ABSTRACT
SQSTM1 (sequestosome 1, also known as p62) is a multifunctional scaffold protein implicated in diverse cell physiology processes, such as autophagy, cell signalling, and protein turnover. FKBP38 (FK506-binding protein 38) is a member of FKBPs family and plays a key role in various cellular processes, including signalling transduction, embryonic development and apoptosis. In order to explore the role of SQSTM1/p62 gene in cell-cycle progression and proliferation of goat foetal fibroblast (GFbs), SQSTM1/p62 gene was cloned and characterized. Furthermore, the yeast two-hybrid screening system was used to identify the interaction between SQSTM1/p62 and FKBP38. The results suggested that SQSTM1/p62 directly interacts with FKBP38. Overexpression vector pIRES-EGFP-SQSTM1/p62 and shRNA eukaryotic expression vector pRNAT-U6.1-shSQSTM1/p62 which harboured siRNA targeting the SQSTM1/p62 mRNA were constructed. The overexpression of SQSTM1/p62 gene in GFbs significantly increase the S-phase cells compared with control cells (p < .05). Furthermore, SQSTM1/p62 gene silencing in GFbs leads to a significant decrease of S-phase cells and cell cycle arrest compared with control cells (p < .05). These data indicate that SQSTM1/p62 gene plays an important role in cell-cycle and proliferation of Cashmere GFbs.
Abbreviations: Aba: aureobasidin A; EIF1: eukaryotic translation initiation factor 1; FKBP: FK506-binding protein; FKBP38: FK506-binding protein 38; GFbs: goat foetal fibroblast; mTOR: mammalian target of rapamycin; PDLIM7: PDZ and LIM domain 7; SD: standard dropout; SQSTM1/p62: sequestosome 1
1. Introduction
SQSTM1/p62 (sequestosome 1, also known as p62) is a scaffold protein that possesses PB1 and UBA domains, and the TRAF6 binding sequence (Moscat et al. Citation2007). SQSTM1/p62 is a multifunctional scaffold protein implicated in diverse biological processes, such as cell signalling, receptor internalization, and protein turnover. Proteins recruited to these domains enable SQSTM1/p62 to integrate kinase-activated and ubiquitin-mediated signalling pathways (Gade et al. Citation2016). SQSTM1/p62 interacts specifically with ubiquitinated proteins (Katsuragi et al. Citation2015), is a vital protein for cell autophagy. SQSTM1/p62 serves as an adaptor that brings ubiquitinated damaged proteins into the autophagosome for degradation (Weidberg et al. Citation2011). Various functions of SQSTM1/p62 have been suggested in cell physiology and pathology; however, the role of SQSTM1/p62 in regulating cell cycle and proliferation has received little attention.
FKBPs (FK506-binding proteins) are a family of proteins that have PPIase (peptidyl-prolyl cis–trans isomerase) activity and similar to cyclophilins in functions (Siekierka et al. Citation1989). FKBPs have been identified in many eukaryotes from yeast to humans and function as protein folding chaperones for proteins containing proline residues. As a new member of the FKBP family, FKBP38 was isolated from a cDNA library of human T cells in the early 1990s (Lam et al. Citation1995). FKBP38 contains an FKBP_C domain, two TPR domains and a TM domain (Zheng et al. Citation2012). In the absence of growth factors and nutrition, the FKBP_C domain may bind to the mammalian target of rapamycin (mTOR) to restrain the phosphorylation of S6K1 and 4EBP1, which are downstream regulatory factors of the mTOR signalling pathway. When growth factors and nutrients are rich, the FKBP_C domain may interact with Rheb-GTP (Ras homolog enriched in brain) to release mTOR (Bai et al. Citation2007). The silencing of the FKBP38 gene activates mTOR signalling in goat cells (Fu et al. Citation2015).
In the present study, we cloned the Cashmere goat SQSTM1/p62 gene and focused on the function of SQSTM1/p62 and the relationship of SQSTM1/p62 and FKBP38 gene in Cashmere goat foetal fibroblast (GFbs). The results show that SQSTM1/p62 could interact with FKBP38 and regulate cell cycle progression in Cashmere GFbs.
2. Materials and methods
2.1. Cell culture
Inner Mongolia Cashmere GFbs were maintained as monolayer cultures in DMEM/F12 (Gibco, Paisley, PA49RF, Scotland, UK) supplemented with 10% foetal bovine serum (FBS) and 100 U/mL penicillin G and 100 mg/mL streptomycin (FBS, Hyclone Laboratories, Inc. Logan, UT USA and penicillin/streptomycin, Sigma-Aldrich, Inc. St. Louis USA). Cell cultures were maintained and incubated at 37°C in humidified air with 5% CO2.
2.2. Cloning and characterization of the SQSTM1/p62 gene cDNA
To amplify Inner Mongolia Cashmere goat SQSTM1/p62 cDNA, a pair of specific primers (forward: 5′CTTGGTTGCCTTTTCCAGTGAC3′, reverse: 5′GATACTCTGTCTTTCCCCTGTG3′) was designed. The PCR programme was as follows: 94°C for 4min; 30 cycles at 94°C for 30 s, 56°C for 30 s, and 72°C for 1 min; and a final extension at 72°C for 10 min. The PCR products were cloned into pMD-19T (Takara, Dalian, China), and sequenced. The predicted length was 1147 base pairs.
The nucleotide sequence of the Cashmere goat SQSTM1/p62 cDNA and amino-acid sequences were deduced using the NCBI BLAST programme (www.ncbi.nlm.nih.gov/BLAST/). Prediction of the open reading frames (ORFs) and theoretical molecular weight of the deduced polypeptides was performed using the Protein property calculator (www.basic.northwestern.edu/biotools/proteincalc.html). The protein isoelectric point was predicted using the calculation of protein isoelectric point web programme (http://isoelectric.ovh.org/). The subcellular localization of SQSTM1/p62 was predicted using the PSORT programme (http://psort.ims.u-tokyo.ac.jp/form2.html). Protein domain analysis was completed using the SMART programme (http://smart.embl-heidelberg.de/). Protein prosite pattern analysis was identified by the Psite programme (www.softberry.com). Three-dimensional models of certain regions were generated using SWISSMODEL Workspace (http://swissmodel.expasy.org). Different phylogenetic trees were constructed from SQSTM1/p62 DNA sequences using the MEGA4.1 software with the neighbor-joining method.
2.3. Yeast two-hybrid analysis of protein interaction
Yeast maker Yeast Transformation System 2 and Matchmaker Gold Yeast Two-Hybrid System were purchased from Clontech (Clontech Laboratorie, Inc., Mountain View, CA, USA) and were performed according to the manufacturer’s instructions. Reagents for the synthetically defined plate for prototroph and colorimetric screening were also obtained from Clontech. An 1147 bp fragment of SQSTM1/p62 gene cDNA was subcloned into pGADT7 Vector (Clontech Laboratorie, Inc.) to generate the pGADT7-SQSTM1/p62 plasmid (expressing the prey protein GAL4AD- SQSTM1/p62). The pGBKT7-FKBP38 (expressing the bait protein GAL4BD-FKBP38) was described previously (Wang et al. Citation2014). The plasmids pGADT7-SQSTM1/p62 and pGBKT7-FKBP38 were co-transferred into the Y2HGold yeast strain. The cultures were plated on SD/-Leu-Trp-His/Amp+Kan+ medium. Diploid cells were then screened on an SD/-Leu-Trp-His-Ade/Amp+Kan+ plate, and the cell colonies were transferred to an SD/-Leu-Trp-His-Ade/X-α-Gal/Amp+Kan+ plate, and the blue colonies were transferred to an SD/-Leu-Trp-His-Ade/Abar/X-α-Gal/Amp+Kan+ for 3–6 days at 30°C, sequentially. Segregation was confirmed based on the appearance of the white and blue clones during the subcultivation process.
2.4. Plasmid construction
SQSTM1/p62 gene was sub-cloned into the pIRES2-EGFP vectors to construct the eukaryotic expression plasmids pIRES2-EGFP-SQSTM1/p62, which structure was shown in (Figure S1A). The small interfering RNA (siRNA) targeting the p62 sequence and the relevant short hairpin RNA (shRNA) were designed with sequence GGATCC CGTCCAGTCTCTACAGATGCCTG TTCAAGAGA CAGGCATCTGTAGAGACTGGA TTTTTTCCAA AAGCTT. A DNA fragment encoding the p62-shRNA was synthesized and then inserted into pRNAT-U6.1/Neo vector to construct the SQSTM1/p62-shRNA expression vector pRNAT-U6.1-shSQSTM1/p62, which structure was shown in (Figure S1B).
2.5. Transfection
The overexpression vector pIRES2-EGFP-SQSTM1/p62 and gene silencing vector pRNAT- U6.1-shSQSTM1/p62 were transferred into GFbs using lipofectamine™ 2000 reagent (Invitrogen, Carlsbad, New Mexico, USA) according to the manufacturer’s instructions, respectively. The transfected cells were imaged with a digital fluorescence microscope (Olympus IX71) after 48 h. The effects of SQSTM1/p62 overexpression and gene silencing were examined by western blot.
2.6. Western blot
Transfected cells and control cells were seeded into 6-well plates at 3×105 per well and incubated for 24 h in medium. Then cells were dissolved in cell lysis buffer (Thermo, USA). The concentrations of protein lysates were measured by Bio-Rad protein determination method (Bio-Rad, CA, USA). Equal amounts (40 μg) of protein were electrophoresed by SDS-PAGE in 12% gels, followed transferred to Immun-Blot PVDF membranes (Bio-Rad). Then the membranes were blocked with 5% non-fat milk for one hour at 37°C and probed with respective primary antibodies overnight at 4°C. After washing with TBST (0.1% Tween-20 in TBS) three times, the blots were incubated with secondary goat anti-rabbit IgG-(HRP) for one hour at room temperature. Finally, they were washed three times with TBST and detected using enhanced chemiluminescence (ECL) (GE Healthcare, UK). The resolved bands were quantified using Gel-Pro Analyzer 4.0 (Media Cybernetics, USA).
2.7. Cell cycle analysis
The transfected cells with pIRES2-EGFP-SQSTM1/p62 or pRNAT-U6.1-shSQSTM1/p62 plasmids and control cells were seeded into 6-well tissue culture plates at 3×105 cells per well and incubated for 48 h at 37°C. Cells were washed with cold PBS and stained with 50 mg/L propidium iodide (PI, Sigma-Aldrich, USA). DNA content was analyzed by flow cytometry (FACS Calibur, Becton-Dickinson Co., USA).
2.8. Statistical analysis
Descriptive statistics were generated for all quantitative data and these are presented as mean ± SD. Each assay was performed in triplicate. Statistical significance was defined as p < .05.
3. Results
3.1. Cloning and characterization of the SQSTM1/p62 gene cDNA
SQSTM1/p62 cDNA from Inner Mongolia Cashmere goat comprises an open reading frame (ORF) of 1147 bp, encoding 356 amino acid residues. The sequence is 99% identical to Capra hircus and Ovis Aries SQSTM1 and 96% identical to Bos taurus gene, respectively. To determine the phylogenetic relationships of an SQSTM1/p62 gene between of various species, the nucleotide sequence was aligned with those of other homologs, and a phylogenetic tree was constructed, based on these alignments (). Based on these results, we inferred that goat SQSTM1/p62 and sheep SQSTM1 are genetically closely related.
Figure 1. Phylogenetic tree of SQSTM1/p62 gene. Cashmere goat SQSTM1/p62 gene was aligned with other SQSTM1/p62 gene homologs, and a phylogenetic tree was constructed by neighbour-joining method using MEGA4.1. The species and Gene ID are Capra hircus (KY310590), Ovis Aries (XM_004011458), Bos Taurus (NM_001038539, Homo sapiens (KJ897404.1) and Mus musculus (NM_011185). The scale of evolutionary distance is 0.01.
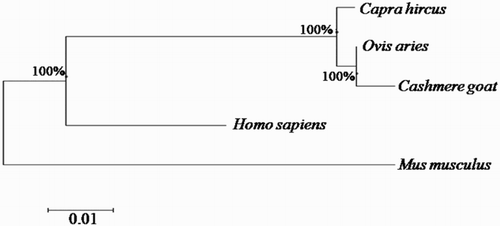
3.2. Primary and secondary structure of the putative Cashmere goat SQSTM1/p62 protein
We analyzed the predicted SQSTM1/p62 protein of the Cashmere goat; it is comprised of 356 amino-acid residues. The predicted molecular weight of the unmodified goat SQSTM1 protein is 38.54 kDa, and the isoelectric point (pI) is 4.88. The basic amino acids comprise 12.1% Ser, 9.6% Gly, and 7.3% Leu, Glu 8.7%, Pro 10.1%. The predict SQSTM1 protein contains a PB1 domain starting at site 1 and ending at site 18, a ZZ domain starting at site 39 and ending at site 79, and a UBA domain starting at site 309 and ending at site 348. There are putative 1 N-glycosylation site,2 Protein kinase C phosphorylation site, 9 Casein kinase II phosphorylation site, 5 N-myristoylation site,1 Amidation site, 1 Prenyl group binding site (CAAX box), 7 Microbodies C-terminal targeting signal. A protein prosite comparison of the goat SQSTM1/p62 with other four animals was also completed (). According to the calculations of the SWISS-MODEL workspace, we get 3D model of goat SQSTM1 (). The goat SQSTM1/p62 subcellular location is in nuclear.
Figure 2. The SQSTM1/p62 domains were predicted with the SMART Software (http://smart.embl.de/). The PB1 domain extends from amino acids 1 to 18. The ZZ domain extends from amino acids 39 to 79. UBA domain extends from amino acids 309 to 348.
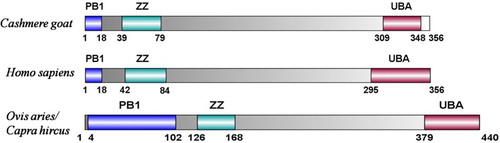
3.3. SQSTM1/p62 directly interacts with FKBP38
In order to identify the interaction between SQSTM1/p62 and FKBP38, we constructed the plasmids pGADT7-SQSTM1/p62 and co-transferred into Y2HGold yeast cells with pGBKT7-FKBP38. The transfected Y2H yeast cells which contain both the plasmids, were verified in 4 rounds screening with SD/-Leu-Trp-His/Amp + Kan+ ((A)), SD/-Leu-Trp-His-Ade/Amp + Kan+ ((B)), SD/-Leu-Trp-His-Ade/X-α-Gal/Amp + Kan+ ((C)) and SD/-Leu-Trp-His-Ade/Abar/X-α-Gal/Amp + Kan+ plates, sequentially. A blue colon was obtained as true positive clone (D). This means that the interaction between the SQSTM1/p62 and FKBP38 led to the GAL4 AD domain is close to the GAL4 BD domain, forming a complete GAL4 and the four reporter genes, AUR1-C, His3, ADE2, and MEL1, were expressed.
Figure 4. The Y2H yeast cells which contain plasmids pGADT7-SQSTM1 and pGBKT7-FKBP38 were verified in 4 rounds screening. (A) Yeasts were screened in the first round. The yeast cells were inoculated on SD/-Leu-Trp-His/Amp+Kan+ plate. (B) Yeasts were screened in the second. The yeast cells were inoculated on SD/-Leu-Trp-His-Ade/Amp+Kan+ plate. (C) Yeasts were screen in the third round. The yeast cells were inoculated on SD/-Leu-Trp-His-Ade/X-α-Gal/Amp+Kan+ plate. (D) In the fourth round of screening, the selected yeast cells were cultured on SD/-Leu-Trp-His-Ade/Abar/X-α-Gal/Amp+Kan+ plate. A blue clone was obtained and considered as positive clone, 4 reporter genes His3, ADE2, AUR1-C and MEL1 were all initiated.
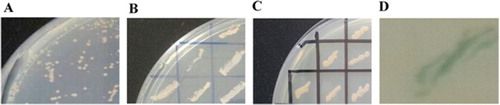
3.4. Overexpression and knockdown of SQSTM1/p62 affects cell cycle progression of GFbs
To study the function of SQSTM1/p62 in GFbs, we constructed the overexpression vector pIRES2-EGFP-SQSTM1/p62 and shRNA expression vector pRNAT-U6.1-shSQSTM1/p62. LipofectamineTM 2000 was used to transfect GFbs with pIRES2-EGFP-SQSTM1/p62 or pRNAT-U6.1-shSQSTM1/p62, respectively. The expression of EGFP was examined under a fluorescence microscope (Figure S2) to evaluate the transfected efficiency. Furthermore, expression of SQSTM1/p62 in transfected GFbs was analyzed by western-blot ((A,C)). The expression of SQSTM1/p62 in pRNAT-U6.1-shSQSTM1/p621 transfected cells is significant lower compared with pRNAT-U6.1 transfected cells ((B)) (p < .05) and significant higher in pIRES2-EGFP-SQSTM1/p62 transfected cells compared with pIRES2-EGFP transfected cells ((D)) (p < .05). Furthermore, we examined the cell-cycle of transfected cell by flow cytometry. The cell percentage of S phase in the p62 gene knockdown cells was 17.26% while the cells transfected with pRNAT-U6.1 had 27.74% S phase cells (p < .05). On the other hand, p62 overexpression in GFbs significantly increased the cell percentage in the S phases (36.72%) while the cells transfected with pIRES2-EGFP had 28.65% S phase cells (p < .05) (). These data indicate that SQSTM1/p62 gene knockdown induced G1/S cell-cycle arrest and overexpression promoted the cell-cycle process in GFbs. SQSTM1/p62 gene plays an important role in cell-cycle and proliferation of Cashmere GFbs.
Figure 5. Expression of SQSTM1/p62 protein detected by Western blot in transfected cells. (A) Expression of SQSTM1/p62 in the gene knockdown cells. Line 1: control cells; line 2: cells transfected with pRNAT-U6.1; line 3: cells transfected with pRNAT-U6.1-shSQSTM1/p62. β-actin was used as the loading control. (B) The data was processed by Gel-Pro Analyzer 4.0. Asterisk showed that expression of SQSTM1/p62 in the pRNAT-U6.1-shSQSTM1/p621 transfected cells is significantly lower compared with the pRNAT-U6.1 transfected cells (p < .05). (C) Expression of SQSTM1/p62 in the gene overexpressed cells. Line 1: control cells; line 2: cells transfected with pIRES2-EGFP; line 3: cells transfected with pIRES2-EGFP-SQSTM1/p62. β-actin was used as the loading control. (D) The data was processed by Gel-Pro Analyzer 4.0. Asterisk showed that expression of SQSTM1/p62 in the pIRES2-EGFP-SQSTM1/p62 transfected cells is significantly higher compared with the pIRES2-EGFP transfected cells (p < .05). The error bar shows three repeated experiment data were processed by Gel-Pro Analyzer average of standard deviation (SD).
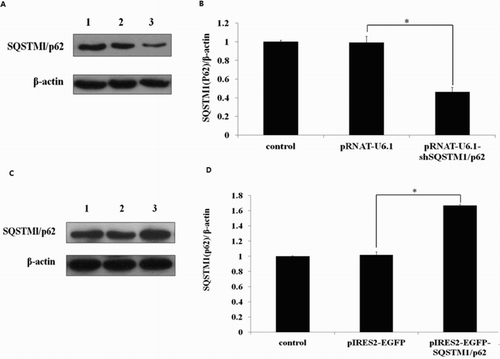
4. Discussion
In the recent years, interactomics has been considerably developed and applied in a global. The unbiased cell systems approach, or in a more targeted approach to study a specific set of proteins have led to a massive increase in our knowledge about the functional properties of genes and their encoded proteins (Alexeyenko et al. Citation2012) and the yeast two-hybrid methods are powerful tools for detecting protein–protein interactions (Goodman et al. Citation2013; Wang et al. Citation2013). There is growing interest in the use of interactomics to provide proteome- and genome-scale descriptions of the regulatory mechanisms of cell physical or pathological processes (Ijssennagger et al. Citation2015; Bellati et al. Citation2016). Large-scale screening technologies promoted the development of interactomics.
FKBP38 is a peptidyl-prolylcis/transisomerase (PPIase; EC5.2.1.8) of the FK506-binding protein (FKBP) family. It principally consists of an N-terminal catalytic FKBP domain and a C-terminal tetratricopeptide repeat (TPR) domain and a leucine-zipper repeat that facilitate its interaction with other proteins (Kang et al. Citation2013). The mTOR assembles a signalling network that regulates a myriad of cellular and developmental processes; FKBP38 is the mTORC1 inhibitor and for mTOR binding at a site encompassing the rapamycin-FKBP12 binding domain (Dunlop and Tee Citation2009; Yoon et al. Citation2011). In cells incubated with rapamycin, FKBPs interact with it and inhibit mTOR activity, which participates in the cell cycle by downregulating the PI3 K/AKT/mTOR complex (Lee et al. Citation2016).
Sequestosome 1 (SQSTM1/p62) has been extensively studied as a scaffold protein which is related with amino acid sensing and the oxidative stress response as well as autophagy. SQSTM1/p62 serves as a signalling hub for mammalian target of rapamycin complex 1 (mTORC1) activation on lysosomes (Siekierka et al. Citation1989; Lam et al. Citation1995; Zheng et al. Citation2012). Raptor, a subunit of the mTORC1 complex, was identified in an unbiased screen as an SQSTM1/p62-interacting protein (Estojak et al. Citation1995). Raptor has the ability to interact with SQSTM1/p62 in an amino acid-dependent manner, leading to their translocation onto lysosomes in response to elevated amino acid levels (Efeyan et al. Citation2012; Bar-Peled and Sabatini Citation2014). Thus, SQSTM1/p62 functions as a pivotal adaptor for mTORC1 activation on the lysosomal surface. In the present study, we identified SQSTM1/p62 interacting with FKBP38 and this relationship as well as its physiological significance has not been identified in other organisms.
In the previous studies, SQSTM1/p62 was focused as a vital protein for cell autophagy. Recent researches showed Atg5-mediated autophagy deficiency in proximal tubules promotes cell cycle G2/M arrest with degradation of SQSTM1/p62 in tubular epithelial cells (Li et al. Citation2016). CDKN1B/p27Kip1, a cell-cycle inhibitor, can form polymers with SQSTM1/p62 and regulate T lymphocyte proliferation (Jia et al. Citation2015). These evidences suggest that SQSTM1/p62 may be related to cell cycle. In this study, we found for the first time that SQSTM1/p62 was relevant with cell cycle as a positive regulator. Above all, our data suggest that SQSTM1/p62 is a novel partner of FKBP38 and take part in regulating the progress of cell-cycle in Cashmere GFbs.
5. Conclusion
The Cashmere goat SQSTM1/p62 gene was cloned and characterized. The yeast two-hybrid screening system was used to identify the interaction between SQSTM1/p62 and FKBP38. The results suggested that SQSTM1/p62 directly interacts with FKBP38. Further research showed that SQSTM1/p62 can regulate the cell-cycle progress of GFbs as a positive regulator. The interaction between FKBP38 and SQSTM1/p62 may play an important role in the cell physiological processes of Cashmere GFbs and it will be significant to explore the role of the interaction.
Supplemental Figures
Download Zip (4.2 MB)Disclosure statement
No potential conflict of interest was reported by the authors.
Additional information
Funding
References
- Alexeyenko A, Schmitt T, Tjärnberg A, Guala D, Frings O, Sonnhammer EL. 2012. Comparative interactomics with Funcoup 2.0. Nucleic Acids Res. 40:821–828. doi: 10.1093/nar/gkr1062
- Bai X, Ma D, Liu A, Shen X, Wang QJ, Liu Y, Jiang Y. 2007. Rheb activates mTOR by antagonizing its endogenous inhibitor, FKBP38. Science. 318:977–980. doi: 10.1126/science.1147379
- Bar-Peled L, Sabatini DM. 2014. Regulation of mTORC1 by amino acids. Trends Cell Biol. 24:400–406. doi: 10.1016/j.tcb.2014.03.003
- Bellati J, Champeyroux C, Hem S, Rofidal V, Krouk G, Maurel C, Santoni V. 2016. Novel aquaporin regulatory mechanisms revealed by interactomics. Mol Cell Proteomics. 15:3473–3487. doi: 10.1074/mcp.M116.060087
- Dunlop EA, Tee AR. 2009. Mammalian target of rapamycin complex 1: signalling inputs, substrates and feedback mechanisms. Cell Signal. 21:827–835. doi: 10.1016/j.cellsig.2009.01.012
- Efeyan A, Zoncu R, Sabatini DM. 2012. Amino acids and mTORC1: from lysosomes to disease. Trends Mol Med. 18:524–533. doi: 10.1016/j.molmed.2012.05.007
- Estojak J, Brent R, Golemis E. 1995. Correlation of two-hybrid affinity data with in vitro measurements. Mol Cell Biol. 15:5820–5829. doi: 10.1128/MCB.15.10.5820
- Fu YT, Zheng X, He Q, Jia XY, Guo ZX, Yao RY, Wang YF, Wang ZG. 2015. Silencing FKBP38 gene by siRNA induces activation of mTOR signaling in goat fetal fibroblasts. Genet Mol Res. 14:9675–9682. doi: 10.4238/2015.August.14.30
- Gade P, Kimball AS, DiNardo AC, Gangwal P, Ross DD, Boswell HS, Keay SK, Kalvakolanu DV. 2016. Death-associated protein kinase-1 expression and autophagy in chronic lymphocytic leukemia are dependent on activating transcription factor-6 and CCAAT/enhancer-binding protein-β. J Biol Chem. 291:22030–22042. doi: 10.1074/jbc.M116.725796
- Goodman SR, Daescu O, Kakhniashvili DG, Zivanic M. 2013. The proteomics and interactomics of human erythrocytes. Exp Biol Med (Maywood). 238:509–518. doi: 10.1177/1535370213488474
- Ijssennagger N, Belzer C, Hooiveld GJ, Dekker J, van Mil SW, Müller M, Kleerebezem M, van der Meer R. 2015. Gut microbiota facilitates dietary heme-induced epithelial hyperproliferation by opening the mucus barrier in colon. Proc Natl Acad Sci U S A. 112:10038–10043. doi: 10.1073/pnas.1507645112
- Jia W, He MX, McLeod IX, Guo J, Ji D, He YW. 2015. Autophagy regulates T lymphocyte proliferation through selective degradation of the cell-cycle inhibitor CDKN1B/p27Kip1. Autophagy. 11:2335–2345. doi: 10.1080/15548627.2015.1110666
- Kang C, Ye H, Chia J, Choi BH, Dhe-Paganon S, Simon B, Schütz U, Sattler M, Yoon HS. 2013. Functional role of the flexible N-terminal extension of FKBP38 in catalysis. Sci Rep. 3:2985–2993. doi: 10.1038/srep02985
- Katsuragi Y, Ichimura Y, Komatsu M. 2015. P62/SQSTM1 functions as a signaling hub and an autophagy adaptor. FEBS J. 282:4672–4678. doi: 10.1111/febs.13540
- Lam E, Martin M, Wiederrecht G. 1995. Isolation of a cDNA encoding a novel human FK506-binding protein homolog containing leucine zipper and tetratricopeptide repeat motifs. Gene. 160:297–302. doi: 10.1016/0378-1119(95)00216-S
- Lee SY, Lee H, Lee HK, Lee SW, Ha SC, Kwon T, Seo JK, Lee C, Rhee HW. 2016. Proximity-Directed labeling reveals a new rapamycin-induced heterodimer of FKBP25 and FRB in live cells. ACS Cent Sci. 2:506–516. doi: 10.1021/acscentsci.6b00137
- Li H, Peng X, Wang Y, Cao S, Xiong L, Fan J, Wang Y, Zhuang S, Yu X, Mao H. 2016. Atg5-mediated autophagy deficiency in proximal tubules promotes cell cycle G2/M arrest and renal fibrosis. Autophagy. 12:1472–1486. doi: 10.1080/15548627.2016.1190071
- Moscat J, Diaz-Meco MT, Wooten MW. 2007. Signal integration and diversification through the p62 scaffold protein. Trends Biochem Sci. 32:95–100. doi: 10.1016/j.tibs.2006.12.002
- Siekierka JJ, Hung SH, Poe M, Lin CS, Sigal NH. 1989. A cytosolic binding protein for the immunosuppressant FK506 has peptidyl-prolyl isomerase activity but is distinct from cyclophilin. Nature. 341:755–757. doi: 10.1038/341755a0
- Wang XJ, Wang YF, Zheng X, Hao XY, Liang Y, Wu ML, Wang X, Wang ZG. 2014. Direct interaction between Ras homolog enriched in brain and FK506 binding protein 38 in Cashmere goat fetal fibroblast cells. Asian Australas J Anim. 27:1671–1677. doi: 10.5713/ajas.2014.14145
- Wang L, Xu K, Lin J, Shao S, Zhang T, Xu H, Wei Z, Zhang Z. 2013. A novel genetic system based on zinc finger nucleases for the identification of interactions between proteins in vivo. PLoS One. 8:85650–85659. doi: 10.1371/journal.pone.0085650
- Weidberg H, Shvets E, Elazar Z. 2011. Biogenesis and cargo selectivity of autophagosomes. Annu Rev Biochem. 80:125–156. doi: 10.1146/annurev-biochem-052709-094552
- Yoon MS, Sun Y, Arauz E, Jiang Y, Chen J. 2011. Phosphatidic acid activates mammalian target of rapamycin complex 1 (mTORC1) kinase by displacing FK506 binding protein 38 (FKBP38) and exerting an allosteric effect. J Biol Chem. 286:29568–29574. doi: 10.1074/jbc.M111.262816
- Zheng X, Hao XY, Chen YH, Zhang X, Yang JF, Wang ZG, Liu DJ. 2012. Molecular characterization and tissue-specific expression of a novel FKBP38 gene in the Cashmere goat (Capra hircus). Asian Australas J Anim. 25:758–763. doi: 10.5713/ajas.2011.11398