ABSTRACT
The nickel impacts on antioxidant enzymes’ activities such as superoxide dismutase (SOD), glutathione peroxidase (GPx), catalase (CAT) and hepatic enzymes’ activity were studied in freshwater carassius auratus. Probite analysis was used to determine acute toxicity of nickel to the goldfish. Fish were subjected to selected concentrations of nickel (control 0, 10, 20, 50, 100, 150, 200, 250 and 300 ppm) and the mortality data were determined after 24, 48, 72 and 96 h. The 96 h LC50 value of nickel to the fish was found to be 95.39 ± 0.73 ppm. SOD, GPx and CAT activities were monitored in the liver. These were determined in goldfish exposed to sub-lethal concentrations (40% and 80% LC50 at 7 days of exposure). There was a marked increase in SOD within both exposure groups and GPx was observed in this tissue having exposure to nickel (P < .05) but CAT was decreased in both the groups (P < .05). AST, ALP, ALT enzymes’ activity in both groups (40% and 80% LC50) was increased significantly when compared to that of the control group (P < .05). This result implied that increasing the dosage of nickel exposure induced antioxidant and hepatic enzymes’ activity in the goldfish.
1. Introduction
Heavy metals’ chronic contamination in the marine environment is found to be an adverse issue, especially in water bodies. Particularly, the aquatic medium is affected by wide varieties of heavy metals deposited into it. Enormous industrial and agricultural processes have been implicated in the contamination of fresh water systems, thereby resulting in adverse impacts on both aquatic biota and human health (Wang Citation2002; Dautremepuits et al. Citation2004). Nickel that enters the environment from natural or anthropogenic sources is dispersed through the systems by chemical and physical processes and is embodied in the food chains through uptake into living organisms (Eisler Citation1998). Nickel is frequently widely utilized in industry and serves as a common aquatic contaminant. Nickel is an important chemical species in natural waters. In aquatic ecosystems, it interacts with many mineral and organic compounds and occurs as soluble salts adsorbed onto materials belonging to various chemical origins (Us epa Citation1980). Many of such pathways are additive or synergistic in causing adverse effects, and some are antagonistic as well. Nickel’s toxicity to aquatic life has been dealt with intensively during previous decades, and a considerable amount of experimental data has been accumulated and reviewed (Atli & Canli Citation2007). Fish inhabiting polluted areas are frequently exposed to toxic compounds, many of them occurring due to cytotoxic impacts by the production of reactive oxygen species (ROS; Di Giulio et al. Citation1989). Most biomolecules, namely lipids, proteins and DNA, are affected by ROS that inflict damage (Winston & Di Giulio Citation1991). To turn ROS into harmless metabolites as well as to conserve and recover normal cellular metabolism and functions endogenous enzymatic and non-enzymatic antioxidants are essential (Bebe & Panemangalore Citation2003). Fish are particularly susceptible to water pollution and pollutants may interrupt many physiological and biochemical pathways in case assimilated by fish tissue among many others (Durmaz et al. Citation2006). Once ROS generation caused by xenobiotics exceeds the thresholds, impairments to cellular components occur. This process is called oxidative stress (Oakes & Van der Kraak Citation2003). The antioxidant defence system involves enzymes such as superoxide dismutase (SOD), glutathione peroxidase (GPx), catalase (CAT), glutathione S-transferase (GST) and other low-molecular-weight scavengers such as reduced glutathione (GSH) (Storey Citation1996; Droge Citation2002). In the present study, the sub-lethal toxicities due to nickel exposure were studied in goldfish as an in vivo model by the measurement of hepatic enzymes’ activity and oxidative damages. Oxidative activities were measured in carassius auratusas as biomarkers of environmental contamination (Van der Oost et al. Citation2003).
2. Materials and methods
2.1. Experimental animals and condition
Goldfish (with weight mean of 14 ± 0.5 g and length mean of 6 ± 0.8 cm) obtained from commercial fish farms, Gorgan, Iran, were used as samples for acute toxicity tests. Only healthy fish, as shown in terms of their activity and external appearance, were kept alive within a fibreglass tank. Samples were transferred to a 400 L aerated tank of which 200 L were considered as the test medium. All samples were acclimated for 1 week in 15 aerated fibreglass tanks at 19 ± 1°C under a constant 12:12 L:D photo-period. Acclimatized fish were daily fed a formulated feed. Dead fish were immediately removed with special plastic forceps to avoid possible deterioration of water quality (Shaluei et al. Citation2012).
2.2. Acute toxicity test
The fish were exposed to the selected concentrations of nickel (control 0, 10, 20, 50, 100, 150, 200, 250 and 300 ppm) and the mortality data were collected after 24, 48, 72 and 96 h. Every 24 h the mortality count was monitored and dead fish were removed to avoid possible deterioration of the water quality (Gooley et al. Citation2000). Acute median lethal concentration (LC50) and their 95% confidence interval for all tests as per Finney’s method were calculated according to the formula developed by Mohapatra and Rengarajan (Citation1995). LC50 values of 24, 48, 72 and 96 h were determined using Finney’s (Citation1971) method, called ‘probit analysis’ and software SPSS. The average lethal concentration LC50 (concentration of nickel at which 50% mortality of the target population occurred) for an exposure period of 24, 48, 72 and 96 h was designated.
2.3. Sub-chronic test
Two concentrations (40% and 80% of 96-h LC50) were selected for the assessment of the antioxidant and hepatic enzyme changes caused by nickel in fish. In this phase, number of 120 randomly selected Carasiuss auratus from acclimation tanks and randomly phased into several experimental 400-L tanks subject to concentrations 40% and 80% of 96-h LC50 for a period of 7 days to antioxidant and hepatic enzymes activity were changed. In order to carry out a sub-acute toxicity assay, the exposure water in the tank was changed daily and fresh water was added to keep the nickel concentration at a stable level. Furthermore, during the experiment, water was continuously controlled. Under similar circumstances, a control test in the absence of nickel was conducted.
2.4. Tissue samples
Fish that belonged to both experimental and control groups were slaughtered at the end of day 7 by spinal cord transection. The livers were carefully excised and immersed in cold physiological saline (0.9% NaCl). Then immediately samples were transferred into liquid nitrogen. Frozen samples were stored at −80C until the subsequent biochemical determinations were carried out.
2.5. Antioxidant capacities
Spectrophotometry was utilized to measure all enzyme activities at 25°C. Frozen tissue specimens were immediately weighed and homogenized at 18,000 rpm within a Turratec TE 102 (parsazmoon, Iran). In a 0.1 M Na+/K+ phosphate buffer, pH 7.0, at a ratio of 1:5 w/v, tissues got homogenized. Samples were subsequently centrifuged at 12,000 rpm for 30 min at 4°C and to SOD, CAT and GPx activity assays supernatants were used.
A Diagnostic Reagent Kit purchased from parsazmoon Bioengineering Institute (parsazmoon, Iran) was utilized according to the manufacturer’s instructions to assess SOD activities. As per this method, the superoxidase that appeared in the xanthine/xanthine-oxidase superoxide generation system inhibited the formation of nitrite from hydroxylammoniumchloride (Ji et al. Citation1991). The reaction mixture included a phosphate buffer (pH 7.8), xanthine oxidase, SOD enzyme supernatants, xanthine solution and aqueous hydroxylammonium chloride. One unit (U) from enzyme activity is defined as the amount of enzyme yielding 50% inhibition of nitrite formation in 1 ml reaction mixture.
The CAT level in the sampled tissues was specified using the same Diagnostic Reagent Kit. The procedure is inspired by that of Claiborne (Citation1985). CAT activity was calculated in respect to nmol H2O2 consumed/s/g protein.
To estimate GPx activities the decrease in absorbance at 340 nm (6.22/mM/cm) due to NADPH oxidation was considered. This was followed by 50 mMTris-phosphate buffer (pH 7.6) containing 0.1 mM EDTA, 2.4 mM GSH, 1 unit glutathione reductase, 0.16 mM NADPH and 2.4 mMcumenehydroperoxide. One unit of GPx activity was determined as 1 nmol NADPH oxidized/min/mg of protein.
2.6. Hepatic enzymes
As for the biochemical tests, the blood was poured in tubes and allowed to clot under room temperature (22°C) for 30 min. After centrifugation at 1000 rpm serum was removed from the clotted sample after 5 min and then frozen at −80°C until analysis. The level of aspartate aminotransferase (AST) enzyme was determined using the Pars-Azmoon Diagnostics Infinity AST reagent kit according to the method of Reitman and Frankel (Citation1957). The oxaloacetate formed in the reaction was coupled with 2, 4-dinitrophenyl hydrazine (DNPH) to give the corresponding hydrazone, which in turn exhibited a brown colour in alkaline medium, measured colorimetrically at 340 nm and 37°C. A standard curve was plotted using different amounts of pyruvate, and the enzyme activity was expressed as U/L to determine level of alanine aminotransferase (ALT) enzyme Sigma Diagnostics Infinity ALT reagent kit (Homburg, Germany) characterized with DNPH as colour reagent by enzymatic methods described by method of Reitman and Frankel (Citation1957) was used. Results were expressed as units per gram of protein. Coupled with 2, 4- DNPH, pyruvate formed in the reaction yielded the corresponding hydrazone, which in turn appeared brown in colour in alkaline medium, which is measured colorimetrically at 340 nm and 37°C. A standard curve was plotted using different pyruvate values, and the enzyme activity was expressed as U/L. At the same time, alkaline phosphatase level (ALP) was determined by the enzymatic method using the DarmanKave kit (Tehran, Iran) at 37°C and 410 nm.
2.7. Statistical analysis
For normality purposes, data were transformed, and significant differences between responses of exposed groups and non-exposed controls were assessed using a one-way analysis of variance at a significance level of P < .05.
3. Results and discussion
The present research aims to investigate the responses of antioxidants and serum biochemical parameters such as enzymes (ALT, AST, ALP) in goldfish under nickel sub-acute concentrations (40% and 80% LC50) for 7 days. Exposure to a sub-lethal concentration of nickel cyanide led to drastic variations in the biochemical parameters of the fish C. auratus. As a whole, the edible freshwater fish are perceived as one of the major sources of nutritious staple food for humans. The biochemical variations due to nickel cyanide stress may be attributed to interrupted metabolisms manifesting as enzymes’ suppression.
The results from the acute toxicity test as to the effect of nickel on the goldfish are shown in . There was no mortality in the control group throughout the experiment. Fish mortality was increased significantly when the concentrations and the time of exposure were increased. As it was expected, the 96-h LC50 values were decreased with prolonged exposure time. This denotes increase in toxicity with increase in exposure duration. Before death, fish showed rapid gill movement, nervous movements, erratic swimming, imbalance and inability to remain upright. The 96 h LC50 value of nickel to the fish was found to be 95.39 ± 0.73 ppm.
Table 1. Lethal concentrations (LC1–99) of nickel (mean ± standard error) depending on time (24–96 h) for goldfish@@@.
3.1. Antioxidant enzyme activities
The liver antioxidant enzyme activities can be seen in . Those fish subjected to sub-acute concentrations exhibited higher SOD and GPx activities in the liver than the control ones; however CAT enzyme activity was significantly lowered (P < .05). In GPx and CAT, among treatments at 40 and 80% LC50, no significant difference was found; however, in SOD, at both 40 and 80% LC50, a significant difference was observed (P < .05).
Figure 1. The activities of SOD, CAT and GPx in the liver of Carassius auratus with exposure to nickel sub-acute concentrations for 7 days. Values are mean ± SD of 10 fish. The significance level is p < .05.
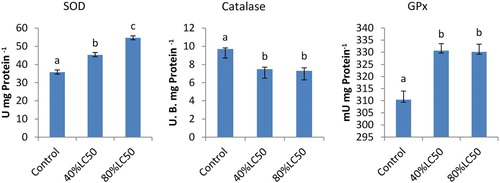
Not only are tissue antioxidant enzymes essential for optimal fish growth and development, but they also make significant contributions to the body immune system. Continuous production of ROS is seen under normal physiological circumstances, which induces oxidative stress to cause damage to cell membranes, inactivation of enzymes as well as damage to genetic material and other vital cell components. Some tissues’ peroxidation, in particular ROS- and free radicals-induced antioxidant, is observed as being highly deleterious, resulting in damage to cellular bio membranes (Mourente et al. Citation1999). Peroxidation induced by oxygen radicals in fish is the main determinant factor in causing several diseases including jaundice (Sakai et al. Citation1989), nutritional muscular dystrophy (Murai & Andrews Citation1974) and hemolysis (Kawatsu Citation1969).
Seven days post nickel treatment, liver SOD activity in goldfish was increased, suggesting that nickel triggers SOD activity. SOD great deal of contributions in defence against the toxic effects of ROS, in turn might scavenge O−2 to protect cells against lesions and strike the equilibrium between oxidant and antioxidant. SOD, GPx and CAT as oxygen-free radical (OFR) enzymatic scavengers enable the system to cope with the adverse effects of OFRs (Khrer Citation1993). Free radicals play a fundamental role in the toxicity of environmental chemicals. Some pesticides may lead to oxidative stress, resulting in generation of free radicals and antioxidants changes or the OFR scavenging enzyme system (Banerjee et al. Citation1999). In other research, SOD activities in the Boleophthalmus pectinirostris liver were substantially triggered after the exposure to 30 mg/L benzo(a)pyrene for 7 days (Feng et al. Citation2000). These research results illustrated that as a sensitive marker, SOD may be used to indicate the biochemical response on organisms. The increased SOD activity denotes a higher intracellular H2O2 formation which can be decomposed by the GPx activity. Antioxidant enzymes such as GPx, SOD and CAT are activated to counteract the negative effect of the ROS (Parvez & Raisuddin Citation2005). Nickel caused increase in GPx activity of goldfish which was recorded at 7 days following nickel treatment (40% and 80% LC50). The GPx function may be induced by xenobiotics, and detoxification of peroxides among many others (Hamed et al. Citation1999).
To protect the biological system against ROS, CAT is an inducible cytosolic enzyme characterized with important functions. Exposed by environmental contamination involving many complicated processes can be evaluated by antioxidant enzyme activity as well as by lipid peroxidation measures. Nevertheless, various CAT responses have been dealt with in fish exposed to sub-acute nickel concentration contaminants under laboratory experiments. Di Giulio et al. (Citation1993) reported a simultaneous increase in CAT activity and lipid peroxidation with laboratory exposures of channel catfish to polluted harbour sediments. Comparing fish Nile tilapia from a fish farm and from a polluted site, Bainy et al. (Citation1996) indicated that the antioxidant defences in the kidney followed a similar pattern to the liver and the gill. They particularly noted a decline in CAT activity attributed to an increase in SOD and O2 (superoxide anion) production by the pollutants. CAT inhibition by O2 was previously reported by Kono and Fridovich (Citation1982). While study on cadmium poisoning in killifish, Fundulus heteroclitus Pruell and Engelhardt (Citation1980) pointed out that CAT activity was inhibited upon exposure to dissolved cadmium under both in vivo and in vitro conditions.
3.2. Hepatic enzymes
As expected, toxicity of nickel increased hepatic enzymes’ activity levels in a significant way (p < .05). The present research showed the increased levels of AST, ALT and ALP in the serum of goldfish once treated with sub-acute nickel (–).
Figure 2. The activities of the Aspartate aminotransferase (AST) enzyme in the plasma of Carassius auratus with exposure to nickel sub-acute concentrations for 7 days. Values are mean ± SD of 10 fish. The significance level is p < .05.
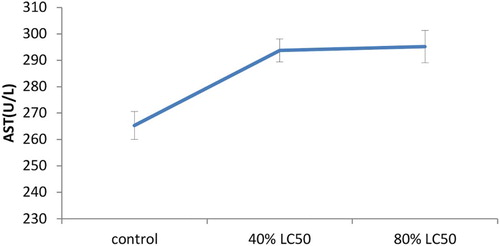
Figure 3. The activities of the alkaline phosphatase (ALP) enzyme in the plasma of Carassius auratus with exposure to nickel sub-acute concentrations for 7 days. Values are mean ± SD of 10 fish. The significance level is p < .05.
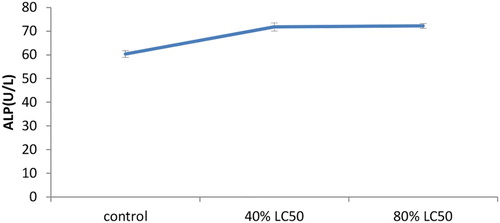
Figure 4. The activities of the Alanine aminotransferase (ALT) enzyme in the plasma of Carassius auratus with exposure to nickel sub-acute concentrations for 7 days. Values are mean ± SD of 10 fish. The significance level is p < .05.
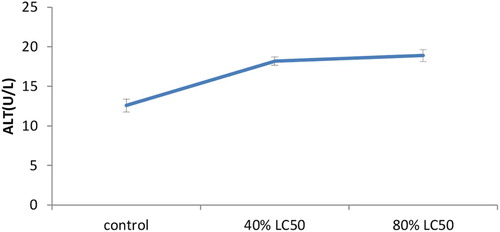
A significant change with 40% and 80% exposures after 7 days was observed in alkaline phosphatase activity. A polyfunctional enzyme called alkaline phosphatase acts as a transphosphorylase at alkaline pH and participates in mineralization of the aquatic fauna skeleton and in membrane transport activities (Bernet et al. Citation2001). There was an increase in ALP activity in the serum and gill in Cyprinus carpio upon exposing to copper (Karan et al. Citation1998). Also in a previous study, an increase in ALP activity was shown in serum O. niloticus after 14 days of 5, 10 and 20 M Zn exposures (Atli & Canli Citation2007). It is apparent that there is an inhibition trend in low Cu exposures, while an increase occurs in higher exposure concentrations presumably due to the structural drastic variations in cells (Karan et al. Citation1998). Changes in ALP activity also could be a result of physiological and functional alterations in metal-exposed fish (Jiraungkoorskul et al. Citation2003).
In nickel-exposed fish aspartate transaminase and ALT activity was increased considerably. The control values for ALT and AST activities in serum were estimated to be 12.6 ± 0.81 and 265.3 ± 5.31 U/L, respectively. In 80% LC50 nickel-exposed fish the highest increase in ALT activity was recorded (18.9 ± 0.74; ); additionally the highest AST activity was measured in 80% LC50 nickel-exposed fish as well (295.2 ± 6.15U/L; ). Like AST and ALT transaminases have a substantial role in protein and amino acid metabolism and may release into the plasma upon tissue damage and dysfunction. Singh and Reddy (Singh and Reddy Citation1990) proved that 0.25 mg Cu/L caused substantial increases in serum AST and ALT activities in Heteropneustes fossilis with increases in exposure time. They attributed the increase to tissue damage and impairment of fish metabolism. Oluah (Citation1999) recorded increased AST activity in Clariaalbo punctatus exposed to Zn and Hg varying from 50 to 0.15 mg/L for 21 days. This increase in AST activity can be attributed to imbalances in the physiology and anatomy of the reservoir tissues. Zikic et al. (Citation2001) proved that plasma AST and ALT activities were increased in Cd-exposed fish Carassius auratus gibelio. The authors indicated that releasing these transaminases into the circulation might occur due to damage of the liver, kidney, heart and other tissues under stress affected by metals. It was suggested that serum enzymes such as ALP, AST and ALT may be used as sensitive biomarkers in ecotoxicology thanks to providing early warning of potentially hazardous changes in contaminated aquatic organisms (De La Torre et al. Citation2000; Levesque et al. Citation2002). Quantitatively ALT and AST enzyme activities are the most abundant aminotransferases in the teleostean fish liver (Cowey & Walton Citation1989). It was found that ALT and AST activity was enhanced following nickel concentration increase in the experimental groups. The activity levels of Alanine and aspartate aminotransferase enzyme showed an ascending trend (–). The 80% LC50 group showed maximum increase in ALT among others. Similarly, AST also followed a similar trend in the 40% LC50 group. The same findings were obtained by Naveed et al. (Citation2011) in Channa punctatus. Increased activities of both aminotransferases suggested amplified transamination processes.
4. Conclusion
Last but not the least, a sub-lethal nickel concentration also imposes various adverse impacts on antioxidant activities and liver enzymes; so there is an urgent need to prevent the entering of such hazardous heavy metals into the aquatic environment.
Acknowledgments
Thanks are extended to Mr Taghavi, Head of Research at Payame Noor University of Kohgiluie and Boyer-Ahmad, for his kindly cooperation.
Disclosure statement
No potential conflict of interest was reported by the author.
References
- Atli G, Canli M. 2007. Enzymatic responses to metal exposures in a freshwater fish Oreochoromisniloticus. Comp Biochem Phys C. 145:282–287.
- Bainy ACD, Saito E, Carvalho PSM, Junqueira V. 1996. Oxidative stress in gill, erythrocytes, liver and kidney of Nile tilapia (Oreochromis niloticus) from a polluted site. Aquat Toxicol. 34:151–162. doi: 10.1016/0166-445X(95)00036-4
- Banerjee BD, Seth V, Bhattacharya A. 1999. Biochemical effects of some pesticides on lipid peroxidation and free-radical scavengers. Toxicol Lett. 107:33–47. doi: 10.1016/S0378-4274(99)00029-6
- Bebe FN, Panemangalore M. 2003. Exposure to low doses of endosulfan and chlorpyrifos modifies endogenous antioxidants in tissue of rats. J Environ Sci Heal B. 38:349–363. doi: 10.1081/PFC-120019901
- Bernet D, Schmidt H, Wahli T, Burkhardt-Holm P. 2001. Effluent from a sewage treatment works causes changes in serum chemistry of brown trout (Salmo trutta L.). Ecotox Environ Safe. 48:140–147. doi: 10.1006/eesa.2000.2012
- Claiborne A. 1985. Catalase activity. In: Greenwald RA, editor. Handbook of methods for oxygen radical research. Boca Raton (FL): CRC; p. 283–284.
- Cowey CB, Walton MJ. 1989. Intermediary metabolism. In: Halver JE, editor. Fish Nutrition. San Diego (CA): Academic Press; p. 260–321.
- Dautremepuits C, Paris-Palacios S, Betoulle S, Vernet G. 2004. Modulation in hepatic and head kidney parameters of carp (Cyprinus carpio L.) induced by copper and chitosan. Comp Biochem Phys C. 137:325–33.
- De La Torre FR, Salibian A, Ferrari L. 2000. Biomarkers assessment in juvenile Cyprinus carpio exposed to waterborne cadmium. Environ Pollut J. 109:277–282. doi: 10.1016/S0269-7491(99)00263-8
- Di Giulio RD, Habig C, Gallagher EP. 1993. Effects of black rock harbor sediments on indices of biotransformation, oxidative stress, and DNA integrity in channel catfish. Aquat Toxicol. 26:1–22. doi: 10.1016/0166-445X(93)90002-I
- Di Giulio RT, Washburn PC, Wenning RJ, Winston GW, Jewell CS. 1989. Biochemical responses in aquatic animals: a review of determinants of oxidative stress. Environ Toxicol Chem. 8:1103–1123. doi: 10.1002/etc.5620081203
- Droge W. 2002. Free radicals in the physiological control of cell function. Physiol Rev. 82:47–95. doi: 10.1152/physrev.00018.2001
- Durmaz H, Sevgiler Y, Üner N. 2006. Tissue-specific antioxidative and neurotoxic responses to diazinon in Oreochromisniloticus. Pestic Biochem Phys. 84:215–226. doi: 10.1016/j.pestbp.2005.07.004
- Eisler R. 1998. Nickel Hazards to fish, wildlife, and invertebrates: a synoptic review. Biological Science Report USGS/BRD/BSR-1998-0001, Patuxent Wildlife Research Center, US Geological Survey. p. 95.
- Feng T, Zheng WY, Hong WS. 2000. The effects of benzo(a)pyrene on antioxidant defenses in the liver of Boleophthalmus pectinirostris. Mar Sci. 24:27–30. (in Chinese).
- Finney DJ. 1971. Probit Analysis. Cambridge: Univ. Press; p. 333.
- Gooley GJ, Gavine FM, Dalton W, De Silva SS, Bretherton M, Samblebe M. 2000. Feasibility of aquaculture in dairy manufacturing wastewater to enhance environmental performance and offset costs. Final Report DRDC Project No. MAF001. Marine and Freshwater Resources Institute, Snobs Creek, p. 84.
- Hamed RR, Elawa SE, Farid NM, Ataya FS. 1999. Evaluation of detoxification enzyme levels in Egyptian catfish, Clariaslazera, exposed to dimethoate. Bull Environ Contam Toxicol. 63:789–796. doi: 10.1007/s001289901048
- Ji J, Wu Z, Liu Q, Zhang Y, Ye M, Li M. 1991. An ultramicroanalytic and rapid method for determination of superoxide dismutase activity. Journal of Nanjing Railway Medical College. 10:27–30.
- Jiraungkoorskul W, Upatham ES, Kruatrachue M, Shaphong S, Vichasri-Grams S, Pokethitiyook P. 2003. Biochemical and histopathological effects of glyphosate herbicide on Nile tilapia (Oreochromis niloticus). Environ Toxicol. 18:260–267. doi: 10.1002/tox.10123
- Karan V, Vitorovic S, Tutundzic V, Poleksic V. 1998. Functional enzymes activity and gill histology of carp after copper sulfate exposure and recovery. Ecotoxi Environ Safe. 40:49–55. doi: 10.1006/eesa.1998.1641
- Kawatsu H. 1969. Studies on the anemia of fish-III. An example of macrcytic anemia found in brook trout, Salvelinus fontinalis. Bull Freshwater Res Lab. 19:161–167.
- Khrer JP. 1993. Free radical as mediator of tissue injury and disease. Crit Rev Toxicol, Inform Healtc. 23:21–48. doi: 10.3109/10408449309104073
- Kono Y, Fridovich I. 1982. Superoxide radical inhibits catalase. J Biol Chem. 257:5751–5754.
- Levesque HM, Moon TW, Campbell PGC, Hontela A. 2002. Seasonal variation in carbohydrate and lipid metabolism of yellow perch (Perca flavescens) chronically exposed to metals in the field. Aquat Toxicol. 60:257–267. doi: 10.1016/S0166-445X(02)00012-7
- Mohapatra B, Rengarajan K. 1995. A manual of bioassays in the laboratory and their techniques. CMFRI Special Publication, 64, CMFRI, Cochin, India. p. 75.
- Mourente G, Tocher DR, Díaz E, Grau A, Pastor E. 1999. Relationships between antioxidant enzyme activities and lipid peroxidation products during early development in Dentex dentex eggs and larvae. Aquacult. 179:309–324. doi: 10.1016/S0044-8486(99)00167-2
- Murai T, Andrews JW. 1974. Interaction of dietary α-tocopherol, oxidized menhaden oil and ethoxyquin on channel catfish Ictalurus punctatus. J Nutr. 104:1416–1431.
- Naveed A, Janaiah C, Venkateshwarlu P. 2011. The effects of lihocin toxicity on protein metabolism of the fresh water edible fish, Channa punctatus (Bloch). J Toxicol Environ Health Sci. 3(1):018–023.
- Oakes KD, Van der Kraak GJ. 2003. Utility of the TBARS assay in detecting oxidative stress in white sucker (Catostomuscommersoni) populations exposed to pulp milleffluent. Aquat Toxicol. 63:447–463. doi: 10.1016/S0166-445X(02)00204-7
- Oluah NS. 1999. Plasma aspartate aminotransferase activity in the catfish Clariasalbopuntatus exposed to sub lethal zinc and mercury. Bull Environ Contam Toxicol. 63:343–349. doi: 10.1007/s001289900986
- Parvez S, Raisuddin S. 2005. Protein carbonyls: novel biomarkers of exposure to oxidative stress inducing pesticides in freshwater fish Channa punctata Bloch. Environ Toxicol Pharmaco. 20:112–117. doi: 10.1016/j.etap.2004.11.002
- Pruell RJ, Engelhardt FR. 1980. Liver cadmium uptake, catalase inhibition and cadmium thionein production in the killifish (Fundulus Heteroclitus) induced by experimental cadmium exposure. Marine Environ Res. 3:101–111. doi: 10.1016/0141-1136(80)90019-7
- Reitman S, Frankel S. 1957. A colorimetric method for the determination of oxaloacetic acid glutamic pyruvic transaminase. Am J Clin Pathol. 28:56–63. doi: 10.1093/ajcp/28.1.56
- Sakai T, Murata H, Endo M, Shimomura T, Yamauchi K, Ito T, Yamaguchi T, Nakajima H, Fukudome M. 1998. Severe oxidative stress is thought to be a principal cause of jaundice of yellowtail Seriola quinqueradiata. Aquacult. 160:205–214. doi: 10.1016/S0044-8486(97)00304-9
- Shaluei F, Hedayati A, Jahanbakhshi A, Baghfalaki M. 2012. Physiological responses of great sturgeon (Huso huso) to different concentrations of 2-phenoxyethanol as an anaethetic. Fish Physiol Biochem. 38:1627–1634. doi: 10.1007/s10695-012-9659-4
- Singh HS, Reddy TV. 1990. Effect of copper sulfate on hematology, blood chemistry, and hepatosomatic index of an Indian catfish, Heteropneustesfossillis (Bloch), and its recovery. Ecotoxi Environ Safe. 20:30–35. doi: 10.1016/0147-6513(90)90043-5
- Storey KB. 1996. Oxidative stress: animal adaptation in nature. Brazi J Med Res. 29:1715–1733.
- Us epa. 1980. Ambient water quality criteria for nickel.EPA-440/5-80-060. Office of Water Regulations and Standards. Washington, DC.
- Van der Oost R, Beyer J, Vermeulen N. 2003. Fish bioaccumulation and biomarkers in environmental risk assessment: a review. Environ Toxicol Pharmacol. 13:57–149. doi: 10.1016/S1382-6689(02)00126-6
- Wang WX. 2002. Interaction of trace metals and different marine food chains. Mar Ecol Prog Ser. 243:295–309. doi: 10.3354/meps243295
- Winston GW, Di Giulio RT. 1991. Prooxidant and antioxidant mechanisms in aquatic organisms. Aquat Toxicol. 19:137–161. doi: 10.1016/0166-445X(91)90033-6
- Zikic RV, Stajn S, Pavlovic Z, Ognjanovic BI, Saicic ZS. 2001. Activities of superoxide dismutase and catalase in erytrocyte and plasma transaminases of goldfish (Carassius auratus gibelio Bloch.) exposed to cadmium. Physiol Res. 50:105–111.