ABSTRACT
The influence of live weight (LW) at first implanting on growth performance and carcass characteristics was evaluated in calf-fed Holstein fed a steam-flaked corn-based diet. Treatments were: (1) control (not implanted); (2) first implanted at 267 kg LW; (3) first implanted at 291 kg LW, and (4) first implanted at 321 kg LW. All implanted cattle were re-implanted on d-112 of trial. Both the initial and final implants contained 120 mg of trenbolone acetate and 24 mg of oestradiol. Compared with non-implanted controls, implanting increased (P < .01) overall dry matter intake (DMI, 5.9%), average daily gain (ADG,16.7%), gain efficiency (ADG:DMI; 9.4%), and estimated dietary net energy for maintenance (NEm, 8.6%) and gain (NEg, 9.7%). Increasing LW at first implanting decreased overall DMI (linear effect; P = .01), but did not affect overall ADG (P = .17) or gain efficiency (P = .32). Within the range of 267–321 kg, weight at first implanting did not affect growth-performance or carcass characteristics. Interval growth performance of implanted and non-implanted calves was a predictable function of LW.
1. Introduction
Calf-fed Holstein steers enter the feedlot at characteristically light weights (115–180 kg), where they are fed for periods typically in excess of 300 days (Zinn et al. Citation2005; Duff & McMurphy Citation2007). As with beef breeds, application of growth implants is among the most important management tools for enhancement of ADG, gain efficiency, carcass weights, and longissiumus muscle area (LMA) of Holstein steers (Chester-Jones et al. Citation1990; Perry et al. Citation1991; Zinn et al. Citation1999). However, the optimal weight at first implant application has received limited research attention. Beckett and Algeo (Citation2002) observed that growth performance of calf-fed Holstein steers (initial weight 156 kg) was not negatively impacted by delaying first implant application until calves had been on feed 120 d. The objective of the present study was to evaluate the weight of calves at first implant application on growth-performance and carcass characteristics of calf-fed Holstein steers.
2. Materials and methods
2.1. Animals processing, housing, and feeding
Ninety-six calf-fed Holstein steers (264 ± 3 kg) were used in a 224-d feeding trial, to evaluate the effects of weight at first implanting on growth performance, and carcass traits of calf-fed Holstein steers. Average daily minimum and maximum air temperature and relative humidity during the trial were was 9.5 and 27.6°C, and 42%, respectively. Upon arrival, steers were vaccinated for IBR-PI3 (TSV-2, Zoetis, Florham Park, NJ), clostridials-haemophilus (Ultrabac 7, Zoetis, Florham Park, NJ), pasteurella hemolytic (One Shot, Zoetis, Florham Park, NJ), treated for internal and external parasites (Dectomax, Zoetis, Florham Park, NJ), injected with 500,000 IU of vitamin A (Vita-Jec A&D 500, RXV Products, Porterville, CA), branded and ear-tagged. Calves were grouped by weight into four weight blocks of four pens each (six calves per pen). Pens were 50 m2 with 26.7 m2 overhead shade, equipped with automatic drinkers, and 4.3 m fence-line feed bunks. Treatments were: (1) control (not implanted); (2) first implanted at 267 kg live weight (LW) (I-267); (3) first implanted at 291 kg LW (I-291); and (4) first implanted at 321 kg LW (I-321). All implanted cattle were re-implanted on d-112 of trial. Both the initial and final implant was Revalor-S (containing 120 mg of trenbolone acetate and 24 mg of oestradiol; Merck & Co. Inc., Millsboro, DE). Composition of the growing-finishing diet is shown in . Calves were provided ad libitum access to feed and water. Fresh feed was added to the feed bunk twice daily. Measures of LW and hip height (HH, cm) were obtained at 28-d intervals. HH was measured from the ileum tuber coxae to the floor of the chute, using a metal ruler with a mobile crossbar. For calculation of growth performance, initial LW is the off-truck arrival weight. Interim and final LW were reduced 4% to account for digestive tract fill. Final weights were adjusted to a constant dressing percentage (final LW = carcass weight/0.623).
Table 1. Ingredient composition of diet fed to Holstein steers calves.
2.2. Estimation of dietary NE
Energy gain (EG, Mcal/d) was derived from measures of LW (kg) and ADG (kg/d) according to the equation: EG = (0.0557 W0.75) ADG1.097 (NRC Citation1984). Net energy content of the diet for maintenance and gain were calculated by assuming a constant maintenance energy (EM, Mcal/d) cost of 0.084W0.75 (NRC Citation2000). The NE values of the diets for maintenance and gain were obtained by means of the quadratic formula: NEm, Mcal/kg = (– b – √ b2 – 4ac)/2c (Zinn & Shen Citation1998), where a = – 0.877DMI, b = 0.877EM + 0.41DMI + EG, c = – 0.41EM, and NEg = 0.877NEm – 0.41.
2.3. Carcass data
Hot carcass weights were obtained at time of slaughter. After carcasses chilled for 48 h, the following measurements were obtained: (1) LM area, by direct grid reading of the muscle at the 12th rib; (2) subcutaneous fat over the eye muscle at the 12th rib taken at a location 3/4 the lateral length from the chine bone end (adjusted by eye for unusual fat distribution); (3) kidney, pelvic and heart fat (KPH) as a percentage of HCW; and (4) marbling score (USDA Citation1965; using 3.0 as minimum slight, 4.0 as minimum small, etc.).
2.4. Statistical design and analysis
The trial was analysed as a randomized complete block design, using pen as the experimental unit. Treatments effects were tested using the following orthogonal contrasts: (1) Control (non-implanted) vs. I-267, I-291, and I-321; (2) Linear effect of LW at first implant and (3) Quadratic effect of LW at first implant. Data for WT, and HH traits were analysed using the MIXED procedure of SAS System (SAS Inst. Inc., Cary, NC) as a repeated measures analysis, to allow for heterogeneous variances and correlations among different time intervals on test (Littell et al. Citation1998). The linear mixed model used for this analysis includes the overall mean, treatment, day, and treatment with day interaction as fixed effects and pen, pen by treatment, and steers within pen by treatment as random components. Estimation was carried out using the method of REML, assuming a variance–covariance structure and correlations over time on the test. The variance–covariance components were subjected to a test of hypothesis using the option COVTEST. The resulting covariance between random parameters was different from zero (P < .01). Therefore, different variance–covariance structures were used in TYPE option of REPEATED statement. Final structure was selected based on values closest to zero for the Akaike’s and Schwarz’s Bayesian information criteria. Least square means for treatment, days and interaction effects were used in multiple comparison and significance was declared at P < .05, unless otherwise. To test hypothesis about parallel trends over time for the treatments, day effect as linear and quadratic regression was introduced into linear mixed model, looking for statistical evidence of treatment by day interaction. Full models containing quadratic regression were reduced by removing factors that did not contribute significantly to the model. Hypothesis for equal regressions was realized. Also, one prediction equation for WT trait was obtained by inclusion of HH traits plus interactions and determining best fit applying coefficient of determination (R2), MSE and Mallow’s coefficient [C(p)] criterions via the STEPWISE option of REG procedure.
Procedures for animal care and management were conducted under protocols approved by the University of California, Animal Use and Care Advisory Committee.
3. Results and discussion
Treatment effects on growth performance of calf-fed Holstein steers are presented in . Compared with non-implanted controls, implanting increased (P < .01) overall 224-d DMI (5.9%), ADG (16.7%), gain efficiency (ADG:DMI; 9.4%), and estimated dietary NEm (8.6%) and NEg (9.7%). These results are consistent with previous studies involving calf-fed Holstein steers, wherein implanting improved ADG by 12–18%, and gain efficiency by 7–12% (Chester-Jones et al. Citation1990; Perry et al. Citation1991; Zinn et al. Citation1999). Likewise, Ainslie et al. (Citation1992) observed that compared with non-implanted steers, implanting Holstein steers enhanced estimated NEm and NEg (8.7 and 11.1%, respectively). They imputed this improvement to a 22% reduction in energy requirements for maintenance. Alternatively, the improved apparent dietary NE for implanted steers may be a reflection of the non-nutritional action of implants on composition of gain, enhancing net protein retention, and hence, leaner-than-expected tissue growth for the specified LW and ADG (Reinhardt Citation2007).
Table 2. Effects of LW at first implant application on growth performance and dietary energetics of calf-fed Holstein steers on entire period of growing-finishing (224-d).
Increasing LW at first implanting decreased overall DMI (linear effect; P = .01), but did not affect overall ADG (P = .17) or gain efficiency (P = .32). Beckett and Algeo (Citation2002) likewise observed that delaying implanting during the initial and growing phase did not influence overall growth-performance of calf-fed Holstein steers. However, very little work has been reported evaluating the limiting initial weight or weight range when the initial implant might be applied before overall growth-performance is impacted. In the present study, growth-performance response was numerically optimal in steers receiving their first implant at an average weight of 291 kg.
The relationship between average LW and ADG as affected by implant treatments is shown in . Daily weight gain of both implanted and non-implanted steers was maximal as steers approached an average weight of approximately 370 kg, averaging 1.76 and 1.50 kg/d, respectively. From roughly 370 kg until harvest (598 kg), ADG of implanted steers was similar (P > .10). Daily weight gain of non-implanted steers decreased markedly after steers reached an average shrunk weight of 510 kg. A comparable marked decline in ADG occurred in implanted steers as they reached a shrunk weight of approximately 550 kg. As a function of average shrunk weight (AW, kg), incremental ADG, kg can be explained by the equations:
Figure 1. Performance of ADG, kg as a function of average LW, kg by treatment: (No Imp) ADG, kg = 1.7444–0.009344 AW + 0.00004213 AW2 – 0.00000005178 AW3, R2 = .796; (I-267) ADG, kg = 0.7188 + 0.002536 AW + 0.000005218 AW2 – 0.00000001394 AW3, R2 = .895; (I-291) ADG, kg = 1.707–0.009025 AW + 0.00004094 AW2 – 0.00000004661 AW3, R2 = .874; (I-321) ADG, kg = 1.952–0.01193 AW + 0.00004994 AW2 – 0.00000005512 AW3, R2 = .740.
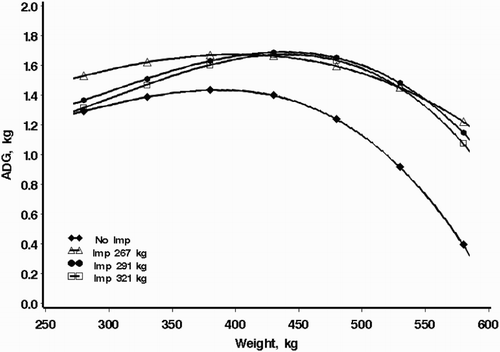
The relationship between AW and DMI as affected by dietary treatments is shown in . Daily DMI of non-implanted steers was maximal as steers approached an AW of approximately 470 kg. In the case of implanted steers, daily DMI was maximal as steers approached an AW of approximately 548 kg. Thereafter, daily DMI began a decline. As a function of AW, incremental DMI, kg/d can be explained by the equations:
Figure 2. Performance of DMI, kg/d as a function of average LW, kg by treatment: (No Imp) DMI, kg/d = 19.925–0.13977AW + 0.000437895AW2 – 0.000000408AW3, R2 = .985; (I-267) DMI, kg/d = 1.1721 + 0.01162AW + 0.000036371AW2 – 0.000000054AW3, R2 = .990; (I-291) DMI, kg/d = 4.5939–0.01810AW + 0.000112629AW2 – 0.000000115AW3, R2 = .993; (I-321) DMI, kg/d = 6.3112–0.03019AW + 0.000141208AW2 – 0.000000139AW3, R2 = .995.
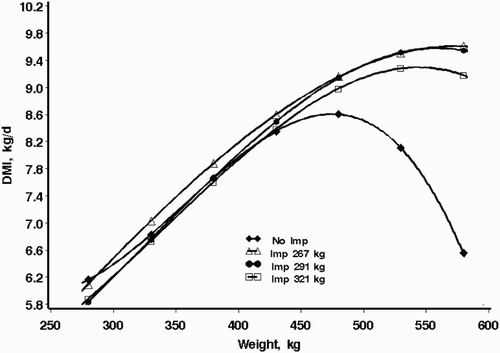
As can be seen from the above two equations, average shrunk weight, alone, does a very good job of explaining DMI. However, the practicality of their use is limited as they do not incorporate differences in dietary NE and ADG.
Conventionally, DMI may be also predicted as a function of LW, ADG, and NE value of the diet according to the relationship: DMI, kg/d = ((0.084LW0.75)/2.25) + ((0.077 LW0.75 ADG1.097)/1.56), where 0.084 is the assumed maintenance coefficient for Holstein steers, and 2.25 and 1.56 are the expected NE values of the diet for maintenance and gain, respectively (NRC Citation1984; ). This relationship was not derived experimentally. Rather, it assumes that EG for calf-fed Holstein steer calves is similar to that of medium-frame beef steer calves (0.077 LW0.75 ADG1.097; NRC Citation1984), and that the maintenance requirement for Holstein steers is 9% greater (0.084 vs. 0.077; Garrett Citation1971). The relationship between observed and predicted DMI using this approach are:
For implanted and non-implanted steers, the ratio of predicted:observed DMI averaged 1.06 ± 0.08 and 0.98 ± 0.06, respectively. With implanted steers, the conventional equation consistently overestimated DMI across the range of LW, whereas with non-implanted steers, DMI was largely underestimated. Although application of this conventional approach has yielded consistent results for calf-fed Holstein steers with respect to overall average observed vs. expected dietary NE and DMI (Ramirez et al. Citation1998; Zinn et al. Citation2000; Carrasco et al. Citation2013), it may be less reliable in estimation of DMI at specific points across the practical LW range (Carrasco et al. Citation2013), or in comparisons where treatments are expected to affect composition of gain (as is the case with growth implants).
NRC (Citation2000) makes an adjustment for animal difference in mature weight (MW) in order to standardize the steer calf equation (NRC Citation1984). This is accomplished by multiplying LW by a scaling factor (478/MW). Using a process of iteration to solve for minimum sums of squares for difference between observed and predicted DMI [DMI, kg/d = ((0.084 LW0.75)/2.25) + ((0.077 ((478/MW)LW)0.75 ADG1.097)/1.56)], the corresponding ‘MW’ for implanted and non-implanted steers are 547 and 454 kg, respectively. The relationship between observed and predicted DMI across the range of LW measures using this approach are:
For implanted and non-implanted steers, the ratio of predicted:observed DMI averaged 1.00 ± 0.03 and 1.00 ± 0.03, respectively can be used to estimate all coefficients and exponents in the application of steer calf equation for calf-fed Holsteins.
DMI, kg/d = ((0.085LW0.66)/2.25) + ((0.175 ((478/MW)LW)0.66 ADG0.234)/1.56), where MW was 550 and 539 for implanted and non-implanted steers, respectively. The relationship between observed and predicted DMI using this approach are:
For implanted and non-implanted steers, the ratio of predicted:observed DMI averaged 1.00 ± 0.03 and 1.00 ± 0.03, respectively.
The relationship between average AW and gain efficiency is shown in . As expected, gain efficiency of both non-implanted and implanted steers decreased with increasing AW. For non-implanted steers, gain efficiency declined linearly (gain feed = 0.335–0.000405 AW, P < .01, r2 = .89) until steers achieved an average LW of 510 kg. Subsequently, gain efficiency declined more precipitously (0.00228 units/kg increase in LW above 510 kg). In the case of implanted steers, gain efficiency declined linearly across the weight range of the study (gain efficiency = 0.3478–0.000368AW, P < .01, r2 = .83).
Figure 3. Gain efficiency, ADG/DMI as a function of average LW, kg by treatment: (No Imp) ADG/DMI = 0.33547–0.000405375AW, R2 = .896; (I-267) ADG/DMI = 0.37151–0.000416106AW, R2 = .930; (I-291) ADG/DMI = 0.34619–0.000363499AW, R2 = .877; (I-321) ADG/DMI = 0.32580–0.000323609AW, R2 = .697.
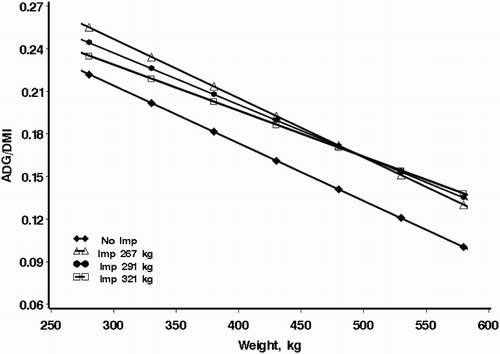
As mentioned previously, overall gain efficiency was not different among implanted groups. This effect was due to a compensating increase in gain efficiency, particularly noticeable during the final 28 days on feed (linear effect, P < .01). It is expected that relative performance response to growth implants declines with time post-implantation (Montgomery et al. Citation2001). Reinhardt (Citation2007) observed that re-implanting cattle around d 70 during these declines boosted performance response to a level equal to or greater than that of the previous implant. In the present study, treatments I-291 and I-321 were re-implanted at 84 and 56 d following the initial implant.
Consistent with Schlegel et al. (Citation2006), HH was a good predictor of LW in Holstein calves. For non-implanted calves, HH explained 77% of the variation in LW. For calves first implanted at an average weight of 267, 291, and 321 kg, HH explained 82%, 83%, and 84%, respectively, of the variation in LW. The close relationship between LW and LW:HH ratio of non-implanted and implanted steers is shown in . As LW increases, LW:HH ratio linearly increases (LW:HH, kg/cm = 0.405 + 0.00607LW, r2 = .997). Consistent with Solis et al. (Citation1989), implanting enhances muscularity largely to the extent that final LW of implanted steers is greater than that of non-implanted steers, allowing for greater LW:HH ratio.
Figure 4. Weight/HH, kg/cm as a function of average LW, kg by treatment: (No Imp) AW/HH, kg/cm = 0.38322 + 0.006148055AW, R2 = .9966; (I-267) AW/HH, kg/cm = 0.42581 + 0.006075823AW, R2 = .9971; (I-291) AW/HH, kg/cm = 0.38869 + 0.006048098AW, R2 = .9981; (I-321) AW/HH, kg/cm = 0.42129 + 0.006011446AW, R2 = .9972.
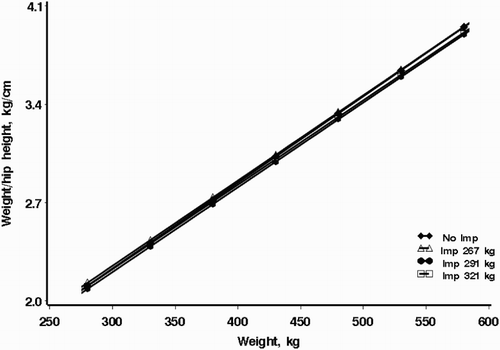
Treatment effects on carcass characteristics are shown in . LW at first implanting did not affect (P > .10) carcass characteristics of implanted steers. Implanting increased (8.8%, P < .01) carcass weight and LMA (9.2%, P < .01), and tended to decrease KPH fat (14%, P = .08) compared with non-implanted steers. LW at first implanting did not affect (P > .10) dressing percentage, fat thickness, yield grade and quality grade. Increased LMA has been a consistent response in comparisons of implanted vs. non-implanted cattle (Bartle et al. Citation1992; Samber et al. Citation1996; Foutz et al. Citation1997; Hermesmeyer et al. Citation2000; Roeber et al. Citation2000). This response is largely attributable to increased carcass weight (Campbell Citation2005). Consistent with the present study, growth implants have shown little or no effect on fat thickness (Eversole et al. Citation1989; Wilson et al. Citation1999), KPH percentage (Bartle et al. Citation1992) and dressing percentage (Samber et al. Citation1996).
Table 3. Effects of LW at first implant application on carcass characteristics of calf-fed Holstein steers
Reduced marbling scores, resulting in lower quality grades, is a concern when combination implants (trenbolone acetate-estrogen implants) are applied (Apple et al. Citation1991; Bartle et al. Citation1992), and is exacerbated by re-implant frequency (Zinn et al. Citation1999). In the present study, marbling score was numerically lower for implanted steers (5.5 vs. 5.0 for non-implanted vs. implanted steers). However, this difference was not detected as statistically significant (P = .17). This effect may be attributable to differences in terminal LW between non-implanted and implanted steer treatments. Perry et al. (Citation1991) observed that implanted Holstein steers required an additional 25–45 kg LW to achieve choice grade compared to non-implanted steers. In the present study the average terminal weight of implanted steers was 49 kg greater than that of non-implanted steers. Consistent with Nour et al. (Citation1983) and Thooney (Citation1987), implanted calf-fed Holstein steers deposited adequate marbling to meet industry standards.
4. Implications
Application of growth implants in calf-fed Holstein steers has marked positive effects on daily weight gain, gain efficiency, carcass weight and LMA. Within the range of 267–321 kg, weight at first implanting did not affect growth-performance or carcass characteristics. Interval growth performance of implanted and non-implanted calves is a predictable function of LW.
Disclosure statement
No potential conflict of interest was reported by the authors.
References
- Ainslie SJ, Fox DG, Perry TC. 1992. Management system for Holstein steers that utilize alfalfa silage and improve carcass value. J Anim Sci. 70:2643–2651.
- Apple JK, Dikeman ME, Simms DD, Kuhl G. 1991. Effects of synthetic hormone, singularly or in combinations, on performance, carcass traits, and longissimus muscle palatability of Holstein steers. J Anim Sci. 69:4437–4448.
- Bartle SJ, Preston RL, Brown RE, Grant RJ. 1992. Trenbolone acetate/estradiol combinations in feedlot steers: dose-response and implant carrier effects. J Anim Sci. 70:1326–1332.
- Beckett JL, Algeo I. 2002. Effects of delayed implant protocols on performance, carcass characteristics and meat tenderness in Holstein steers. J Anim Sci. 80(Suppl. 1):49 ( Abstract).
- Campbell C. 2005. Dairy beef implants strategies: the benefits of TBA containing implants for Holstein steers. Pages 237–246 in Proc. Managing and Marketing Quality Holstein Steers, Rochester, MN.
- Carrasco R, Arrizon AA, Plascencia A, Torrentera NG, Zinn RA. 2013. Comparative feeding value of distillers dried grains plus solubles as a partial replacement for steam-flaked corn in diets for calf-fed Holstein steers: characteristics of digestion, growth performance, and dietary energetics. J Anim Sci. 91:1801–1810. doi: 10.2527/jas.2012-5260
- Chester-Jones H, Ziegler DM, Meiske JC, Larson BT. 1990. Performance and carcass characteristics of Holstein steers in the feedlot with or without Finaplix vs. Synovex implants during the last 100 days. University of Minnesota. Report of Progress; p. 63–64.
- Duff GC, McMurphy CP. 2007. Feeding Holstein steers from start to finish. Vet Clin Food Anim. 23:281–297. doi: 10.1016/j.cvfa.2007.04.003
- Eversole DE, Fontenot JP, Kirk DJ. 1989. Implanting trenbolone acetate and estradiol in finishing beef steers. Nutr Rep Int. 39:995–1007.
- Foutz CP, Dolezal HG, Gardner TL, Gill DR, Hensley JL, Morgan JB. 1997. Anabolic implant effects on steer performance, carcass traits, sub-primal yields and longissimus muscle properties. J Anim Sci. 75:1256–1265.
- Garrett WN. 1971. Energetic efficiency of beef and dairy steers. J Anim Sci. 32:451–456.
- Hermesmeyer GN, Berger LL, Nash TG, Brandt Jr. RT. 2000. Effects of energy intake, implantation and subcutaneous fat end point on feedlot steer performance and carcass composition. J Anim Sci. 78:825–831.
- Littell RC, Henry PR, Ammerman CB. 1998. Statistical analysis of repeated measures data using SAS Procedures. J Anim Sci. 76:1216–1231.
- Montgomery TH, Dew PF, Brown MS. 2001. Optimizing carcass value and the use of anabolic implants in beef cattle. J Anim Sci. 79:(E Suppl.)E296.
- Nour AYM, Thooney ML, Stouffer JR, White Jr. WRC. 1983. Changes in carcass weight and characteristics with increasing weight of large and small cattle. J Anim Sci. 57:1154–1165.
- NRC. 1984. Nutrient requirements of beef cattle. 6th rev. ed. Washington, DC: Natl. Acad. Press.
- NRC. 2000. Nutrient requirements of beef Cattle. Updated 7th rev. ed. Washington, DC: Natl. Acad. Press.
- Perry TC, Fox DG, Beerman DH. 1991. Effect of an implant of trenbolone acetate and estradiol on growth, feed efficiency and carcass composition of Holstein and beef steers. J Anim Sci. 69:4696–4702.
- Ramirez JE, Alvarez EG, Montaño M, Shen Y, Zinn RA. 1998. Influence of dietary magnesium level on growth performance and metabolic responses of Holstein steers to laidlomycin propionate. J Anim Sci. 76:1753–1759.
- Reinhardt C. 2007. Growth-promotant implants: managing the tools. Vet Clin North Am Food Anim Prac. 23:309–319. doi: 10.1016/j.cvfa.2007.03.004
- Roeber DL, Cannel RC, Belk KE, Miller RK, Taum JD, Smith GC. 2000. Implant strategies during feeding: impact on carcass grades and consumer acceptability. J Anim Sci. 78:1867–1874.
- Samber JA, Tatum JD, Wray MI, Nichols WT, Morgan JB, Smith GC. 1996. Implant program effects on performance and carcass quality of steer calves finished for 212 days. J Anim Sci. 74:1470–1476.
- Schlegel ML, Bergen WG, Schroeder AL, VandeHaar MJ, Rust SR. 2006. Use of bovine somatotropin for increased skeletal and lean tissue growth of Holstein steers. J Anim Sci. 84:1176–1187.
- Solis JC, Byers FM, Schelling GT, Greene LW. 1989. Anabolic implant and frame size effects on growth regulation, nutrient re-partitioning and energetic efficiency of feedlot steers. J Anim Sci. 67:2792–2801.
- Thooney ML. 1987. Growth, feed efficiency and variation of individually fed Angus, Polled Hereford and Holstein steers. J Anim Sci. 65:1–8.
- USDA. 1965. Official United States standards for grades of carcass beef. USDA. Consumer and marketing services, service and regulatory announcement No. 99. Washington, DC: U.S. Government Printing Office.
- Wilson LL, Smith JL, Swanson DL, Mills EW. 1999. Implant sequence effects in intact males Holstein veal calves; Carcass characteristics. J Anim Sci. 77:3133–3139.
- Zinn RA, Alvarez EG, Montano M, Ramirez JE, Shen Y. 1999. Implant strategies for calf-fed Holstein steers. Proc Western Sec Am Soc Anim Sci. 50:306–309.
- Zinn RA, Alvarez EG, Montaño MF, Ramirez JE. 2000. Interaction of protein nutrition and laidlomycin on feedlot growth performance and digestive function in Holstein steers. J Anim Sci. 78:1768–1778.
- Zinn RA, Corona L, Plascencia A. 2005. Fat and protein supplementation of calf-fed Holstein steers. Paper # 89 in Proc. Managing and Marketing Quality Holstein Steers. Rochester, MN.
- Zinn RA, Shen Y. 1998. An evaluation of ruminally degradable intake protein and metabolizable amino acid requirements of feedlot calves. J Anim Sci. 76:1280–1289.