed by HCl. Excess HNO3 was evaporated on a hot plate set at 100–120°C. The Mn, Zn, Fe, and Cu concentrations in the ashed solution were then analysed by using a flame atomic absorption spectroscopy as described by AOAC (Citation1990) (Spectro AA, VARIAN).
2.5. Statistical analysis
A total of three samples for each treatment were used for the present report. SPSS10 was used for statistical analysis. Experimental data, expressed as mean ± SD, were analysed by one way ANOVA. The differences between means were separated by Duncan's multiple range tests. Significant differences were declared at p < .05.
3. Results
Chemical composition as moisture, protein, ash, ether extract, and total carbohydrate content of the wheat and rice bran are presented in . Other way, amount of NDF, ADF, ADL, phytic acid, and crude fibre of the wheat and rice bran are shown in . Thus, polysaccharide in the cell wall as hemicelluloses, cellulose, total dietary fibre, soluble dietary fibre, insoluble dietary fibre, and DF + lignin content of wheat and rice bran are presented in .
Table 1. Proximate analysis of wheat bran and rice bran (%mean ± SD).
Table 2. NDF, ADF, ADL, and phytic acid of wheat bran and rice bran (%mean ± SD).
Table 3. Cellulose and hemicellulose of wheat bran and rice bran (%mean ± SD).
The endogenous Mn, Zn, Cu, and Fe contents of wheat bran, rice bran, and perlite are presented in . The endogenous mineral contents greatly varied among the three samples. Under the simulated physiological conditions of the stomach, the strong acidic environment removed most of the endogenous Mn from wheat bran, rice bran, and perlite (72.08–91.42% removal).
Table 4. Endogenous mineral content and in vitro mineral-binding capacity of the samples under sequential simulated physiological conditions of the stomach (% removal), small intestine (% binding), and colon (% releasing) (mean ± SD).
The mineral-binding behaviour of the samples treated under the three gastric conditions under the simulated physiological conditions of the small intestine is also shown in . Among the samples treated under the three gastric conditions, rice bran exhibited the highest % binding for all of the minerals studied except Fe. Perlite showed the lowest binding capacity while rice bran the highest for Mn, Zn, and Cu. Organic sources (wheat bran and rice bran) seem to bind more Mn, Zn, and Cu than perlite.
In , the column titled ‘% binding’ shows that the cereal brans had an affinity for Cu > Zn > Fe > Mn and inorganic source (perlite) had an affinity for Fe > Zn > Cu > Mn. Among the minerals, Mn exhibited the lowest % binding under the simulated physiological conditions of the small intestine and the highest % removal under the simulated physiological conditions of the stomach for cereal brans.
Table 5. Endogenous mineral content and in vitro mineral-binding capacity of the samples under sequential simulated physiological conditions of the stomach (% removal), small intestine (% binding), and colon (% releasing).
Perlite showed the lowest binding capacity while rice bran the highest for Mn, Zn, and Cu. Organic sources (wheat bran and rice bran) seem to bind more Mn, Zn, and Cu than perlite ().
4. Discussion
Among the samples treated under the three gastric conditions, rice bran exhibited the highest % binding for all of the minerals studied except Fe. These results showed that perlite might contain the highest number of specific sites for Fe or it might have the same number of sites but with stronger affinity for Fe than that of the other two treatments. Rice bran seems to bind more Fe than wheat bran.
Under the simulated physiological conditions of the stomach, the strong acidic environment removed most of the endogenous minerals from perlite (Mn, 91.42% removal; Zn, 94.30% removal; Cu, 86.99% removal) with the exception of Fe (only 35.73% removal). According to Laszlo (Citation1989) as well as Thompson and Weber (Citation1981), the ineffective release of endogenous Fe under gastric condition might suggest that the binding mechanism of this mineral might only be partial via electrostatistic interactions.
Binding of iron to cellulose has been observed to be minimal under any pH. After reviewing the literature on Fe-cellulose binding, Clydesdale (Citation1983) found that a very weak bond was formed between the mineral and the DF component. Southgate (Citation1987) reported that there is no evidence of bonding between iron and cellulose and suggests that some Fe is adsorbed to the surface of cellulose.
DF is believed to have a negative impact on mineral bioavailability. A similar result had been observed previously (Caprez & Fairweather-Tait Citation1982; Davies & Nightingale Citation1975; McKenzie & Davies Citation1981).
The high mineral-binding capacity of rice bran could be related to the higher ADF, lignin, phytic acid, cellulose, and CF of this cereal bran compared to wheat bran and perlite ().
Figure 2. Comparison of some components and mineral-binding capacity in wheat bran, rice bran, and perlite.
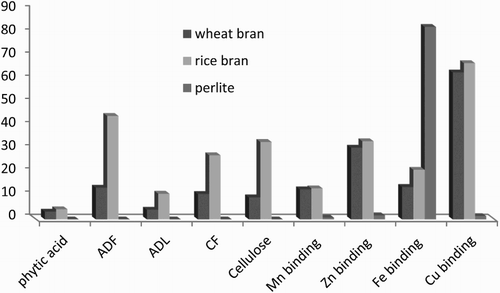
Cellulose is a insoluble linear unbranched polymer of β-(1,4)-d-glucose residues associated with other cellulose chains by hydrogen bonding and Van der Waals forces. Cellulose chains aggregate together to form microfibrils, which are highly crystalline and insoluble structures, each one about 3 nm in diameter. Cellulosemicrofibrils comprise the core of the plant cell wall; one-third of the total mass of wall is cellulose. Themicrofibrilar disposition allows the existence of micro spaces between the microfibrils that are fulfilled by matricial polysaccharides according to the age and tissue type. Minerals can be trapped in the cell wall matrix. Rice bran has cellulose more than wheat bran ().
Phytate (myo-inositol 1, 2, 3, 4, 5, 6-hexakis dihydrogen phosphate) is an anionic acid with anti-nutritional properties. Phytate has been shown to form complexes with trace minerals and decreases their availability (Davies & Nightingale Citation1975; Erdman Citation1979; Maddaiah et al. Citation1964; Ravindran et al. Citation1995; Vohra et al. Citation1965).
It has been shown that cations bind to DF in vitro (Camire & Clydesdale Citation1981). Fibres from different plant sources have been shown to have different chemical structures, and thus different binding capacities (Slavin Citation1987; Van Soest & Jones Citation1988). It is difficult to draw definite conclusions regarding the relation between the composition of studied products, and mineral binding, because plant foods are usually associated with substances which may also bind minerals. Moreover, the fibre content and composition of these plant foods differ among studies, depending on the analytical methods used to measure DF content (Torre et al. Citation1991).
Although DF from plant material or isolated components has been shown to bind essential inorganic nutrients, the mechanisms involved are still being investigated.
5. Conclusion
Mn, Zn, Cu, and Fe content varied significantly among the samples of wheat bran, rice bran, and perlite. The amounts of Mn, Zn, Cu, and Fe bound to these sources do not appear to be correlated only to the parameters that were determined in this study. This is probably due to the variation in chemical composition and chemical structures of these sources when considered as a whole. Other components or more complex mechanisms might also be involved. Further work is needed on the total binding of different sources for minerals not only to understand the mechanism involved but also to predict the degree of binding of a given fibre for a given mineral, which could be useful for consumers.
Disclosure statement
No potential conflict of interest was reported by the authors.
References
- AACC. 2000. Approved methods of the American association of cereal chemists. 10th ed. St. Paul (MN): American Association of Cereal Chemists.
- [AOAC] Association of Official Analytical Chemists. 1990. Official methods of analysis. Washington, DC: American Association of Cereal Chemists. 14th ed., 1st Suppl., Secs. 43, A14–43, A20; p. 399.
- Camire AL, Clydesdale FM. 1981. Effect of pH and heat treatment on the binding of calcium, magnesium, zinc and iron to wheat bran and fractions of dietary fiber. J Food Sci. 46: 548–551. doi: 10.1111/j.1365-2621.1981.tb04907.x
- Caprez A, Fairweather-Tait SJ. 1982. The effect of heat treatment and particle size of bran on mineral absorption in rats. Br J Nutr. 48: 467–475.
- Clydesdale FM. 1983. Physicochemical determinants of iron bioavailability. Food Technol. 37:133–138.
- Coudray C, Bellanger J, Castiglia-Delavaud C, Remesy C, Vermorel M, Rayssignuir Y. 1997. Effect of soluble or partly soluble dietary fibers supplementation on absorption and balance of calcium, magnesium, iron and zinc in healthy young men. Eur J Clin Nutr. 51: 375–380. doi: 10.1038/sj.ejcn.1600417
- Coudray C, Demigne C, Rayssiguier, Y. 2003. Effects of dietary fiber on magnesium absorption in animals and humans. J Nutr. 133:1–4.
- Davies NT, Nightingale R. 1975. The effects of phytate on intestinal absorption and secretion of zinc, and whole-body retention of Zn, copper, iron and manganese in rats. Br J Nutr. 34:243–258. doi: 10.1017/S0007114575000293
- De Boland AR, Garner GB, O Dell BL. 1975. Identification and properties of phytate in cereal grains and oil seed products. J Agr Food Chem. 23:1186–1189. doi: 10.1021/jf60202a038
- Egan H, Kirt RS, Sawyer R. 1981. General chemical methods. In Pearson's chemical analysis of foods. 8th ed. Edinburgh: Churchill Livingston; p. 7–34.
- Elhardallou SB, Walker AF. 1999. The effect of multi-mineral mix (Fe, Zn, Ca and Cu) on magnesium binding to starchy legumes under simulated gastrointestinal conditions. Food Chem. 67:113–121. doi: 10.1016/S0308-8146(99)00059-X
- Erdman Jr. JW, 1979. Oilseed phytates: nutritional implications. J Am Oil Chem Soc. 56:736–741. doi: 10.1007/BF02663052
- Harland BF. 1989. Dietary fiber and mineral bioavailability. Nutr Res Rev. 2:133–147. doi: 10.1079/NRR19890011
- Idouraine A, Hassani BZ, Claye SS, Weber CW. 1995. In vitro mineral binding capacity of various fiber sources magnesium, zinc and copper. J Agr Food Chem. 43:1580–1584. doi: 10.1021/jf00054a031
- Idouraine A, Khan MJ, Kohlhepp EA, Weber CW. 1996. In vitro mineral binding capacity of three fiber sources for Ca, Mg, Cu, and Zn by two different methods. Int J Food Sci Nutr. 47:285–293. doi: 10.3109/09637489609041027
- Idouraine A, Khan MJ, Weber CW. 1996. In vitro binding capacity of wheat bran, rice bran and oat fiber for Ca, Mg, Cu and Zn alone and in different combinations. J Agr Food Chem. 44:2067–2072. doi: 10.1021/jf960151e
- Ji SK, Biomineral. 1998. Absorptive process of mineral nutrients through small intestine membrane. Seoul: K.I.P; p. 43–61.
- Kelsay JL. 1986. Update on fiber and mineral availability. In: Vahouny G, Kritchevsky D, editors. Dietary fibers, basic and clinical aspects. New York: Plenum Press; p. 361–372.
- Laszlo JA. 1989. Effect of gastrointestinal conditions on the mineral binding properties of dietary fibers. In: Dintzis FR, Laszlo JA, editors. Mineral absorption in the monogastric GI tract. New York: Plenum Press; p. 133–145.
- Luccia BHD, Kunkel ME. 2002. In vitro availability of calcium from sources of cellulose, methylcellulose, and psyllium. Food Chem. 77:139–146. doi: 10.1016/S0308-8146(01)00168-6
- Maddaiah VT, Kurnick AA, Reid BL. 1964. Phytic acid studies. Proc Soc Exp Biol Med. 115:391–393. doi: 10.3181/00379727-115-28922
- McKenzie JM, Davies NT. 1981. Influence of dietary protein on zinc availability from bread in rats. In: McC. Howell J, Gawthorne JM and White CL, editors. Trace element metabolism in man and animals-4. Canberra: Australian Academy of science; p. 111.
- Moak S, Pearson N, Shin K. 1987. The effect of oat and wheat bran fibers on mineral metabolism in adult males. Nutr Rep Int. 36:1137–1146.
- Munoz JM, Harland BF. 1993. Overview of the effect of dietary fiber on the utilization of minerals and trace elements. In: Spiller GA, editor. CRD handbook of dietary fiber in human nutrition. Boca Raton (FL): CRC Press; p. 245–252.
- Persson H, Nair BM, Frolich W, Nyman M, Asp N. 1987. Binding of mineral elements by some dietary fiber components in vitro (II). Food Chem. 26:139–148. doi: 10.1016/0308-8146(87)90124-5
- Platt SR, Clydesdale FM. 1987. Mineral binding characteristics of lignin, guar gum, cellulose, pectin and neutral detergent fiber under simulated duodenal pH conditions. J Food Sci. 52:1414–1419. doi: 10.1111/j.1365-2621.1987.tb14096.x
- Ravindran V, Bryden WL, and Kornegay ET. 1995. Phytates: occurrence, bioavailability and implications in poultry nutrition. Poult Avian Biol Rev. 6:125–143.
- Shah BG, Malcom S, Belonje B, Trick KD, Brassard R, Monge AR. 1990. Effect of dietary cereal brans on the metabolism of calcium, phosphorus and magnesium, in a long-term rat study. Nutr Res. 10:1015–1028. doi: 10.1016/S0271-5317(05)80043-0
- Slavin JL. 1987. Dietary fiber: classification, chemical analyses and food sources. J Am Diet Assoc. 87:1164–1171.
- Southgate DAT. 1987. Minerals, trace elements and potential hazards. Am J Clin Nutr. 45:1256–1266.
- Thompson SA, Weber CW. 1981. Copper and zinc binding to dietary fiber sources: an ion exchange column method. J Food Sci. 47:125–126. doi: 10.1111/j.1365-2621.1982.tb11042.x
- Torre M, Rodriguez AR, Saura-Calixto F. 1991. Effects of dietary fiber and phytic acid on mineral availability. Crit Rev Food Sci Nutr. 1:1–22. doi: 10.1080/10408399109527539
- Van Soest PJ. 1963. Use of detergents in the analysis of fibrous feeds. II. A rapid method for the determination of fiber and lignin. J Assoc Off Anal Chem. 50:50–55.
- Van Soest PJ, Jones LHP. 1988. Analysis and classification of dietary fiber. In: Bratter P, Schramel P, editors. Trace element analytical chemistry in medicine and biology. New York: Walter de Gruyter; p. 351–352.
- Vohra P, Gray GA, Kratzer FH. 1965. Phytic acid-metal complexes. Proc Soc Exp Biol Med. 120:447–449. doi: 10.3181/00379727-120-30559