ABSTRACT
This study aimed to investigate the effect of urea (U) and molasses (M) supplement on the nutritive value of cassava pulp and in vitro gas fermentation. The ensiling study was randomly assigned according to a 4 × 4 factorial arrangement in a completely randomized design. The first factor was U supplementation at U0, U2, U4, and U6% and the second was M supplementation at M0, M2, M4, and M6% of dry matter, respectively. After 14 days of ensiling, treated cassava pulp was sampled for chemical composition analysis and subsequently used to study in vitro fermentation. The results revealed that increasing U supplement levels could increase crude protein and decrease fibre contents of treated cassava pulp (P < .05). Increasing U and M supplement levels increased gas production and in vitro true digestibility (P < .05). Total bacteria, Fibrobacter succinogenes and Ruminococcus flavefaciens were increased in cassava pulp treated with U and M supplemented groups (P < .05). Based on this experiment, it could be concluded that U4 and M4% supplement could improve the nutritive value of treated cassava pulp and increase gas production, in vitro digestibility, and the growth of dominant cellulolytic bacterial population. However, further research should be conducted on the use of treated cassava pulp in ruminant feeding.
1. Introduction
Cassava root contains high levels of energy and has been used as a source of readily fermentable energy in ruminant rations (Wanapat Citation2003). Cassava starch production is a huge and growing industry in Thailand, with approximately 10 million tonnes of fresh cassava roots used annually (Chauynarong et al. Citation2009). Cassava pulp is a by-product which constitutes approximately 30% of the original weight of roots (Ghimire et al. Citation2015). Approximately 1.5–2.0 million tonnes of cassava pulp are produced annually from the entire cassava starch industry. It contains abundant starch (60%), while the other main carbohydrates are cellulose and hemicelluloses (20%) (Sriroth et al. Citation2000; Manish and Banerjee Citation2008; Kosugia et al. Citation2009). Cassava pulp offers an alternative to high-starch grains, and can be used as an energy source in ruminant diets, but is low in crude protein (CP) (Lounglawan et al. Citation2010). Ensiling is a feed preservation method based on natural lactic acid fermentation under anaerobic conditions where anaerobic microbes build up organic acids, mainly lactic acid, by using fermentable carbohydrates (Gollop et al. Citation2005; Ki et al. Citation2009). To improve animal feed quality, additives such as chemicals, enzymes, and bacterial agents can be employed. Wanapat, Kang, Khejornsart et al. (Citation2013) reported that supplementation of urea (U) and molasses (M) could improve the quality of whole-crop rice silage in terms of nutritive value and rumen degradation. Addition of carbon and nitrogen sources could improve the quality of feed and have subsequent effects on rumen degradation characteristics and production in ruminants. Therefore, the objective of this study was to determine the effect of U and M treatment on the nutritional value of cassava pulp and in vitro rumen fermentation.
2. Materials and methods
2.1. Ensiling of cassava pulp
Cassava pulp was treated to the respective supplementation according to a 4 × 4 factorial arrangement in a completely randomized design (CRD). Factor A was U supplementation levels at 0%, 2%, 4%, and 6% and factor B was M supplementation levels at 0%, 2%, 4%, and 6% of cassava pulp dry matter (DM), respectively. Cassava pulp was mixed with U and M, and then packed into plastic containers kept at ambient temperature (about 25–30°C). All treatment combinations were done in triplicates at 300 g each. After 14 days, the treated cassava pulp was sampled for chemical compositions analysis. The samples were divided into two parts: the first part was for DM analysis (AOAC Citation1997) and the second part was dried in a forced-air oven at 60°C for 96 hour, ground through a 1-mm stainless steel screen (Cyclotec 1093 Sample mill, Tecator, Hoganas, Sweden), and analysed for organic matter (OM) and CP according to the AOAC (Citation1997) method. The method of Van Soest et al. (Citation1991) was used to determine neutral detergent fibre (NDF) and acid detergent fibre (ADF) on an ash-free basis using an Ankom fibre analyser incubator (Ankom Technology, Fairport, NY).
2.2. In vitro gas techniques
2.2.1. Experimental design and dietary treatments
The experimental design was a 4 × 4 factorial arrangement in a CRD, with three replications per treatment including triplicates of blank (medium only) in three incubation runs. The dietary treatments were 16 kinds of treated cassava pulp. Substrates of treated cassava pulp were milled to pass through a 1-mm screen (Cyclotec 1093 Sample mill, Tecator, Hoganas, Sweden).
2.2.2. Animals and preparation of rumen inoculums
Two rumen-fistulated dairy steers with body weight (BW) of 230 ± 15 kg were used as rumen fluid donors. The animals were fed on concentrated diet (16% CP) at 0.5% BW and rice straw was used as the main roughage before collecting the rumen fluid. Animals were placed on a routine for at least 20 days and kept in individual pens with free choice of clean fresh water and mineral blocks. On day 21, about 2000 ml of rumen liquor was obtained from both animals before the morning feeding. The rumen fluid was filtered through four layers of cheesecloth into pre-warmed thermo flasks and then transported to the laboratory.
2.2.3. In vitro fermentation of substrates
Samples of 0.2 g of treated cassava pulp were weighed into 60 ml serum bottles, respectively. The method used for in vitro fermentation was based on the technique described by Menke et al. (Citation1979) and strict anaerobic techniques were used in all steps during the rumen fluid transferring and incubation periods (Menke et al. Citation1979; Makkar et al. Citation1995). The bottles were categorized into separate sets of sample incubations for the determination of gas production kinetics, in vitro digestibility, and fermentation end products with three replications per treatment including triplicates of blank (medium only). The bottles with the mixture of substrate treatments sealed with rubber stoppers and aluminium caps were pre-warmed in a water bath at 39°C for 1 h before filling with 30 ml of rumen inoculum mixture (Menke and Steingass Citation1988).
2.2.4. Medium solution preparation
The medium preparation per run (4500 ml) was as described by Makkar et al. (Citation1995). The reduced medium (3000 ml) consists of 1425 ml distilled water, 720 ml rumen buffer solution (35.0 g NaHCO3 and 4 g NH4HCO3 made up to 1 l with distilled water), 720 ml macro-mineral solution (6.2 g KH2PO4, 5.7 g Na2HPO4, 2.22 g NaCl, and 0.6 g MgSO4. 7H2O made up to 1 l with distilled water), 0.36 ml micro-mineral solution (10.0 g MnCl2. 4H2O, 13.2 g CaC12. 2H2O, 1 g CoCl2 .6H2O, and 8.0 g FeC13. 6H2O made up to 100 ml with distilled water), 3.66 ml resazurin (0.1 g made up to 100 ml with distilled water), and 148.5 ml freshly prepared reduction solution (142.5 ml distilled water, 1008 mg Na2S. 9H2O, and 6 ml 1 M NaOH). Then, 1500 ml of rumen fluid from dairy steers (2:1; reduced medium: rumen fluid) was mixed with the reduced medium as described by Makkar et al. (Citation1995). The mixture was stirred continuously under CO2 at 39°C using a magnetic stirrer fitted with a hot plate under continuous flushing with CO2. A portion (30 ml) of the rumen fluid medium was transferred into each bottle and incubated in a water bath at 39°C as described by Blummel and Ørskov (Citation1993).
2.2.5. Sample collection and analysis
Gas production kinetics: During the incubation, the gas production kinetics was recorded at 0, 1.5, 3, 6, 8, 12, 18, 24, 48, 72, and 96 h following extraction using glass syringes. Cumulative gas production data were calculated based on the gas production at each time using the Fit Curve program fitted to the model of Orskov and McDonald (Citation1979) as follows: y = a + b (1−e(−ct)), where a = the gas production from the immediately soluble fraction, b = the gas production from the insoluble fraction, c = the gas production rate constant for the insoluble fraction (b), t = the incubation time, (a + b) = the potential extent of gas production, and y = gas produced at time ‘t’.
In vitro digestibility: At 48-hour post-inoculation, the contents of the incubation substrate were washed with 100 ml of neutral detergent solution and then filtered through pre-weighed Gooch crucibles (40 mm porosity), and residual DM was weighed and estimated for in vitro true digestibility based on the following equation according to Van Soest and Robertson (Citation1985): True digestibility (TD) = ((DM of feed taken for incubation-NDF residue) × 100)/DM of feed taken for incubation.
Extraction of genomic deoxyribonucleic acid (DNA): Community DNA was extracted from 0.5 g of rumen content (fluid and digesta) by the repeated bead beating plus column (RBB + C) method (Yu and Morrison Citation2004). In brief, the RBB + C method employs two rounds of bead beating in the presence of NaCl and sodium dodecyl sulfate, followed by sequential ammonium acetate and isopropanal precipitations. The precipitated nucleic acids were then treated with RNase A and proteinase K, and the DNA was purified using columns from QIAGEN DNA Mini Kit (QIAGEN, Valencia, CA), according to the manufacturer’s recommendations.
Primers and real-time polymerase chain reaction (real-time PCR): The targeted microorganisms were total bacteria and three cellulolytic bacteria (Fibrobacter succinogenes, Ruminococcus albus, and Ruminococcus flavefaciens). The primers used for the real-time PCR were as follows: primers for F. succinogenes, Fs219f (5’-GGT ATG GGA TGA GCT TGC-3’) and Fs654r (5’-GCC TGC CCC TGA ACT ATC-3’), were selected to allow the amplification (446-bp product) of all 10 F. succinogenes strains deposited in GenBank. For R. albus, primers were Ra1281f (5’-CCC TAA AAG CAG TCT TAG TTC G-3’) and Ra1439r (5’-CCT CCT TGC GGT TAG AAC A-3’) (175-bp product). R. flavefaciens primers, Rf154f (5’-TCT GGA AAC GGA TGG TA-3’) and Rf425r (5’-CCT TTA AGA CAG GAG TTT ACA A-3’), were also selected to allow species–species amplification (295 bp) of all seven R. flavefaciens strains deposited in GenBank. All these primer sets were previously published by Koike and Kobayashi (Citation2001). Primers for total bacteria were F (5’-GC-clamp-CCT ACG GGA GGC AGC AG-3’) and R (5’-GWA TTA CCG CGG CKG CTG-3’) according to Lane (Citation1991).
Regular PCR conditions for F. succinogenes were as follows: 30 s at 94°C for denaturing, 30 s at 60°C for annealing, and 30 s at 72°C for extension (48 cycles), except for 9-min denaturation in the first cycle and 10-min extension in the last cycle. Amplification of 16S rRNA for the other two species was carried out similarly, except an annealing temperature of 55°C was used. Regular PCR conditions were as follows: 30 s at 94°C for denaturing, 30 s at 60°C for annealing, and 30s at 72°C for extension (48 cycles), except for 9-min denaturation in the first cycle and 10-min extension in the last cycle. The targeted populations were total bacteria and cellulolytic bacteria (i.e. R. albus, F. succinogenes, and R. flavefaciens). Four sample-derived standards (for total bacteria, R. albus, F. succinogenes, and R. flavefaciens) were prepared from a pooled set of all treatments of community DNA. The regular PCR was used to generate sample-derived DNA standards for each real-time PCR assay. Then the PCR product was purified using a QIA quick PCR purification kit (QIAGEN, Inc., Valencia, CA) and quantified using a spectrophotometer. For each sample-derived standard, copy number concentration was calculated based on the length of the PCR product and the mass concentration (Yu et al. Citation2005). The target DNA was quantified by using serial 10-fold dilutions from 101 to 109 DNA copies of the previously quantified DNA standards (Yu et al. Citation2005). Real-time PCR amplification and detection were performed in a Chromo 4TM system (Bio-Rad, USA). All samples were assayed in duplicate in a 20 μl reaction mixture containing 4–6 mM MgCl2, 10 μl of Mastermix (including Taq DNA polymerase, reaction buffer, dNTP mixture, MgCl2, and SybrGreen), 2 μl of DNA template, and 0.8 μl of each primer (10 μM/μl).
2.3. Statistical analysis
All obtained data were subjected to the General Linear Models (GLM) procedures of SAS (Citation1996) according to a 4 × 4 factorial arrangement in CRD. Differences among treatment means were contrasted by Tukey’s Multiple Comparison Test (Crichton Citation1999). Comparison between plant oil source supplementation was tested by orthogonal contrast.
3. Results and discussions
The effect of U and M treatments on the nutritive value of cassava pulp is shown in . Increasing U supplementation increased the CP content of cassava pulp (P < .01). In addition, NDF and ADF contents of treated cassava pulp were decreased by either U or M inclusion. The nutritive values were relatively higher than the value of Ki et al. (Citation2009), especially the CP content. This indicated that the combination of U and M supplementation could enhance the quality of the cassava pulp ensilage. Wanapat, Kang, Khejornsart et al. (Citation2013) and Giang et al. (Citation2016) also found that the supplementation of U and M could improve the quality of whole-crop rice silage and Leucaena leucocephala silage by increasing CP, and reducing NDF and ADF contents. Gas production affected by different treated cassava pulp in the present study is presented in and . Supplementation of U and M affected the cumulative gas production (96 h), gas production for the insoluble fraction (b), the gas production rate constant for the insoluble fraction (c), and the potential extent of gas production (a + b) (P < .05), but did not affect gas production from the immediately soluble fraction (a) (P > .05). The increase in gas production kinetics in the present study could be due to the addition of U in the silage which could improve rumen fermentation and nutrient digestibility (Miller et al. Citation2005; Oji et al. Citation2007; Cherdthong et al. Citation2011; Sweeny et al. Citation2014).
Figure 1. Effect of fermented cassava pulp on cumulative gas production at different times of incubation in dairy steers rumen fluid.
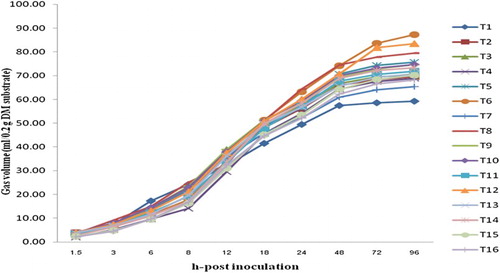
Table 1. Chemical compositions of treated cassava pulp affected by urea and molasses treatment.
Table 2. Effect of treated cassava pulp on gas production kinetics and degradability from in vitro incubation.
The effect of cassava pulp fermented with U and M on in vitro digestibility is shown in . The in vitro true digestibility was increased with the increasing level of U and M supplement in treated cassava pulp. Silvestre et al. (Citation1977) reported that the voluntary feed intake of cattle reduced with the increasing level of U concentration in the M solution, which could be due to the low palatability of U. In the present study, the in vitro true digestibility in U and/or M supplemented groups was higher than that in the non-supplemented group and this could be due to a corresponding increase in microbe growth. However, there was no different effect among each level of U and M supplement. Rumen fermentation and digestibility were increased with the increasing levels of U supplement, which could be due to the increased rate of rumen microorganisms growth resulting from the presence of more available N in the form of ammonia from the hydrolysis of U (Boucher et al. Citation2007; Khattab et al. Citation2013; Kang et al. Citation2015). In addition, Wanapat, Kang, Khejornsart et al. (Citation2013) reported that the supplementation of U and M could increase rumen degradability of whole-crop rice silage.
The application of real-time PCR to quantify predominant cellulolytic bacteria (16S rRNA) targets revealed that total bacteria, F. succinogenes, and R. flavefaciens were significantly increased ( and ; P < .05) with the increasing level of M in treated cassava pulp. Similarly, Salais et al. (Citation1977) reported that both the nature of fibre and protein content of the forage are important contributing factors in determining the responses to M/U diets. It is well known that cellulolytic bacteria utilize NH3 for their growth (Bryant Citation1973). They were unable to grow on other nitrogen sources in the absence of NH3 (Russell et al. Citation2009). Cassava root contains high levels of readily fermentable energy and could be used in combination with readily available NPN sources such as U in ruminant rations and this could improve the growth of rumen bacteria (Wanapat and Kang Citation2013; Wanapat, Kang, Polyorach Citation2013). All groups of bacteria were enhanced by the supplementation group, especially in the treatment with high M and U ratio (P < .05).
Figure 2. Genomic DNA agarose gel electrophoresis to check the DNA quality (1 kb = ladder; T = treatment).
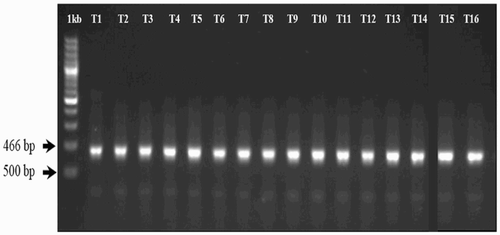
Table 3. The effect of urea- and molasses-fermented cassava pulp on total bacterial and predominant cellulolytic bacterial after in vitro incubation with rumen fluid.
4. Conclusion and recommendations
Based on this study, it could be concluded that cassava pulp nutritive value could be enhanced by U and M supplementation. Moreover, the treatment of U and M on cassava pulp could improve gas production kinetics, in vitro digestibility, and the growth of dominant cellulolytic bacterial population. It is suggested that the level of U and M treatment be at U4 and M4% of cassava pulp DM. However, further research should be conducted on the use of treated cassava pulp in ruminant feeding.
Acknowledgements
The authors would like to express their most sincere thanks to the Tropical Feed Resources Research and Development Center (TROFREC), Department of Animal Science, Faculty of Agriculture, Khon Kaen University, and Department of Animal Production Technology, Faculty of Agro-Industrial Technology, Kalasin University for experimental animals, laboratory, and the use of research facilities.
Disclosure statement
No potential conflict of interest was reported by the authors.
References
- AOAC. 1997. Official methods of analysis. 16th ed. Gaithersburg (MD): Association of Official Analytical Chemists.
- Blummel M, Orskov ER. 1993. Comparison of in vitro gas production and nylon bay degradability of roughages in prediction feed intake in cattle. Anim Feed Sci Technol. 40:109–119. doi: 10.1016/0377-8401(93)90150-I
- Boucher SE, Ordway RS, Whitehouse NL, Lundy FP, Kononoff PJ, Schwab CG. 2007. Effect of incremental urea supplementation of a conventional corn silage-based diet on ruminal ammonia concentration and synthesis of microbial protein. J Dairy Sci. 90:5619–5633. doi: 10.3168/jds.2007-0012
- Bryant MP. 1973. Nutritional requirements of the predominant rumen cellulolytic bacteria. Federal Process Corporation. 32:1809–1813.
- Chauynarong N, Elangovan AV, Iji PA. 2009. The potential of cassava products in diets for poultry. World’s Poult Sci J. 65:23–36. doi: 10.1017/S0043933909000026
- Cherdthong A, Wanapat M, Wachirapakorn C. 2011. Influence of urea calcium mixture supplementation on ruminal fermentation characteristics of beef cattle fed on concentrates containing high levels of cassava chips and rice straw. Anim Feed Sci Technol. 163:43–51. doi: 10.1016/j.anifeedsci.2010.10.003
- Crichton N. 1999. Information point: Tukey multiple comparison test. J Clinical Nurs. 8:299–304.
- Ghimire A, Sen R, Annachhatre AP. 2015. Biosolid management options in cassava starch industries of Thailand: present practice and future possibilities. Procedia Chem. 14:66–75. doi: 10.1016/j.proche.2015.03.011
- Giang NTT, Wanapat M, Phesatcha K, Kang S. 2016. Level of Leucaena leucocephala silage feeding on intake, rumen fermentation and nutrient digestibility in dairy steers. Trop Anim Health Prod. 48:1057–1064. doi: 10.1007/s11250-016-1060-3
- Gollop N, Zakin V, Weinberg ZG. 2005. Antibacterial activity of lactic acid bacteria included in inoculants for silage and in silages treated with these inoculants. J Appl Microbiol. 98:662–666. doi: 10.1111/j.1365-2672.2004.02504.x
- Kang S, Wanapat M, Phesatcha K, Norrapoke T. 2015. Effect of protein level and urea in concentrate mixture on feed intake and rumen fermentation in swamp buffaloes fed rice straw-based diet. Trop Anim Health Prod. 47:671–679. doi: 10.1007/s11250-015-0777-8
- Khattab IM, Salem AZM, Abdel-Wahed AM, Kewan KZ. 2013. Effects of urea supplementation on nutrient digestibility, nitrogen utilization and rumen fermentation in sheep fed diets containing dates. Livest Sci. 155:223–229. doi: 10.1016/j.livsci.2013.05.024
- Ki KS, Khan MA, Lee WS, Lee HJ, Kim SB, Yang SH, Baek KS, Kim JG, Kim HS. 2009. Effect of replacing corn silage with whole crop rice silage in total mixed ration on intake, milk yield and its composition in Holsteins. Asian-Austral J Anim Sci. 22(4):516–519. doi: 10.5713/ajas.2009.80556
- Koike S, Kobayashi Y. 2001. Development and use of competitive PCR assays for the rumen cellulolytic bacteria: fibrobacter succinogenes, ruminococcus albus and ruminococcus flavefaciens. FEMS Microbiol Letters. 204:361–366. doi: 10.1111/j.1574-6968.2001.tb10911.x
- Kosugia A, Kondob A, Uedac M, Murataa Y, Vaithanomsatd P, Thanapased W, Araia T, Moria Y. 2009. Production of ethanol from cassava pulp via fermentation with a surface-engineered yeast strain displaying glucoamylase. Renewable Energy. 34(5):1354–1358. doi: 10.1016/j.renene.2008.09.002
- Lane DJ. 1991. 16S/23S rrna sequencing. In: Stackebrandt E, Goodfellow MD, editors. Nucleic acid techniques in bacterial systematics. New York, NY: John Wiley; p. 115–175.
- Lounglawan P, Khungaew M, Lounglawan W, Suksombat W. 2010. Utilization of cassava peel as energy source of silage. Proceeding of the 14th AAAP Animal Science Congress; Aug 23–27; Pingtung, Taiwan.
- Makkar HPS, Blummel M, Becker K. 1995. Formation of complexes between polyvinyl pyrrolidones or polyethylene glycols and tannins, and their implication in gas production and true digestibility in in vitro techniques. Bri J Nutr. 73:897–913. doi: 10.1079/BJN19950095
- Manish S, Banerjee R. 2008. Comparison of biohydrogen production processes. Int J Hydrog Energy. 33:279–286. doi: 10.1016/j.ijhydene.2007.07.026
- Menke KH, Raab L, Salewski A, Steingass H, Fritz D, Schneider W. 1979. The estimation of the digestibility and metabolizable energy content of ruminant feedstuffs from the gas production when they are incubated with rumen liquor in vitro. J Agri Sci (Camb). 93:217–222. doi: 10.1017/S0021859600086305
- Menke KH, Steingass H. 1988. Estimation of the energetic feed value obtained from chemical analysis and gas production using rumen fluid. Anim Res Dev. 28:7–55.
- Miller SM, Lennie G, Clelland D. 2005. Fortifying native pasture hay with molasses–urea mixtures improves its digestibility and nutrient intake by weaner sheep. Anim Feed Sci Technol. 119:259–270. doi: 10.1016/j.anifeedsci.2004.11.014
- Oji UI, Etim HE, Okoye FC. 2007. Effects of urea and aqueous ammonia treatment on the composition and nutritive value of maize residues. Small Rum Res. 69:232–236. doi: 10.1016/j.smallrumres.2006.01.015
- Ørskov ER, McDonal I. 1979. The estimation of protein degradability in the rumen from incubation measurements weighted according to rate of passage. J Agri Sci. 92:499–503. doi: 10.1017/S0021859600063048
- Russell JB, Muck RE, Weimer PJ. 2009. Quantitative analysis of cellulose degradation and growth of cellulolytic bacteria in the rumen. FEMS Microbiol Letters. 67:183–197. doi: 10.1111/j.1574-6941.2008.00633.x
- Salais FJ, Sutherland TM, Wilson A. 1977. Effect on animal performance of different sources of forage in diets based on molasses and urea. Trop Anim Health Prod. 2:158–162.
- SAS. 1996. Sas/STAT user’s guide: version 6.12. 4th ed. Cary, North Carolina: SAS Institute Inc.
- Silvestre R, MacLeod NA, Preston TR. 1977a. Voluntary intake and liveweight gain of cattle given chopped sugar cane and solutions of molasses containing different concentrations of urea. Trop Anim Health Prod. 2:1–12.
- Sriroth K, Chollakup R, Chotineeranat S, Piyachomkwan K, Christopher GO. 2000. Processing of cassava waste for improved biomass utilization. Bioresour Technol. 71:63–69. doi: 10.1016/S0960-8524(99)00051-6
- Sweeny JPA, Surridge V, Humphry PS, Pugh H, Mamo K. 2014. Benefits of different urea supplementation methods on the production performances of Merino sheep. Vet J. 200:398–403. doi: 10.1016/j.tvjl.2014.04.003
- Van Soest PJ, Robertson JB. 1985. Analysis of forage and fibrous feeds. a laboratory manual for animal science. Ithaca, NY: Department of Animal Science, Cornell University. 202.
- Van Soest PJ, Robertson JB, Lewis BA. 1991. Methods for dietary fiber neutral detergent fiber, and non-starch polysaccharides in relation to animal nutrition. J Dairy Sci. 74:3583–3597. doi: 10.3168/jds.S0022-0302(91)78551-2
- Wanapat M. 2003. Manipulation of Cassava cultivation and utilization to improve protein to energy biomass for livestock feeding in the tropics. Asian-Austral J Anim Sci. 16:463–472. doi: 10.5713/ajas.2003.463
- Wanapat M, Kang S. 2013. Enriching the nutritive value of cassava as feed to increase ruminant productivity. J Nut Ecol Food Res. 1:262–269.
- Wanapat M, Kang S, Khejornsart P, Pilajun R. 2013. Improvement of whole crop rice silage nutritive value and rumen degradability by molasses and urea supplementation. Trop Anim Health Prod. 45(5):1–5.
- Wanapat M, Kang S, Polyorach S. 2013. Development of feeding systems and strategies of supplementation to enhance rumen fer-mentation and ruminant production in the tropics. J Anim Sci Biotechnol. 4:32. doi:10.1186/2049-1891-4-32.
- Yu Z, Michel FC, Hansen G, Wittum T, Morrison M. 2005. Development and application of real-time PCR assays for quantification of genes encoding tetracycline resistance. Appl Environ Microbiol. 71: 6926–6933. doi: 10.1128/AEM.71.11.6926-6933.2005
- Yu Z, Morrison M. 2004. Improved extraction of PCR-quality community DNA from digesta and fecal samples. Bio Techniques. 36:808–812.