ABSTRACT
The fatty acid translocase (FAT/CD36) plays an important role in trans-membrane uptake of long-chain fatty acids (LCFA) and has been identified and described in many organisms, while little is known about this gene in freshwater fish, and especially in the grass carp (Ctenopharyngodon idellus). In this study, the uptake of LCFA in hepatocytes of C. idellus was firstly determined and then FAT/CD36 gene was cloned; then the gene was sequenced and mRNA levels from different tissues and the effect of dietary oils on its expression were determined. Present data showed that trans-membrane absorption of LCFA was related to the type and concentration of fatty acids (FAs) and it could be inhibited by specific membrane uptake inhibitors. The FAT/CD36 isolated from grass carp contained 1422 bp and the open reading frame was predicted to have 1403 bp (GenBank access number: KU361231.1). FAT/CD36 was highly expressed in the brain and its lowest expression was in the gills. FAT/CD36 expression in white muscle was up-regulated when fish were fed with groundnut oil, whereas it was down-regulated in fish fed fish and linseed oils, respectively. These results indicated n-3 PUFA might inhibit the FAT/CD36 mRNA expression and therefore the oxidation of FAs.
Abbreviations: Docosahexaenoic acid: DHA; Fatty acid: FA; Fatty acid translocase: FAT/CD36; Fish oil: FO; eicosapentaenoic acid: EPA; Groundnut oil: GO; Linseed oil: LO; Peroxisome proliferator-activated nuclear receptor: PPARs; Polyunsaturated fatty acid: PUFA; Unsaturated long-chain fatty acids: LCFA; Olive oil: OO
1. Introduction
Long-chain fatty acids (LCFAs) are nutrients associated with many important functions of the organism, as they (i) are a main fuel for cellular energy production and a reserve energy source, (ii) are part of phospholipids constituting biological membranes, (iii) may covalently be attached to specific proteins to regulate their function, and (iv) act in selected signal transduction events, (v) and (vi) act to modify gene expression. (Jump Citation2004; reviewed by Glatz and Luiken Citation2017). Initially, it was considered that LCFAs were taken up by cells through passive diffusion mechanisms (Kamp et al. Citation2003; Pownall and Hamilton Citation2003; Hamilton and Brunaldi Citation2007), while evidence has shown that LCFA uptake is also facilitated by a protein-mediated mechanism (Abumrad et al. Citation1999; Bonen et al. Citation2007; Guo et al. Citation2013). In this sense, the intracellular transport of free FAs in mammals is facilitated by specific low-molecular-weight and highly conserved cytoplasmic proteins that bind both long-chain FAs and other hydrophobic ligands. These fatty acid binding proteins (FABPs) are tissue specific and those from the liver, intestine, adipose tissue, brain and heart have been characterized extensively in mammals (Glatz and Luiken Citation2017). Similar studies have been reported in different fish species (Tocher Citation2003).
The fatty acid translocase (FAT/CD36), now officially being designated as the scavenger receptor B2 (SR-B2) (Prabhudas et al. Citation2014), is one of the most important FA transporters associated with LCFA trans-membrane uptake as it has been reported in humans (Luiken et al. Citation2016) and different teleost fish species (Torstensen et al. Citation2009; Zhou et al. Citation2010; Fink et al. Citation2015). The molecular and functional characterization of this protein has been described in zebra fish (Danio rerio), common carp (Cyprinus carpio) (Fink et al. Citation2015) and grass carp (Ctenopharyngodon idellus) (Tian et al. Citation2017). FAT/CD36-mediated FA transport into the skeletal muscle was reported to be critically coupled with muscle fuel selection and FA oxidation (McFarlan et al. Citation2012; Kim and Lim Citation2016), probably indicating that an increase in FAT/CD36 stimulated fat lipolysis and oxidation (Zhou et al. Citation2012). In mammals, FAT/CD36 (SR-B2) has been described to be implicated in lipid and FA disorders such as in the aetiology of obesity-induced or high fat diet-induced ectopic lipid accumulation and insulin resistance (Glatz et al. Citation2010; Liu et al. Citation2016; Glatz and Luiken Citation2017).
The grass carp is a herbivorous fish species and one of the most important freshwater carps cultured in China (Guo et al. Citation2015), as well as in other countries like Bangladesh, the Islamic Republic of Iran, the Lao People’s Democratic Republic, Myanmar and the Russian Federation among others (FAO Citation2017). However, one of the main problems related with the culture of this species is the accumulation of excessive fat intra-peritoneally and in the hepatopancreas tissue, which affects the health and quality of fish fed diets with high lipid levels (Guo et al. Citation2015). Regardless of the fact that the dietary lipid levels can be easily managed by aquafeed manufacturers, how LCFAs are taken up by the hepatocyte and whether the excessive fat accumulation may be correlated with an excessive uptake process or excessive amount of FAT/CD36 are not yet completely clear. In this regard, grass carp are generally fed with many types of dietary oil sources, depending on the cost of the feed, without any detrimental effect on growth performance (Cao et al. Citation1996). The inclusion of highly unsaturated fatty acids (HUFAs), including arachidonic acid (ARA), eicosapentaenoic acid (EPA) and docosahexaenoic acid (DHA) inclusion, was reported to suppress lipid accumulation in this species (Tian et al. Citation2014; Lei et al. Citation2016); thus, authors aimed to test whether the excessive fat accumulation normally found in grass carp could be suppressed by dietary oils through the regulation of FAT/CD36 expression.
The molecular dynamics of LCFAs’ uptake and of LCFAs on FA absorption and their impact on lipid accumulation was studied in C. idellus. For this purpose, the uptake of LCFAs in the hepatic tissue through the activity of FAT/CD36, as well as the cloning, specific-tissue expression and mRNA expression of FAT/CD36 in response to different dietary oil sources were analysed in this study.
2. Materials and methods
2.1. Trans-membrane uptake of LCFA in hepatocyte of grass carp
2.1.1. Preparation of hepatocytes
Healthy C. idellus (body weight, BW = 460 ± 100 g) were purchased from Kang Le fish market (Yangling, Shanxi Province, China) and transported to the laboratory. Once there, fish were sedated with 0.1 g L−1 metacain (MS222, Sigma-Aldrich), and the hepatopancreas (2–3 g) removed. The protocol described by Yu et al. (Citation2003) was followed for obtaining primary cell cultures. In particular, small pieces of liver were washed in a PBS solution three times, then the tissues were digested with 20 mL PBS buffer (8 g NaCl L−1, 0.2 g KCl L−1, 1.44 g Na2HPO4 L−1, 0.24 g KH2PO4 L−1) containing collagenase (0.1 mg mL−1). Then, the digested hepatopancreatic tissue was gently filtered through a 100 μm nylon gauze twice; cells were then collected by centrifugation (50 g for 5 min at 4°C, three times) and washed after each centrifugation. Finally, cells were re-suspended in 10 mL complete dulbecco's modified eagle medium (DMEM) medium. The cell viability was routinely determined by the trypan blue excluded method and cells with viability values >85% were considered for further use. Cells were diluted into a density of 5–8 × 105 cells mL−1 and 100 μL cell solution were put into each well of a 96-well plate (BD Falcon 96-well Flat-bottom ELISA PLATES from BD Biosciences-Discovery Labware) and cultured at 20°C for 8 h before in vitro assays.
2.1.2. LCFAs’ uptake-time course study by QBT kit
Firstly, working solutions of the QBT kit (QBt Fatty Acid uptake Assay Kit, Molecular Devices, Inc.) were prepared and then 100 μL of the working solution was added into each well containing hepatocytes and incubated for 5, 10, 15, 25, 35, 45 min, and the relative fluorescence units (RFUs; λ = 515 nm) were read by micro-plate reader (TECAN M200pro, Switzerland) with 515 nm exciting light. shows two images of hepatocytes’ primary cell cultures incubating with and without QBT.
2.1.3. LCFA uptake depending on oleic acid (OA) incubating time and concentration
The aim of the present experiment was to measure the LCFA uptake depending on OA incubating time and concentration in order to find the proper time and OA concentration to show specificity of the LCFA uptake process in C. idellus. A volume of 75 μM OA, dissolved into 0.05% dimethyl sulfoxide (DMSO)-complete DMEM medium, was incubated for 0, 0.5, 1, 2 and 4 h as described by Liao et al. (Citation2005); then, 0.1 mL QBT working solution was added into each well for 30 s and the RFUs were read by micro-plate reader, as previously indicated. Each condition (incubation time) had six methodological replicates.
In order to evaluate the optimal OA concentration, 0, 37.5, 75, 150, 300 and 600 μM OA, previously dissolved into 0.05% DMSO-complete DMEM medium, respectively, were incubated with cells for 1 h (six replicates per OA concentration). Then, 0.1 mL QBT working solution was added into each well and the RFUs determined after 30 s.
2.1.4. LCFA uptake
Five different LCFAs (OA, LA (18:2n-6), ALA (18:3n-3), EPA and DHA) were used for testing their uptake in primary hepatocytes’ cell culture conditions. For this purpose, 75 μM of OA, LA, ALA, EPA and DHA (Fluka Chemicals, Sigma, USA) were initially dissolved into 0.05% DMSO-complete DMEM medium and incubated with cells for 1 h (six replications per LCFA). Then, 0.1 ml QBT working solution was added into each well and fluorescence read after 30 s as previously described. Cells without LCFA incubation were used as control groups.
2.1.5. Inhibition of FA uptake
Hepatocytes were pre-incubated with specific FA uptake inhibitors according to Zhou et al. (Citation2010): 250 μM phloretin, 250 μM 4,4'-Diisothiocyanatostilbene-2,2'-disulfonic acid, disodium salt (DIDS), 200 μM Sulfosuccinimidyl 4-(N-maleimidomethyl) cyclohexane-1-carboxylate (SSM) and 10 mM cyclodextrin (Fluka Chemicals) were dissolved in 0.05% DMSO-complete DMEM medium for 0.5 h. Then, cells were incubated with QBT working solution kit and the RFUs read as previously described. Cells without the specific inhibitors were used as control groups. Each treatment had six methodological replicates.
2.2. Cloning and sequence analysis of FAT/CD36 gene in C. idellus
Six C. idellus (BW = 500 ± 16 g) were collected from the local fish supermarket of Cheng Du (Si Chuan Province, China) and were gently anesthetized with metacain (0.1 g L−1). The hepatopancreas was removed and total RNA extracted using TRIzol reagent (Invitrogen, Carlsbad, CA, USA) according to the manufacturer’s instructions. RNA was quantified using a Gene-Quant spectrophotometer (Amersham Biosciences) and purity established by the absorbance ratio 260/280 nm. The integrity of the RNA was examined by gel electrophoresis. The cDNA was synthesized by Revert Aid First Strand cDNA Synthesis Kit (Fermentas Life Science, Hanover, MD, USA). The 25 μL reaction volume was composed of 2 × Long Taq PCR Master Mix 12.5 μL, forward primer (10 μmol L−1) 1.0 μL, reverse primer (10 μmol L−1) 1.0 μL, total RNA 1.0 μL and 9.5 μL ddH2O. Real-time qPCR was performed using a fluorescence temperature cycler (Bio-Rad, Hercules, CA, USA) and the qPCR programme started at 95°C for 4 min to pre-denature the cDNA, then it was followed by 40 cycles of denaturation at 94°C for 30 s, annealing at 60°C for 90 s and extension at 72°C for 1 min with a final extension at 72°C for 10 min. A final dissociation reaction (melting curve) was performed (95°C for 15 s, 60°C for 20 s, 95°C for 15 s and 60°C for 15 s) to evaluate the specificity of the amplicon. The PCR fragments were separated by 1% agarose gel electrophoresis, and then purified for cloning and subsequent sequencing. The sequences of FAT/CD36 gene primers were referred to that of common carp (Cyprinus carpio), whose GenBank accession number was KM 030422 (, Fink et al. Citation2015).
Table 1. Primer sequence for molecular cloning and quantitative real-time PCR of FAT/CD36 in grass carp (C. idellus). All sequences are presented as 5′-3′.
Fragments of interest were cloned into pMD19-T vector (TaKaRa, Dalian, China) and transformed into Escherichia coli DH5α. For each fragment, five clones were selected and sequenced by Qing Ke Zhi Xin Biotechnology Co. Ltd. (Cheng Du, Si Chuan Province, China). This sequence was used to predict the amino acid sequence, isoelectric point and molecular weight of FAT/CD36 by ExPASy-Tools (http://www.expasy.org/tools). Putative protein domains were characterized by lnterproscan (http://www.ebi.ac.uklinterpro/). Signal peptides were predicted by SignalP 4.0 (http://www.cbs.dtu.dk/services/SignalP/) (Petersen et al. Citation2011). The amino acid sequence homology was identified by BioEdit software version 5.0.6 (Hall Citation2001). Phylogenetic analysis of FAT/CD36 among C. idellus, common carp (accession number: KM030422), zebra fish (NM_001002363), Homo sapiens (KR710356) and Macaca mulatta (AY600441) has been used to study the evolutionary relationship by amino acid sequences extensively.
2.3. Analysis of FAT/CD36 mRNA levels in different tissues of C. idellus by qPCR
Different tissues, including the heart, liver, red and white muscle, intraperitoneal fat tissue, hepatopancreas, spleen, kidney, intestine and brain were sampled from six fish (BW = 500 ± 16 g); then total RNA of these tissues were extracted using TRIzol reagent (Invitrogen, Carlsbad, CA, USA) and treated with Rnase-free DNase in order to remove genomic DNA. RNA integrity and quality were determined by means of agarose gel electrophoresis and spectrophotometry (NanoDrop 1000, Thermo Scientific, Wilmington, USA); then RNA (1 μg) was reverse-transcribed into cDNA by PrimeScript® RT reagent kit (TaKaRa, Dalian, China), respectively. The volume of amplification mixture for Real-Time PCR contained total RNA 1 μL, SYBR® Premix Ex Taq TM (2×) (TaKaRa, Dalian, China) 10 μL, primers (10 μmol/L) 0.5 μL and ddH2O 8.5 μL. The threshold cycle (CT) was analysed using the 2−ΔΔCt method (Livak and Schmittgen Citation2001). Real-time analysis was performed by a fluorescence temperature cycler (Bio-Rad, Hercules, CA, USA) according to the following parameters: pre-denaturation at 95°C for 1 min, followed by 40 cycles of denaturation at 95°C for 30 s, annealing at 54–63.5°C for 30 s and then extension at 72°C for 30 s. Primers for Real-Time PCR are given in .
2.4. Effect of different dietary oil sources on the relative expression of FAT/CD36
2.4.1. Preparation of experimental diets
Four different dietary oil sources, linseed oil (LO), groundnut oil (GO), olive oil (OO) and fish oil (FO), were added into a basal diet for C. idellus. Ingredient list and proximate composition of experimental diets are shown in . Feed ingredients were purchased from Huaqin Husbandry and Technology Co., Ltd. (Yangling, Shaanxi, China). Diets were prepared by mixing all dry ingredients for 30 min; then four oils and sufficient distilled water were added respectively to form soft mixtures that were mechanically extruded to obtain pellets of ca. 2 mm in size. Pellets were air-dried at 25°C and stored in re-sealable plastic bags at −20°C until use.
Table 2. Composition and nutrient levels of the experimental diets (air-dry basis, %) for grass carp (C. idellus) used in the in vivo study.
Lipids from these 4 diets were extracted by homogenization using the chloroform:methanol (2:1 v/v) method as previously described (Folch et al. Citation1957). FAs were saponified and methylated using 12% boron trifluoride (BF3) in methanol and analysed by gas chromatography using the methods described previously by Lie and Lambertsen (Citation1991). The gas chromatograph (HP model 6890) was equipped with a carbowax capillary column and a flame ionization detector, with a 50-m CP-sil 88 (Chromopack) fused silica capillary column (i.d., 0.32 mm). Injection and detection temperatures were 250°C, nitrogen was the carrier gas and the column temperature was programmed to rise from 100°C to 220°C at a rate of 25°C/min. The FAs were identified by retention time using standard mixtures of methyl esters (Nu-Chek-Prep) and quantified using Totalchrom software (version 6.2, Perkin Elmer). The FA composition of the diets is shown in .
Table 3. FA composition of diets (% of total FA) used in the in vivo study with grass carp (C. idellus).
2.4.2. Feeding and management of fish
A total of 80 juvenile grass carps (BW = 56.9 ± 4.2 g) were divided into 4 groups, 20 fish per group and were reared in 4 indoor tanks (112 cm × 34 cm × 54 cm) and fed with the above-mentioned experimental diets (feeding rate: 3%). Fish were fed by hand 3 times per day (8:30, 12:30 and 16:30). Water in each tank was aerated 24 h per day with airstones and 2/3 of the water in the tank was exchanged with fresh water every morning. The water temperature and dissolved oxygen were 22–25°C and 5–8 mg L−1, respectively. The nutritional trial lasted for 60 days. Fish BW was measured every 20 days in order to adjust feeding rates with grass carp somatic growth.
2.4.3. Sampling procedures and FAT/CD36 expression in white muscle by qPCR
At the end of the feeding trial, fish were gently anesthetized with metacain (0.1 g L-1) and their final body weight (BWf) measured to the nearest 0.1 g. Then, eight fish per tank were slaughtered and their white muscles were sampled. Total RNA was extracted from white muscle and the expression of FAT/CD36 in this tissues was determined by qPCR analysis as previously described.
2.5. Statistical analysis
Data are shown as mean ± standard deviation (SD). Statistical differences were analysed by one way ANOVA, followed by a Tukey’s post-hoc test when statistically significant differences were found between experimental groups. All data were checked for normality (Kolmogorov–Smirnov test) and homoscedasticity of variance (Bartlett’s test) prior to the ANOVA test. All statistical analyses were performed using SPSS13.0 for Windows Software (SPSS, Chicago, IL, USA). Results were considered significant at P < .05.
3. Results
3.1. Trans-membrane uptake of LCFA in grass carp hepatocytes
3.1.1. LCFA uptake-time course study
LCFAs’ uptake-time course study showed that the QBT was progressively taken up by hepatocytes between 5 and 45 min, whereas it gradually reached a steady state (uptake saturation) after 20 min. The relationship between LFCA uptake and time was linear and represented by the following curve: y = 1724.2 ln(x) + 16533 (R2 = 0.9065), where x corresponds to the incubation and y the RFUs; (). As the uptake of QBT increased very fast, the RFU determination using the QBT could be done within 5–15 min of incubation time.
3.1.2. Establishing optimal LCFA uptake depending on experimental conditions
Uptake of OA by hepatocytes progressively increased with increasing OA concentration as it is shown in (P < .05). Considering these results and the FA concentration in fish blood the concentration of 75 μM was chosen for the following experiments. The OA uptake kinetics in hepatocytes is shown in . Results indicated that LCFA uptake with incubating hepatocytes with 75 μM OA was maximum during the first hour, whereas it decreased later on at 2 and 4 h post-incubation (P < .05). Thus, incubation time was set up at 1 hour for further in vitro trials. LCFA uptake varied depending on the FA considered. The highest absorption rates (RFUs) were observed when hepatocytes were incubated with 18:3n-3 and 8:2n-6, followed by 18:1n-9 and 20:5n-3, whereas the lowest uptake was observed when hepatocytes were incubated with 22:6n-3 (; P < .05).
3.1.3. Modulation of LCFAs’ uptake by specific inhibitors
To clarify whether LCFAs’ uptake into hepatocytes of grass carp was mediated by membrane proteins, the effects of several membrane protein inhibitors (phloretin, DIDS, SSM and cyclodextrin) on FA uptake was studied (). The exposure of hepatocytes to SSM and phloretin resulted in the highest inhibition of LCFAs’ uptake (14.0 ± 10.3% and 10.19 ± 6.4%, respectively) compared to the control group (P < .05). DIDS showed lower inhibition levels (3.8 ± 2.5%), whereas cyclodextrin showed no inhibition on LCFAs’ uptake, since values of RFUs were similar in comparison to the control group (P > .05).
3.2. Cloning and characterization of FAT/CD36 gene in C. idellus
The FAT/CD36 cDNA of C. idellus, being cloned and sequenced, was found to be composed of 1.422 nucleotides with an open reading frame (ORF) of 1403 nucleotides. This ORF was postulated to encode a protein with 468 amino acids and a molecular weight of 52.47 kDa. This gene sequence was submitted to GenBank and the accession number was KU361231.1.
The multiple sequence alignments showed that the amino acid sequence of FAT/CD36 in C. idellus had a very high identity with that of other cyprinid species like Cyprinus carpio and Danio rerio, with similarity values of 82% and 76% respectively (). By further analysis of the post-translational modifications, it was postulated that there were 6 serine phosphorylation sites, 7 threonine phosphorylation sites, 3 tyrosine phosphorylation sites, 2 oxygen-glycosylation sites and 7 nitrogen-glycosylation sites along the gene sequence ().
Figure 7. Comparison of FAT/CD36 amino acids’ homology in grass carp (C. idellus), common carp (C. carpio) and zebrafish (D. rerio). The line of consensus shows the conserved residues of FAT/CD36 among these fish and these identical amino acid residues are shown in white words with black background. The trans-membrane domain of FAT/CD36 is boxed. The alignment was generated using vector DNAMAN software.
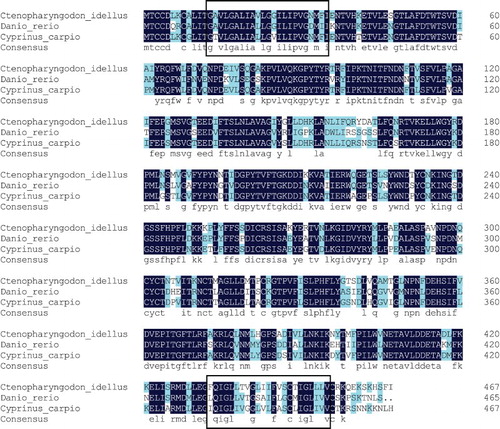
The secondary protein structure of FAT/CD36 suggested that this protein contains139 amino acids (blue line domain), 109 amino acids (red line domain) and 220 amino acids (purple line domain), occupying 29.7%, 23.3% and 47.0% of total amino acids, respectively, which may be attributed to be α-helices, β-brands and random coils, respectively (). Two trans-membrane domains were postulated to be located in this protein without a messenger peptide structure ().
Figure 9. Secondary structure of FAT/CD36 protein is predicted. The domain of amino acids in blue line are α-helices, the domain of amino acids in red line are β-brands and the amino acids left in purple line are random coils.

Figure 10. Three-dimensional model of FAT/CD36 shown by cartoon and surface models. This protein included many α-helices, β-brands and random coils. It was generafted by PHYRE2 Server.
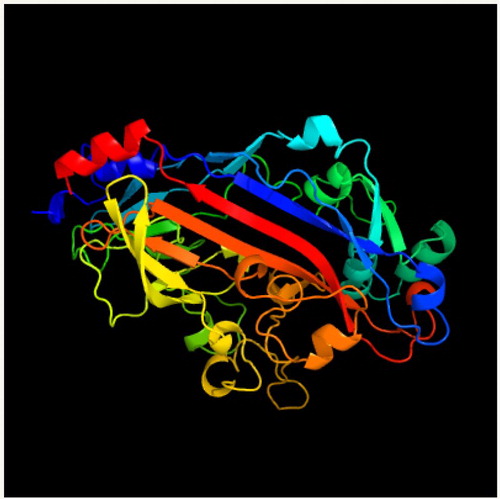
The phylogenetic analysis based on the protein sequences of FAT/CD36 showed that the genetic relationship between C. idellus, C. carpio and D. rerio was very similar (99% similarity; ). However, the genetic relationship between grass carp and higher vertebrates like Homo sapiens or Macaca mulatta was very different, where the homology of ITS was less than 87% comparing their ITS sequences of FAT/CD36 ().
Figure 11. Phylogenetic analysis of FAT/CD36. Phylogenetic tree based on protein sequences was constructed by the neighbour-joining method with Mega 5.0 software. The strength of branch relationships was assessed by bootstrap replication (N = 1000 replicates). Grass carp FAT/CD36 was indicated by ‘●’. Accession numbers of protein sequences for FAT/CD36 are as follows: Cyprinus carpio (KM030422); Danio rerio (NM_001002363); Homo sapiens (KR710356); Macaca mulatta (AY600441); Rattus norvegicus (NM_031561); Mus musculus (NM_001159558); Lipotes vexillifer (XM_007467039); Bos taurus (NM_001278621); Capra hircus (JF690773); Gallus gallus (NM_001030731); Xenopus (Silurana) tropicalis (NM_001113679).
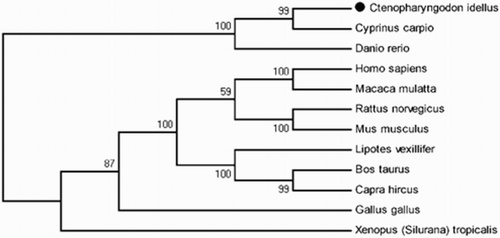
3.3. Gene expression patterns of FAT/CD36 in C. idellus
Among the 10 different tissues where FAT/CD36 was expressed, the brain and hepatopancreas were the organs where this gene was mainly expressed, whereas the lowest expression values were found in the gills (; P < .05). Diets containing different types of oils statistically affected the expression of FAT/CD36 in the white muscle of C. idellus (; P < .05). The highest mRNA levels of FAT/CD36 in the white muscle were found in grass carp fed the diet containing GO, followed by those measured in fish fed the diet containing OO, whereas fish fed the LO and FO diets showed the lowest levels in FAT/CD36 gene expression.
4. Discussion
4.1. LCFA trans-membrane uptake in the hepatocytes of C. idellus
Hepatocytes of grass carp are an established in vitro system that has been used in a range of lipid metabolic studies (Lu et al. Citation2012). The present study is the first one focused on trying to elucidate the mechanisms underlying the LCFAs update in C. idellus hepatocytes. Traditionally, the experimental approaches used for studying the FA uptake in cells and their metabolism were based on the use of a 14C labelled FA and then measuring the intracellular levels of radioactivity (Torstensen and Stubhaug Citation2004; Stubhaug et al. Citation2005; Vegusdal et al. Citation2005; Zhou et al. Citation2010). The quantification of FA uptake using fluorescence by means of the QBT kit, where QBT is a kind of LCFA being fluorescently labelled, is a quite recent and environmental-friendly procedure and a safer protocol from the point of view of the user (Liao et al. Citation2005). In the present study, QBT was efficiently taken up by grass carp hepatocytes of grass carp, being highest during the first 45 min post LCFA incubation, whereas this uptake occurred through a saturable transport mechanism (Sorrentino et al. Citation1989), which was in line with the results reported in other Teleost species like in rainbow trout (Richards et al. Citation2003), Atlantic salmon (Zhou et al. Citation2010) as well as in different tissues from higher vertebrates [i.e. skeletal muscle (Turcotte et al. Citation1991), mammalian adipocytes (Abumrad et al. Citation1981; Schwieterman et al. Citation1988), cardiac myocytes (Sorrentino et al. Citation1988) and hepatocytes (Stremmel and Berk Citation1986)]. Under current experimental conditions, LCFA uptake was stimulated in hepatocytes of C. idellus when incubated 18:2n-6 and 18:3n-3, while it was suppressed in 22:6n-3. These results differed with those already reported in Atlantic salmon (Stubhaug et al. Citation2005; Zhou et al. Citation2010), where 20:5n-3 and 22:6n-3 were predominantly taken up by hepatocytes in comparison to 18-C FAs. Such differences between both studies may be attributed to the different essential FA requirements between both studies. The grass carp is a warm water fish species, whereas the main essential FAs for this species are 18:2n-6 and 18:3n-3 (Takeuchi et al. Citation1991) while the Atlantic salmon is mainly a marine fish species with important requirements in n-3 HUFA, especially EPA and DHA (NRC Citation1993). In general terms, the membrane proteins, FABPpm, FAT/CD36 and fatty acid transport protein (FATP), are responsible for the cellular uptake of LCFAs (Frohnert and Bernlohr Citation2000) and phloretin was reported to be a potent non-specific inhibitor of membrane transport proteins (Lefevre Citation1975; Andersen et al. Citation1978). This meant that the buffer containing this inhibitor would suppress the efflux or influx of FAs across the cell membrane by blocking the activity of these proteins (Abumrad et al. Citation1981). In this study, LCFAs’ uptake was differently inhibited by the five inhibitors tested, although any of the tested inhibitors resulted in a complete inhibition of LCFA uptake across the hepatocytes’ membrane. In particular, hepatocytes exposed to phloretin showed 10.1 ± 6.4% inhibition compared with the control, whereas DIDS and SSM inhibited LCFA transport by 3.8 ± 2.5% and 13.9 ± 10.3%, respectively. These values were lower than those reported earlier in Atlantic salmon and rainbow trout, where LCFA uptake was inhibited by 28% in hepatocytes (Zhou et al. Citation2010) and up to 40% in muscle sarcolemma (Richards et al. Citation2003), respectively. In contrast, inhibition values were higher in higher vertebrates (rodents) and ranged from 60% to 9% in cardiac myocytes (DeGrella and Light Citation1980; Stremmel Citation1988; Luiken et al. Citation1997) and adipocytes (Abumrad et al. Citation1984; Harmon et al. Citation1991). These discrepancies between different studies may suggest that the mechanisms of LCFAs’ uptake in the hepatocytes of C. idellus grass might be not so reliable on a protein-mediated transport compared to other fish and mammalian cell lines. In addition, the inhibitor of cyclodextrin was previously reported to disturb the lipid raft by lowering the cholesterol levels in the lipid raft (Ikonen Citation2001), and no QBT uptake inhibition by cyclodextrin was found in the present study, being in line with previous results in hepatocytes of Atlantic salmon (Zhou et al. Citation2010), probably indicating that lipid raft was not included in FA uptake in hepatocytes of grass carp or the contribution of lipid raft to FA uptake was little. More research is required to explore the mechanisms of LCFAs’ uptake by lipid raft in grass carp.
4.2. Molecular cloning of FAT/CD36 in C. idellus
Characterization of FAT/CD36 in the hepatopancreas of grass carp showed that FAT/CD36 is an integral membrane glycoprotein with two trans-membrane domains, a large extracellular loop and two short intracellular tails. This topology is similar to the mammalian CD36 (Febbraio et al. Citation2001) as well as the CD36 described in common carp and zebrafish (Fink et al. Citation2015). Although the grass carp FAT/CD36 sequence seems relatively similar to that of mammalians, common carp and zebrafish CD36, there were a number of intriguing differences among them. Contrary to the findings in the human, common carp and zebrafish CD36 proteins that they did not have O-glycosylation sites (Febbraio et al. Citation2001; Fink et al. Citation2015), there were two predicted O-glycosylation sites in FAT/CD36 of grass carp. However, the significance of these potential O-glycosylation sites in FAT/CD36 in fish is not yet known (Fink et al. Citation2015). Grass carp FAT/CD36 was predicted to be a 52.47 kDa protein, which was similar to the molecular weight of this protein described in pigeons (52.7 kDa; Xie et al. Citation2012), while it had a lower molecular weight than in rats (88 kDa; Abumrad et al. Citation1993; Baillie et al. Citation1996). These differences may be attributed to species-specific differences rather than to their functional properties.
4.3. Tissue distribution of FAT/CD36 measured by qPCR in C. idellus
The amount of FAT/CD36 mRNA in different tissues may be attributed to its role of facilitating FA transport in tissues with a high capacity for FA metabolism, which may fluctuate depending on developmental, hormonal and environmental conditions (Silverstein and Febbraio Citation2009). In grass carp, FAT/CD36 was expressed in all analysed tissues, which was in accordance with previous results in mammalians where FAT/CD36 was expressed in the skeletal and smooth muscle, the intestinal epithelium, adipocytes, endothelial cells, platelets, dendritic cells, and monocytes and macrophages (Febbraio et al. Citation2001). Results from the qPCR analyses indicated that the expression of this gene in the brain and the hepatopancreas was the highest in comparison to the other studied tissues; results that were in accordance with previous results in common carp and zebrafish (Fink et al. Citation2015) by their higher requirements of FAs due to specific-tissular metabolism. The lowest FAT/CD36 gene expression was found in the spleen and gill, regardless of their importance as tissues involved in the immune defence of the organism (Han et al. Citation2010). Similar results were recently reported by Tian et al. (Citation2017), who showed that levels ciCD36 mRNA were absent in the kidney of grass carp. In mammals, it has been reported that FAT/CD36 (SR-B2) expression in the liver was absent or very low (Febbraio et al. Citation1999), while in the present study FAT/CD36 expression was relatively high in the hepatopancreas of grass carp, probably indicating an important role of this protein in the cellular FA uptake in the hepatocytes of grass carp, which may be different from mammals.
4.4. FAT/CD36 mRNA expression responses to different dietary oil sources in C. idellus
The effect of different dietary oil sources and/or FAs on their cellular uptake and accumulation has received a lot of attention during the last decades. In the hepatopancreas of Atlantic salmon, the expression of FAT/CD36 was not affected by vegetable or FOs (Zhou et al. Citation2014), while in mammals, unsaturated LCFAs significantly increased FAT/CD36 mRNA levels in macrophages and ventricular myocytes (van der Lee et al. Citation2000; Vallvé et al. Citation2002), the small intestine, preadipocytes and neonatal cardiomiocytes (Chen et al. Citation2001), mature adipose cells (Nisoli et al. Citation2000) and abdominal fat of hypertensive rats (Aguilera et al. Citation2006). Furthermore, Yang et al. (Citation2007) showed that FAs with a chain length longer than eight carbons could effectively inhibit FAT/CD36 expression in adipocytes, but unsaturated LCFAS had no effect. Dietary oil sources, such as lard oil, FO or sunflower-seed oil, differently influenced FAT/CD36 expression in the liver and muscle of rats (Feillet-Coudray et al. Citation2013) and in the mammary secretory tissue and mental adipose tissue of goats (Toral et al. Citation2013), whereas FAT/CD36 expression in the hepatopancreas was not affected by diets containing lard oil diet or FO in grass carp (Tian et al. Citation2015). These previous studies give scarcely identical information on different long-chain unsaturated FAs affecting FAT/CD36 expression.
In the present study, the highest FAT/CD36 expression in the white muscle was found in the GO group, a diet rich in 18:2n-6, whereas the lowest FAT/CD36 expression levels were found in fish fed the LO and FO diets, which contained high contents of 18:3n-3, 20:5n-3 and 22:6n-3. These results indicated that n-3 PUFAs inhibited FAT/CD36 expression in the white muscle, and therefore reduced or even suppressed LCFA uptake in this tissue, which was in agreement with the above-mentioned results from the in vitro study conducted in hepatocytes from grass carp. These findings are of special significance when formulating compound diets for grass carp, since selecting the proper type of oil source with a particular FA profile may be of interest for reducing the adiposity in this herbivorous species, once the nutritional requirements are met in terms of growth performance and health condition. Nevertheless, further research is required to determine the molecular mechanisms of LCFAs’ uptake and how LCFAs regulate the activity of transport membrane proteins in grass carp.
In conclusion, results from the in vitro studies revealed that the uptake of FAs by grass carp hepatocytes was shown to be partly membrane protein associated, since LCFAs were found to be absorbed by cells regardless of the transport protein inhibitor tested. FAT/CD36 gene in grass carp has high homology with other fish, especially cyprinid species, and it is primarily expressed in the brain and in the hepatopancreas, whereas its lower expression levels were found in the gill and spleen, differences that may be attributed to tissue-specific differences in FA requirements and metabolism. Dietary oil sources, containing different LCFAs, have a significant impact on FAT/CD36 expression in the white muscle of grass carp, where mRNA levels were highest in fish fed the GO diet, being rich in 18:2n-6, and lowest in fish fed the LO and GO diets that were rich in 18:3n-3, 20:5n-3 and 22:6n-3, showing a regulatory role of FAs on FATA/CD36 expression and FA absorption in cells.
Acknowledgements
Thanks are due to Xiao Ling Chen and XiaoYan Chen for their help in sampling and preparing the cells.
Disclosure statement
No potential conflict of interest was reported by the authors.
Additional information
Funding
Notes
* The research was conducted in both ‘College of Animal Science and Technology, Northwest A&F University, Yangling, Shaanxi 712100, People’s Republic of China’ and ‘College of Life Science and Technology, Southwest University for Nationalities, Chengdu 610041, People’s Republic of China’.
References
- Abumrad N, Coburn C, Ibrahimi A. 1999. Membrane proteins implicated in longchain fatty acid uptake by mammalian cells: CD36, FATP and FABPm. Biochim Biophys Acta. 1441:4–13. doi: 10.1016/S1388-1981(99)00137-7
- Abumrad NA, Elmaghrabi MR, Amri EZ, Lopez E, Grimaldi PA. 1993. Cloning of a rat adipocyte membrane-protein implicated in binding or transport of long-chain fatty-acids that is induced during preadipocyte differentiation-homology with human CD36. J Biol Chem. 268:17665–17668.
- Abumrad NA, Park JH, Park CR. 1984. Permeation of long-chain fatty acid into adipocytes. J Biol Chem. 259:8945–8953.
- Abumrad NA, Perkins RC, Park JH, Park CR. 1981. Mechanism of long chain fatty acid permeation in the isolated adipocyte. J Biol Chem. 256:9183–9191.
- Aguilera AA, Díaz GH, Barcelata ML, Guerrero OA, Ros RMO. 2006. Induction of Cd36 expression elicited by fish oil PUFA in spontaneously hypertensive rats. J Nutr Biochem. 17:760–765. doi: 10.1016/j.jnutbio.2005.12.007
- Andersen BL, Tarpley HL, Regen DM. 1978. Characterization of β-hydroxybutyrate transport in rat erythrocytes and thymocytes. Biochim Biophys Acta. 508:525–538. doi: 10.1016/0005-2736(78)90097-4
- Baillie AGS, Coburn CT, Abumrad NA. 1996. Reversible binding of long-chain fatty acids to purified FAT, the adipose CD36 homolog. J Membr Biol. 153:75–81. doi: 10.1007/s002329900111
- Bonen A, Chabowski A, Luiken J, Glatz JFC. 2007. Is membrane transport of FFA mediated by lipid, protein, or both? Mechanisms and regulation of proteinmediated cellular fatty acid uptake: molecular, biochemical, and physiological evidence. Physiology. 22:15–29.
- Cao JM, Tian LX, Chen Z, Liu YJ, Liang GY. 1996. Effect of dietary fatty acids on growth and tissue chemical composition of grass carp, Ctenopharyngodon idellus. J South China Univ Techn (Natural Science). 24(Suppl.):149–154. (in Chinese).
- Chen M, Yang Y, Braunstein E, Georgeson KE, Harmon CM. 2001. Gut expression and regulation of FAT/CD36: possible role in fatty acid transport in rat enterocytes. Am J Physiol Endocrinol Metab. 281:E916–E923.
- DeGrella RF, Light RJ. 1980. Uptake and metabolism of fatty acids by dispersed adult rat heart myocytes. J Biol Chem. 255:9739–9745.
- FAO. 2017. Ctenopharyngodon idella (Valenciennes, 1844). In: Cultured Aquatic Species Information Programme, Fisheries and Aquaculture Department; [accessed: 2017 July 4]. http://www.fao.org/fishery/culturedspecies/Ctenopharyngodon_idellus/en.
- Febbraio M, Abumrad NA, Hajjar DP, Sharma K, Cheng W, Frieda S, Pearce A, Silverstein RL. 1999. A null mutation in murice CD36 reveals an important role in fatty acid and lipoprotein metabolism. J Biol Chem. 274:19055–19062. doi: 10.1074/jbc.274.27.19055
- Febbraio M, Hajjar DP, Silverstein RL. 2001. CD36: a class B scavenger receptor involved in angiogenesis, atherosclerosis, inflammation, and lipid metabolism. J Clin Invest. 108:785–791. doi: 10.1172/JCI14006
- Feillet-Coudray C, Aoun M, Fouret G, Bonafos B, Ramos J, Casas F, Cristol JP, Coudray C. 2013. Effects of long-term administration of saturated and n-3 fatty acid-rich diets on lipid utilisation and oxidative stress in rat liver and muscle tissues. Br J Nutr. 110:1789–1802. doi: 10.1017/S0007114513001311
- Fink IR, Benard EL, Hermsen T, Meijer AH, Forlenza M, Wiegertjes GF. 2015. Molecular and functional characterization of the scavenger receptor CD36 in zebrafish and common carp. Mol Immunol. 63(2):381–393. doi: 10.1016/j.molimm.2014.09.010
- Folch M, Lees GH, Stanley S. 1957. A simple method for the isolation and purification of total lipids from animal tissues. J Biol Chem. 226:497–509.
- Frohnert BI, Bernlohr DA. 2000. Regulation of fatty acid transporters in mammalian cells. Prog Lipid Res. 39:83–107. doi: 10.1016/S0163-7827(99)00018-1
- Glatz JFC, Luiken JJFP. 2017. From fat to FAT (CD36/SR-B2): understanding the regulation of cellular fatty acid uptake. Biochimie. 136:21–26. doi: 10.1016/j.biochi.2016.12.007
- Glatz JFC, Luiken JJFP, Bonen A. 2010. Membrane fatty acid transporters as regulators of lipid metabolism: implications for metabolic disease. Physiol Rev. 90:367–417. doi: 10.1152/physrev.00003.2009
- Guo X, Liang XF, Fang L, Yuan X, Zhou Y, Zhang J, Li B. 2015. Effects of dietary non-protein energy source levels on growth performance, body composition and lipid metabolism in herbivorous grass carp (ctenopharyngodon idella Val.). Aquac Res. 46:1197–1208. doi: 10.1111/are.12275
- Guo J, Shu G, Zhou L, Zhu X, Liao W, Wang S, Yang J, Zhou G, Xi Q, Gao P, et al. 2013. Selective transport of long-chain fatty acids by FAT/CD36 in skeletal muscle of broilers. Animal. 7(3):422–429. doi: 10.1017/S1751731112001619
- Hall T. 2001. Bioedit version 5.0.6. Department of Microbiology, North Carolina State University. http://www.mbio.ncsu.edu/BioEdit/bioedit.html.
- Hamilton JA, Brunaldi K. 2007. A model for fatty acid transport into the brain. J Mol Neurosci. 33(1):12–17. doi: 10.1007/s12031-007-0050-3
- Han Y, Huang G, Zhang Q, Yuan S, Liu J, Zheng T, Fan L, Chen S, Xu A. 2010. The primitive immune system of amphioxus provides insights into the ancestral structure of the vertebrate immune system. Dev Comp Immunol. 34:791–796. doi: 10.1016/j.dci.2010.03.009
- Harmon CM, Luce P, Beth AH, Abumrad NA. 1991. Labeling of adipocyte membranes by sulfo-N-succinimidyl derivatives of long-chain fatty acids: inhibition of fatty acid transport. J Membr Biol. 121:261–268. doi: 10.1007/BF01951559
- Ikonen E. 2001. Roles of lipid rafts in membrane transport. Curr Opin Cell Biol. 13:470–477. doi: 10.1016/S0955-0674(00)00238-6
- Jump DB. 2004. Fatty acid regulation of gene transcription. Crit Rev Clin Lab Sci. 41:41–78. doi: 10.1080/10408360490278341
- Kamp F, Guo W, Souto R, Pilch PF, Corkey BE, Hamilton AJ. 2003. Rapid flip-flop of oleic acid across the plasma membrane of adipocytes. J Biol Chem. 278:7988–7995. doi: 10.1074/jbc.M206648200
- Kim J, Lim K. 2016. Relationship between FAT/CD36 protein in skeletal muscle and whole-body fat oxidation in endurance-trained mice. J Exerc Nutr Biochem. 20(4):48–52. doi: 10.20463/jenb.2016.0057
- Lefevre PG. 1975. The present state of the carrier hypothesis. Curr Top Membr Transport. 7:109–215. doi: 10.1016/S0070-2161(08)60859-X
- Lei CX, Ji H, Zhang JL, Ji H, Li J. 2016. Effects of dietary DHA/EPA ratios on fatty acid composition, lipid metabolism-related enzyme activity and gene expression of juvenile grass carp (Ctenopharyngodon idellus). J World Aquac Soc. 47(2):287–296. doi: 10.1111/jwas.12266
- Liao JF, Sportsman R, Harris J, Stahl A. 2005. Real-time quantification of fatty acid uptake using a novel fluorescence assay. J Lipid Res. 46:597–602. doi: 10.1194/jlr.D400023-JLR200
- Lie Ø, Lambertsen G. 1991. Fatty acid composition of glycerophospholipids in seven tissues of cod (Gadus morhua), determined by combined high-performance liquid chromatography and gas chromatography. J Chromatogr B Biomed Sci Appl. 565:119–129. doi: 10.1016/0378-4347(91)80376-N
- Liu Y, Neumann D, Glatz JFC, Luiken JJFP. 2016. Molecular mechanism of lipid induced cardiac insulin resistance and contractile dysfunction. Prostagl Leukot Essent Fat Acids. xx. ePub ahead of print.
- Livak KJ, Schmittgen TD. 2001. Analysis of relative gene expression data using real-time quantitative PCR and the 2−△△Ct method. Methods. 25:402–408. doi: 10.1006/meth.2001.1262
- Luiken JJFP, Chanda D, Nabben M, Neumann D, Glatz JFC. 2016. Post-translational modifications of CD36 (SR-B2): implications for regulation of myocellular fatty acid uptake. Biochim Biophys Acta. 1862:2253–2258. doi: 10.1016/j.bbadis.2016.09.004
- Luiken JJFP, van Nieuwenhoven FA, America G, van der Vusse GJ, Glatz JFC. 1997. Uptake and metabolism of palmitate by isolated cardiac myocytes from adult rats: involvement of sarcolemmal proteins. J Lipid Res. 38:745–758.
- Lu RH, Liang XF, Wang M, Zhou Y, Bai XL, He Y. 2012. The role of leptin in lipid metabolism in fatty degenerated hepatocytes of the grass carp Ctenopharyngodon idellus. Fish Physiol Biochem. 38:1759–1774. doi: 10.1007/s10695-012-9673-6
- McFarlan JT, Yoshida Y, Jain SS, Han XX, Snook LA, Lally J, Smith BK, Glatz JFC, Luiken JJFP, Sayer RA, et al. 2012. In vivo, fatty acid translocase (CD36) critically regulates skeletal muscle fuel selection, exercise performance, and training-induced adaptation of fatty acid oxidation. J Biol Chem. 287(28):23502–23516. doi: 10.1074/jbc.M111.315358
- National Research Council. 1993. Nutrient requirements of fish. Washington (DC): National Research Council, National Academy Press.
- Nisoli E, Carruba MO, Tonello C, Macor C, Federspil G, Vettor R. 2000. Induction of fatty acid translocase/CD36, peroxisome proliferator-activated receptor-gamma2, leptin, uncoupling proteins 2 and 3, and tumor necrosis factor-alpha gene expression in human subcutaneous fat by lipid infusion. Diabetes. 49:319–324. doi: 10.2337/diabetes.49.3.319
- Petersen TN, Brunak S, Heijne GV, Nielsen H. 2011. Signal P 4.0: discriminating signal peptides from transmembrane regions. Nature Methods. 8:785–786. doi: 10.1038/nmeth.1701
- Pownall HJ, Hamilton JA. 2003. Energy translocation across cell membranes and membrane models. Acta Physiologica Scandinavica. 178:357–365. doi: 10.1046/j.1365-201X.2003.01154.x
- Prabhudas M, Bowdish D, Drickamer K, Febbraio M, Herz J, Kobzik L, Krieger M, Loike J, Means TK, Moestrup SK, et al. 2014. Standardizing scavenger receptor nomenclature. J Immunol. 192:1997–2006. doi: 10.4049/jimmunol.1490003
- Richards JG, Bonen A, Heigenhauser GJF, Wood CM. 2003. Palmitate movement across red and white muscle membranes of rainbow trout. Am J Physiol Regul Integr Comp Physiol. 286:46R–R53. doi: 10.1152/ajpregu.00319.2003
- Schwieterman W, Sorrentino D, Potter BJ, Rand J, Kiang CL, Stump D, Berk PD. 1988. Uptake of oleate by isolated rat adipocytes is mediated by a 40-kDa plasma membrane fatty acid binding protein closely related to that in liver and gut. Proc Natl Acad Sci. 85:359–363. doi: 10.1073/pnas.85.2.359
- Silverstein RL, Febbraio M. 2009. CD36, a scavenger receptor involved in immunity, metabolism, angiogenesis, and behavior. Sci Signal. 2:re3. doi: 10.1126/scisignal.272re3
- Sorrentino D, Robinson RB, Kiang CL, Berk PD. 1989. At physiological albumin/oleate concentrations oleate uptake by isolated hepatocytes, cardiac myocytes and adipocytes is a saturable function of the unbound oleate concentration. Uptake kinetics are consistent with the conventional theory. J Clin Invest. 84:1325–1333. doi: 10.1172/JCI114301
- Sorrentino D, Stump D, Potter BJ, Robinson RB, White R, Kiang CL, Berk PD. 1988. Oleate uptake by cardiac myocytes is carrier mediated and involves a 40-Kd plasma membrane fatty acid binding protein similar to that in liver, adipose tissue and gut. J Clin Invest. 82:928–935. doi: 10.1172/JCI113700
- Stremmel W. 1988. Fatty acid uptake by isolated rat heart myocytes represents a carrier-mediated transport process. J Clin Invest. 81:844–852. doi: 10.1172/JCI113393
- Stremmel W, Berk PD. 1986. Hepatocellular influx of [14C] oleat reflects membrane transport rather than intracellular metabolism or binding. Proc Natl Acad Sci. 83:3086–3090. doi: 10.1073/pnas.83.10.3086
- Stubhaug I, Tocher DR, Bell JG, Dick JR, Torstensen BE. 2005. Fatty acid metabolism in Atlantic salmon (Salmo salar L.) hepatocytes and influence of dietary vegetable oil. Biochim Biophys Acta. 1734:277–288. doi: 10.1016/j.bbalip.2005.04.003
- Takeuchi T, Watanabe K, Yong W-Y, Watanabe T. 1991. Studies on nutritive value of dietary lipids in fish-XXXII. essential fatty acids of grass carp ctenopharyngodon idella. Nippon Suisan Gakkaishi. 57:467–473. doi: 10.2331/suisan.57.467
- Tian J, Ji H, Oku H, Zhou JS. 2014. Effects of dietary arachidonic acid (ARA) on lipid metabolism and health status of juvenile grass carp, Ctenopharyngodon idellus. Aquaculture. 430:57–65. doi: 10.1016/j.aquaculture.2014.03.020
- Tian J, Liu W, Gao WH, Wu F, Yu LJ, Lu X, Yang CG, Jiang M, Wen H. 2017. Molecular cloning and gene/protein expression of FAT/CD36 from grass carp (Ctenopharyngodon idella) and the regulation of its expression by dietary energy. Fish Physiol Biochem. 43:875–888. doi: 10.1007/s10695-017-0342-7
- Tian JJ, Lu RH, Ji H, Sun J, Li C, Liu P, Lei CX, Chen LQ, Du ZY. 2015. Comparative analysis of the hepatopancreas transcriptome of grass carp (Ctenopharyngodon idellus) fed with lard oil and fish oil diets. Gene. 565:192–200. doi: 10.1016/j.gene.2015.04.010
- Tocher DR. 2003. Metabolism and functions of lipids and fatty acids in teleost fish. Rev Fisheries Sci. 11:107–184. doi: 10.1080/713610925
- Toral PG, Bernard L, Delavaud C, Gruffat D, Leroux C, Chilliard Y. 2013. Effects of fish oil and additional starch on tissue fatty acid profile and lipogenic gene mRNA abundance in lactating goats fed a diet containing sunflower-seed oil. Animal. 7(6):948–956. doi: 10.1017/S1751731113000049
- Torstensen BE, Nanton DA, Olsvik PA, Sundvold H, Stubhaug I. 2009. Gene expression of fatty acid-binding proteins, fatty acid transport proteins (cd36 and FATP) and β–oxidation-related genes in (Salmo salar L.) fed fish oil or vegetable Oil. Aquac Nutr. 15:440–451. doi: 10.1111/j.1365-2095.2008.00609.x
- Torstensen BE, Stubhaug I. 2004. Beta-oxidation of 18:3n-3 in Atlantic salmon (Salmo salar L.) hepatocytes treated with different fatty acids. Lipids. 39:1–8. doi: 10.1007/s11745-004-1194-6
- Turcotte LP, Kiens B, Richter EA. 1991. Saturation kinetics of palmitate uptake in perfused skeletal muscle. FEBS Lett. 279:327–329. doi: 10.1016/0014-5793(91)80180-B
- Vallvé JC, Uliaque K, Girona J, Cabre A, Ribalta J, Heras M, Masana L. 2002. Unsaturated fatty acids and their oxidation products stimulate CD36 gene expression in human macrophages. Atherosclerosis. 164:45–56. doi: 10.1016/S0021-9150(02)00046-1
- Van der Lee KA, Vork MM, De Vries JE, Willemsen PH, Glatz JF, Reneman RS, Van der Vusse GJ, Van Bilsen M. 2000. Long-chain fatty acid-induced changes in gene expression in neonatal cardiac myocytes. J Lipid Res. 41:41–47.
- Vegusdal A, Gjøen T, Berge RK, Thomassen MS, Ruyter B. 2005. Effect of 18:1n-9, 20:5n-3 and 22:6n-3 on lipid accumulation and secretion by Atlantic salmon hepatocytes. Lipids. 40:477–486. doi: 10.1007/s11745-005-1407-z
- Xie P, Zhang AT, Wang C, Azzam MMM, Zou XT. 2012. Molecular cloning, characterization, and expression analysis of fatty acid translocase (FAT/CD36) in the pigeon (Columba livia domestica). Poult Sci. 91:1670–1679. doi: 10.3382/ps.2011-02097
- Yang Y, Chen M, Loux TJ, Harmon CM. 2007. Regulation of FAT/CD36 mRNA gene expression by long chain fatty acids in the differentiated 3T3-L1 cells. Pediatr Surg Int. 23:675–683. doi: 10.1007/s00383-007-1942-6
- Yu M, Guan HS, Guo HR, Zhang SC. 2003. Fish’s cell culture and its application. Ocean Sci. 27(3):4–8. (in Chinese).
- Zhou D, Samovski D, Okunade AL, Stahl PD, Abumrad NA, Su X. 2012. CD36 level and trafficking are determinants of lipolysis in adipocytes. FASEB J. 26(11):4733–4742. doi: 10.1096/fj.12-206862
- Zhou JS, Stubhaug I, Torstensen BE. 2010. Trans-membrane uptake and intracellular metabolism of fatty acids in Atlantic salmon (Salmo salar L.) hepatocytes. Lipids. 45:301–311. doi: 10.1007/s11745-010-3396-1
- Zhou JS, Torstensen BE, Stubhaug I. 2014. Oleic acid trans-membrane uptake in hepatocytes of Atlantic salmon (Salmo salar L.) and effect of replacing dietary fish oil with vegetable oil. Acta Hydrobiologica Sinica. 38(1):137–144. (in Chinese).