ABSTRACT
The insulin-like growth factor binding protein (IGFBP) family participates in transportation, localization, and biological regulation of insulin-like growth factors (IGFs). In the present study, IGFBP5 gene from the Pinctada fucata (PfIGFBP5) was cloned and characterized. The full-length cDNA sequence (1319 bp) contained an open reading frame of 399 bp encoding a predicted protein of 132 amino acids. The amino acid sequence of PfIGFBP5 included an IGF binding domain and multiple cysteine residues. The genomic sequence of PfIGFBP5 consisted of three exons and two introns. PfIGFBP5 was found to be expressed in all tissues and developmental stages investigated, although the expression level was significantly higher in the pearl sac and in trochophore larvae than in other tissues or developmental stages (P < 0.05). Expression of PfIGFBP5 was induced by notching the oyster shell margin; the gene was mainly expressed in the outer epithelium of the mantle, and to a lesser extent in the whole pearl sac, including connective tissues. These findings suggest that PfIGFBP5 is very likely to be involved in modulating biomineralization in the mantle and pearl sac of P. fucata since they are the main organs for shell or pearl formation.
1. Introduction
The insulin-like growth factor (IGF) family is one of the most widely distributed peptide families in animals, including invertebrate species (Renteria et al. Citation2008). These peptides play pivotal roles in regulating growth, development, metabolism, and reproduction (Lardans et al. Citation2001; Riehle and Brown Citation2002; Schlueter et al. Citation2007). The IGF system generally includes two ligands (IGF-I and IGF-II), two cell surface receptors (IGF-IR and IGF-IIR), and a family of IGF-binding proteins (Hwa et al. Citation1999a; Duan et al. Citation2010). The IGF ligands interact with specific IGF membrane receptors, which possess tyrosine kinase activity, to activate downstream signal transduction networks regulating growth and metabolism (Denley et al. Citation2005). During this regulatory process, IGFBPs bind and transport IGFs as carriers and control the bioavailability and bioactivity of IGFs by modulating their interaction with IGF receptors (Denley et al. Citation2005). Many studies have indicated that in addition to their IGF-dependent activity IGFBPs also have IGF-independent actions during cell growth and differentiation (Firth and Baxter Citation2002; Tripathi et al. Citation2009).
The IGFBP family contains IGFBP1-6, which binds IGFs with high affinity, and IGFBP7-15 or IGFBP-rP, which bind IGF with low affinity but insulin with high affinity (Hwa et al. Citation1999a). The common skeleton of IGFBPs consists of an IGF binding (IB) domain at the N-terminus, a variable mid-region, and a TY domain at the C-terminus. Although structurally related, each IGFBP type has unique properties and performs stimulatory or inhibitory functions in different IGF-mediated biological activities (Wanscher et al. Citation2015; Breves et al. Citation2017). To date, most studies on IGFBPs have been carried out in vertebrate species, including Homo sapiens (Ding et al. Citation2016), Mus musculus (Moaeen-Ud-Din et al. Citation2015), and Danio rerio (Tripathi et al. Citation2009). The identified IGFBPs in vertebrate species are encoded by four exons, with the exception of IGFBP3 that is encoded by five exons (Hwa et al. Citation1999b). At present, comparatively little information is available on the IGFBPs of mollusks. An IGFBP gene was cloned from the scallop Patinopecten yessoensis and was suggested to have a role in biomineralization on the basis of its tissue expression profile; the gene was proposed to be to be an IGFBP5 ortholog (Feng et al. Citation2014). Similarly, IGFBP7 was cloned from Haliotis diversicolor and was suggested to be involved in responses to pathogenic infections in adults (Li et al. Citation2012). In addition, an IGFBP has been reported from Sinonovacula constricta (Xie et al. Citation2015). However, no IGFBPs have been described in the pearl oyster Pinctada fucata.
P. fucata is the main species used to produce pearls in China. It is well known that the mantle, especially the outer epithelium, plays an important role in pearl and shell formation (Sudo et al. Citation1997). Pearl culture involves transplanting a nucleus and pieces of outer epithelium of mantle tissue into a host where a pearl sac rapidly forms around the nucleus. The pearl sac then secretes nacre onto the nucleus to form a pearl (Acosta-Salmón et al. Citation2004). The selection of the mantle pieces is important as they directly determine the quality of the pearl (Taylor Citation2002). Thus, the mantle and pearl sac are associated with shell and pearl formation, a process of biomineralization in P. fucata. Shell and pearl formation involve a series of cell growth and differentiation stages. Currently, it is unclear whether any IGFBP is involved in the regulation of the pearl sac and mantle tissue growth and/or biomineralization.
In the present study, the cDNA sequence and the genomic structure of IGFBP5 gene(pfIGFBP5) in P. fucata were cloned and characterized. The expression of pfIGFBP5 mRNA in various tissues, at different developmental stages and in shell notching experiment were investigated. The precise positions of the gene expressions in the mantle and pearl sac were detected via in situ hybridization. Our results thus provide an insight into the functions of pfIGFBP5 in biomineralization.
2. Materials and methods
2.1. Sample preparation
Adult oysters (shell height 50 ± 1.45 mm, wet mass 45 ± 2.1 g) and larvae were obtained from the pearl oyster culture base of the South China Sea Fisheries Research Institute (Xincun Port, Hainan Province, China). Nuclear implantation was carried out by experienced technicians in June 2013 using a similar method as in conventional pearl culture practice. A total of 20 implanted oysters was sampled in September 2014. All individuals were kept in an 80 L aerated tank with sand-filtered seawater at 25°C and fed twice daily with Chlorella vulgaris for one week before dissection. Various adult tissues including mantle, gill, adductor muscle, gonad, hepatopancreas, and pearl sac were dissected. The pearl sac was excised from host oysters by removing the outer layers with a surgical blade until a thin tissue layer (<0.6 mm) surrounding the pearls remained (Zhu et al. Citation2015). All samples were immediately preserved in sample protector (TaKaRa) and stored at −80°C until RNA extraction.
Larvae at different developmental stages were obtained after artificial fertilization. The larvae were incubated in 4 × 6 × 1.6 m concrete tanks, at a temperature of 25°C and salinity of 25.0–28.9%. The following stages were collected: trochophore larvae (8 h post fertilization, hpf), D-shape veliger larvae (24 hpf), umbo veliger larvae (10 days post fertilization, dpf), pediveliger larvae (17 dpf), and larvae during metamorphosis (24 h post settlement). Different stage larvals were observed under binocular microscope to determine the exact stage and harvested using a 40 µm nylon mesh membrane. The larval samples were washed with 1× PBS (phosphate-buffered saline) and immediately preserved in 2 ml tubes containing sample protector (TaKaRa) until RNA extraction.
For the shell damage experiment, 48 healthy oysters were used, of which 24 individuals were damaged on the shell edge in accordance with the method described by shell notching exprement was carried out as described by Mount et al. (Citation2004). 24 healthy oysters were damaged on the shell edge (). Damage entailed cutting a V-shaped notch in the shell margin close to the mantle of the oyster. Mantle near the cut were collected from four individuals at 0 h (control group), 6, 12, 24, and 48 h post-notching, respectively and immediately preserved in sample protector and stored at −80°C. Adductor muscle was collected and frozen in liquid nitrogen for DNA extraction. Mantle and pearl sac tissues were excised from adult P. fucata and immediately fixed in 4% (w/v) paraformaldehyde at 4°C overnight for in situ hybridization.
2.2. RNA isolation and first-strand c DNA synthesis
Total RNA was isolated by TRIzol reagent (Invitrogen), following the manufacturer's protocol. The quality of RNA was assessed by electrophoresis on a 1% agarose gel,and the concentration and purity were examined at 260 and 280 nm, respectively, in NanoDropND-1000UV–Visible Spectrophotometer. 1 µg of total RNA from each sample was used as a template for a reverse transcription reaction using the Reverse Transcriptase MMLV Kit (TaKaRa). First-strand cDNA was synthesized and used as a template for further PCR analysis.
2.3. Cloning of Full-length cDNA of PfIGFBP5
cDNA fragment of PfIGFBP5 gene was obtained from the transcriptome sequences,through Blast X search with the NCBI database (http://www.ncbi.nlm.nih.gov/BLAST). The accuracy of PfIGFBP5 sequence was confirmed by using two pairs of gene-specific primers, IGFBP5-F and IGFBP5-R (). PCR was performed in a 20 µl reaction volume containing 13.8 µl double-distilled water, 2 µl 10× Ex Taq buffer, 1.6 µl dNTP mix (2.5 mmol l−1), 0.8 µl of each primer (10 mmol l−1), 0.2 µl Ex Taq (TaKaRa) and 0.8 µl cDNA as template. The PCR programme was as follows: denaturation at 94°C for 5 min, followed by 30 cycles at 94°C for 30 s, 52.5°C for 30 s, and 72°C for 1 min. The reaction was ended by a further extension of 10 min at 72°C.
Table 1. The main primers used in this study.
The full-length cDNA sequence was cloned using the SMARTer® RACE 5′/3′Kit (TaKaRa), according to the manufacturer's protocol. The gene-specific nested primers for 5′-RACE (IGFBP5-5′-out and IGFBP5-5′-inner) and 3′-RACE (IGFBP5-3′-outer and IGFBP5-3′-inner) were designed based on the partial cDNA fragment (). RACE PCR was performed in a 25 µl reaction volume containing 17.25 µl double-distilled water, 2.5 µl 10× Ex Taq buffer, 2 µl dNTP mix (2.5 mmol l−1), 1 µl of each primer (10 mmol l−1), 0.25 µl Ex Taq (TaKaRa) and 1 µl cDNA as template. The PCR programme was as follows:95°C for 5 min; 35 cycles of 94°C for 30 s, 55°C for 30 s, 72°C for 1 min; a final elongation step of 72°C for 10 min.
The PCR products were isolated using DNA Gel Extraction Kit (OMEGA, USA) and cloned into pMD18-T vector (TaKaRa) and sequenced.
2.4. Genome DNA extraction and cloning
Total genomic DNA was extracted from 20 mg of adductor muscle using the Marine Animals DNA kit (Tiangen, Beijing, China). DNA quality and quantity were determined by spectrophotometry and agarose gel electrophoresis. The full-length cDNA sequence of PfIGFBP5 was aligned with the draft genome of P. fucata (Takeuchi et al. Citation2012). Genomic sequences were amplified with the gene specific primers gIGFBP5-F1/R1, gIGFBP5-F2/R2, gIGFBP5-F3/R3, gIGFBP5-F4/R4 and gIGFBP5-F5/R5 (). PCR was performed in a 20 µl reaction volume, containing 13.8 µl double-distilled water, 2 µl 10× Ex Taq buffer, 1.6 µl dNTP mix (2.5 mmol l−1), 0.8 µl of each primer (10 mmol l−1), 0.2 µl Ex Taq (TaKaRa), and 0.8 µl DNA as template. PCR was performed as follows: denaturation at 94°C for 5 min, followed by 35 cycles at 94°C for 30 s, 55°C for 30 s, 72°C for 1 min and a final extension step at 72°C for 10 min. The amplified DNA product was separated by electrophoresis on a 1.5% agarose gel. The PCR products were isolated using DNA Gel Extraction Kit (OMEGA, USA) and cloned into pMD18-T vector (TaKaRa) and sequenced.
2.5. Bioinformatics analysis
The PfIGFBP5 cDNA sequence was analyzed with the BLAST algorithm at NCBI (http://blast.ncbi.nlm.nih.gov/Blast) for similarity to known gene. The protein transmembrane domain was predicted by TMHMM server2.0 (http://www.cbs.dtu.dk/services/TMHMM/). The ORF of PfIGFBP5 was predicted using ORF finder. (http://www.ncbi.nlm.nih.gov/gorf/orfig.cgi). Phosphorylation sites were predicted using NetPhos2.0 (http://www.cbs.dtu.dk/services/NetPhos/). Protein domain features were predicted by SMART (http://smart.embl-heidelberg.de/). Signal peptides were predicted by SignalP4.1 (http://www.cbs.dtu.dk/services/SignalP/). The physical and chemical properties of the protein were predicted by ProtParam (http://web.expasy.org/protparam/). The multiple alignments of the PfIGFBP5 proteins of P. fucata and other species were performed using the Clustal W2 multiple alignment programme (http://www.ebi.ac.uk/Tools/msa/clustalw2/).
2.6. Quantitative real-time quantitative RT-PCR (qRT-PCR)
A quantitative real-time PCR (qRT-PCR) was used to determine PfIGFBP5 mRNA levels in different tissues, different developmental stages and mantle after shell damage. First strand cDNA was synthesized using a PrimerScriptTM 1st strand cDNA synthesis Kit (TaKaRa). cDNA was diluted to 1:10 and stored at −20°C. Primers used for qRT-PCR were shown in . qRT-PCR was performed in triplicate for each sample using CFX96 real-time PCR Detection System (Eppendorf,Germany). PCR was performed in a 20 µl reaction volume, containing 10 µl 2× SYBR Green Real-time PCR Master Mix (TaKaRa), 0.4 µl of each primer (10 µM), 1.0 µl cDNA, and 8.2 µl RNase-free water. PCR cycling programme consisted of denaturation at 95°C for 30 s, 40 cycles at 95°C for 5 s, 55°C for 15 s. The 18S-rRNA gene was used as the reference for internal standardization (Miyazaki et al. Citation2010). The relative mRNA levels of PfIGFBP5 were calculated with the 2−ΔΔCT method (Livak and Schmittgen Citation2001). The statistical analysis was performed with one-way ANOVA and Duncan's variance significance tests for pairwise comparison,using SPSS 19.0. Differences were considered significant at P < 0.05.
2.7. In situ hybridization
The distribution of PfIGFBP5 mRNA in the mantle and pearl sac was analyzed using in situ hybridization with DIG-labelled antisense RNA probes. The probes were produced using the DIG RNA Labelling Kit (Biosense Bio-engineering Limited Company).The primers YIGFBP5-F1 and YIGFBP5-R1 were used to amplify Pf-EGFR () Amplified fragments were cloned into pGEM-easy T vector and recombinant plasmids were used as templates to synthesize both sense and antisense RNA probes by in vitro transcription.
A rectangular piece of mantle tissue and pearl sac tissue (0.8 × 0.5 cm) were fixed in 4% (w/v) paraformaldehyde in 1× PBS at 4°C for 24 h. Fixed tissue was dehydrated through an alcohol series and embedded in paraffin wax. Four micron thick sections were cut and dewaxed in xylene, then rehydrated in an alcohol series before hybridization. The DIG labelled sense or antisense probes were applied to the sections overnight for hybridization at 37°C. The tissue sections were then washed in PBS and visualized with the substrate BCIP/NBT (Roche).
3. Results
3.1. Cloning and sequence analysis of PfIGFBP5
The complete cDNA sequence of PfIGFBP5 was obtained and deposited in GenBank with the accession number KT899332.The full length cDNA of PfIGFBP5 consisted 1319 bp with a 5′-UTR of 543 bp, a 3′-UTR of 377 bp and an open reading frame of 399 bp ().The PfIGFBP5 gene encoded a protein sequence of 132 amino acid residues, with an estimated molecular mass of 14.52 kDa and an isoelectric point (pI) of 8.54, indicating that PfIGFBP5 was a hydrophilic protein. Sequence analysis indicated that PfIGFBP5 has a putative signal peptide (1-21 aa) in the N-terminal region. Three putative phosphorylation sites were also found at deduced amino acid positions 7 (Ser7), 72 (Thr72), and 125 (Ser125) of the mature protein (). The IB domain, a characteristic domain of the IGFBP protein family, was observed in the N-terminal region of PfIGFBP5. However, a CGCCXXC motif was present in P. fucata rather than the typical GCGCCXXC motif of IGFBP. In addition, 14 cysteine residues were deduced in amino acid sequence of PfIGFBP5. Multiple sequence alignments showed that the deduced IB domain was relatively conserved ().
Figure 2. The full-length cDNA and deduced amino acid sequences of PfIGFBP5. The red arrowheads mark the intron-exon boundaries in the PfIGFBP5 gene. Phosphorylation sites are marked with shadow. The characteristic GCGCCXXC motif is underlined. The poly A signal sequence is italicized. Two putative polyadenylation signals are in green.
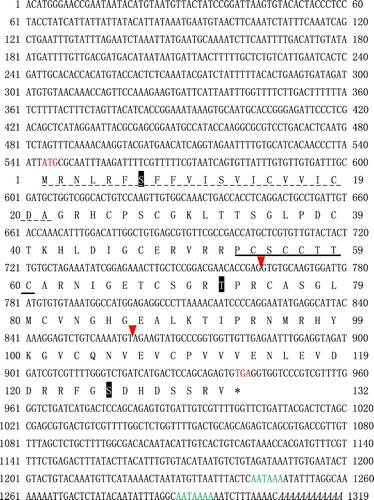
3.2. Genomic structure of PfIGFBP5
The genomic sequence of PfIGFBP5 was 5884 bp length (GenBank accession number KT899333). Comparison of the sequences of the genomic DNA and cDNA indicated that the gene contained three exons and two introns (). The lengths of introns 1 and 2 were 3859 bp and 706 bp, respectively. All intron-exon boundaries were consistent with the GT-AT rule.
3.3. Tissue expression profiles of PfIGFBP5
PfIGFBP5 mRNA was detected in all the six tissues examined, with a high level in pearl sac, moderate levels in mantle, gonad, muscle, and hepatopancreas, and a low level in gill (P < 0.05) ().
3.4. Expression levels of PfIGFBP5 during larval development
PfIGFBP5 was expressed in each larval development, with the highest level in trochophore larvae, and with lower levels in D veliger, metamorphic, pediveliger and umbo veliger larvae ().
3.5. PfIGFBP5 expression analysis after shell notching
The expression of PfIGFBP5 after notching of the shell margin is shown in . The relative levels of PfIGFBP5 mRNA varied with time post-notching. Compared to the control group (0 h), no significant differences in PfIGFBP5 expression were observed until 12 h post-notching. Expression increased significantly from 12 to 24 h, and then remained relatively constant from 24 to 48 h (). The maximum expression of PfIGFBP5 was occurred at 24 h.
3.6. Distribution of PfIGFBP5 in mantle and pearl sac
In situ hybridization was performed to determine the precise location of PfIGFBP5 mRNA expression in the mantle and pearl sac of P. fucata (). Strong hybridization signals were observed in the mantle edge, especially in the outer fold and the outer epithelium. Light hybridization signals were found in the outer epithelial cells of the mantle pallial zone and the mantle center. In addition, hybridization signals were detected on pearl sac. No hybridization signals were detected in negative controls.
Figure 8. The distribution of Pf PfIGFBP5 in mantle and pearl sac. Panel A-D are longitudinal sections of mantle tissue. Panel C-D were different higher magnifications of B. Panel E-H are cross sections of mantle tissue. Panel G-H were higher magnifications of F. Panel I-L are the distribution of PfIGFBP gene in pearl sac. Panel J-L were higher magnifications of I. Panel A and E are negative controls. No signal was observed in negative control. The black box represent the enlarged position. Hybridization signal are indicated with black arrowheads. ME, mantle edge; MP, mantle pallial; MC, mantle center; OF, outer fold; MF, middle fold; IF, inner fold; OEP, outer epithelium; PG, periostracal groove.
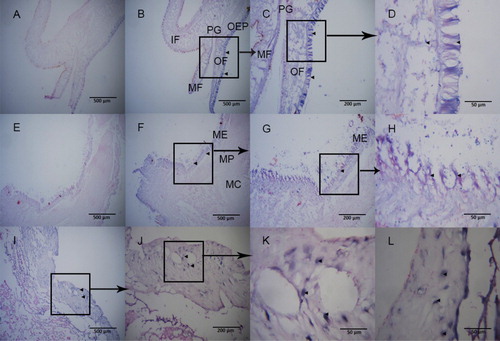
4. Discussion
IGFBPs have been reported to contain conserved cysteine-rich N-terminal and C-terminal domains and a variable mid-region (Lichtor et al. Citation1991). The PfIGFBP5 gene was cloned in this study. There was a long deletion in PfIGFBP5 between the mid-region and C-terminal region, similar to M. yessoensis (Feng et al. Citation2014) and S. constricta (Xie et al. Citation2015). A conserved IB domain was recognized in PfIGFBP5. The typical GCGCCXXC motif of IGFBP was mutated to -CSCCXXC in PfIGFBP5 with two amino acid differences. The typical thyroglobulin type-1 repeat (TY) domain in the C-terminal region of vertebrate IGFBPs is absent in all identified IGFBPs in invertebrate species.The IB domain of PfIGFBP5 was similar to that of most IGFBPs (). The identified IGFBPs in vertebrate species are encoded by four exons, except for IGFBP3 that has five exons (Hwa et al. Citation1999b). However, in invertebrate, IGFBP was composed of variable exon. PfIGFBP5 consisted of three exons (), and was consistent with IGFBP from M. yessoensis (Feng et al. Citation2014). However, S. constricta IGFBP only has two exons (Xie et al. Citation2015). These comparisons indicate that the structure of IGFBP in invertebrates is more complex than in vertebrates.
PfIGFBP5 was expressed in all tissues examined, especially expressed highly in pearl sac and mantle (). The pearl sac and mantle are the main organs for biomineralization during shell and pearl formation, respectively (Addadi et al. Citation2003; Kinoshita et al. Citation2011). Thus, the high expression of PfIGFBP5 in the pearl sac and mantle suggests that this gene may be involved in biomineralization in P. fucata, possibly in an IGF-independent manner as proposed by Luther et al. (Citation2013). The effects of IGF system members on mantle growth and shell formation have been demonstrated in other oyster species (Gricourt et al. Citation2003). In mammals, IGFBP5 is thought to be involved in bone formation,which is one kind of biomineralization procedure (Kanatani et al. Citation2000; Schneider et al. Citation2002). This suggests that the function of PfIGFBP5 is conservation during evolution.
The mantle can be divided into three regions: mantle edge, mantle pallial zone, and mantle center. Nacreous and prismatic layers of the shell are formed by secretions from epithelial cells in the mantle center and mantle edge (Sudo et al. Citation1997). The mantle edge is further subdivided into three terminal folds: an outer fold, a middle fold, and an inner fold (Wada Citation1999). Histological analysis has shown that the periostracum is formed in the periostracal groove, which lies between the outer and middle folds (Sudo et al. Citation1997). On the basis of the adult tissue expression results presented above, we performed in situ hybridization of PfIGFBP5 expression in the mantle and pearl sac for further verification of its function. In situ hybridization is a useful method to detect the location of mRNA expression in mantle or peal sac. Nakayama et al. (Citation2013) found the hybridization signals for P. fucata ppp-10 mRNA detected at the outer epithelial cells of the outer fold were strong, indicating that PPP-10 was secreted from the epithelium of the outer fold to the periostracum. A strong signal of HcCA3 gene of Hyriopsis cumingii wasdetected in epidermal cells in both the inside and outside of the mantle (Wang et al. Citation2017). EGFR of P. fucata was specifically expressed on both the inner side of the outer fold and the outer side of the inner fold of the mantle (Zhu et al. Citation2015). Our results showed strong hybridization signals in the mantle edge, especially in the outer fold and the outer epithelia (). Hybridization signals were also detected throughout the whole pearl sac including the epidermal cells and the connective tissues (). These findings suggest that PfIGFBP5 is mainly involved in the formation of nacreous and prismatic layers in P. fucata.
PfIGFBP5 mRNA expression was detected at all five developmental stages examined (). The highest level of PfIGFBP5 mRNA was found in the trochophore stage, with a slightly lower level in D-shape veliger larvae, and the lowest in umbo veliger larvae. In M. yessoensis, IGFBP mRNA was detected in newly fertilized eggs (Feng et al. Citation2014), implying that the transcripts might be involved in early development. However, in P. fucata, it is still unclear whether IGFBP functions in early development for muscle formation or in the preparations for shell formation in D-shape veliger larvae. In bivalve mollusks, muscle formation starts at the trochophore stage (Wanninger et al. Citation1999). The larval shell is termed the prodissoconch and is composed of two distinct shells: prodissoconch I and prodissoconch II (Miyazaki et al. Citation2010). The former is first formed in D-shape larvae and is secreted by the shell gland and mantle epithelia, just after the trochophore stage (Miyazaki et al. Citation2010). The second shell type is formed in later in D-shape larval development (Miyazaki et al. Citation2010). This pattern of development suggests that PfIGFBP5 could play an important role in the formation of prodissoconch shells during early larval development in bivalves.
In conclusion, pfIGFBP gene was successfully cloned and characterized in P. fucata. Our study indicates that PfIGFBP5 may be associated with biomineralization during larval development, shell growth, and pearl formation. However, our knowledge of the roles of pfIGFBP5 is currently limited and further investigations will be required.
Acknowledgments
Funding for this research was provided by the Special Fund for Central Public-Interest Scientific Institution Basal Research Fund, South China Sea Fisheries Research Institute, CAFS (NO. 2017YB10, 2015TS28), Guangxi Key Laboratory of Beibu Gulf Marine Biodiversity Conservation, Qinzhou University (2017KB01), Marine Fisheries Research and Extension of Guangdong Province (B201601-Z01).
Disclosure statement
No potential conflict of interest was reported by the authors.
Additional information
Funding
References
- Acosta-Salmon H, Martinezfernandez E, Southgate PC. 2004. A new approach to pearl oyster broodstock selection: can saibo donors be used as future broodstock? Aquaculture. 231:205–214. doi: 10.1016/j.aquaculture.2003.08.022
- Addadi L, Raz S, Weiner S. 2003. Taking advantage of disorder: amorphous calcium carbonate and its roles in biomineralization. Adv Mater. 15(33):959–970. doi: 10.1002/adma.200300381
- Breves JP, Fujimoto CK, Phipps-Costin SK, Einarsdottir IE, Björnsson BT, McCormick SD. 2017. Variation in branchial expression among insulin-like growth-factor binding proteins, (igfbps) during Atlantic salmon smoltification and seawater exposure. BMC Physiology. 17(1):275. doi: 10.1186/s12899-017-0028-5
- Denley A, Cosgrove LJ, Booker GW, Wallace JC, Forbes BE. 2005. Molecular interactions of the IGF system. Cytokine Growth Factor Rev. 16(4–5):421–439. doi: 10.1016/j.cytogfr.2005.04.004
- Ding H, Kharboutli M, Saxena R, Wu T. 2016. Insulin-like growth factor binding protein-2 as a novel biomarker for disease activity and renal pathology changes in lupus nephritis. Clin Exp Immunol. 184(1):11–18. doi: 10.1111/cei.12743
- Duan C, Ren H, Gao S. 2010. Insulin-like growth factors (IGFs), IGF receptors, and IGF-binding proteins: roles in skeletal muscle growth and differentiation. Gen Comp Endocrinol. 167:344–351. doi: 10.1016/j.ygcen.2010.04.009
- Feng L, Li X, Yu Q, Ning X, Dou J, Zou J, Zhang L, Wang S, Hu X, Bao Z, Liu Z. 2014. A scallop IGF binding protein gene: molecular characterization and association of variants with growth traits. PLoS One. 9:e89039. doi: 10.1371/journal.pone.0089039
- Firth SM, Baxter RC. 2002. Cellular actions of the insulin-like growth factor binding proteins. Endocr Rev. 23:824–854. doi: 10.1210/er.2001-0033
- Gricourt L, Bonnec G, Boujard D, Mathieu M, Kellner K. 2003. Insulin-like system and growth regulation in the Pacific oyster Crassostrea gigas: hrIGF-1 effect on protein synthesis of mantle edge cells and expression of an homologous insulin receptor-related receptor. Gen Comp Endocrinol. 134:44–56. doi: 10.1016/S0016-6480(03)00217-X
- Hwa V, Oh Y, Rosenfeld R. 1999a. Insulin-like growth factor binding proteins: a proposed superfamily. Acta Paediatrica. 88:37–45. doi: 10.1111/j.1651-2227.1999.tb14349.x
- Hwa V, Oh Y, Rosenfeld R. 1999b. The insulin-like growth factor-binding protein (IGFBP) superfamily 1. Endocr Rev. 20:761–787.
- Kanatani M, Sugimoto T, Nishiyama K, Chihara K. 2000. Stimulatory effect of insulin-like growth factor binding protein-5 on mouse osteoclast formation and osteoclastic bone-resorbing activity. J Bone Miner Res. 15:902–910. doi: 10.1359/jbmr.2000.15.5.902
- Kinoshita S, Wang N, Inoue H, Maeyama K, Okamoto K, Nagai K, Kondo H, Hirono I, Asakawa S, Watabe S. 2011. Deep sequencing of ESTs from nacreous and prismatic layer producing tissues and a screen for novel shell formation-related genes in the pearl oyster. PLoS One. 6:e21238. doi: 10.1371/journal.pone.0021238
- Lardans V, Coppin JF, Vicogne J, Aroca E, Delcroix M, Dissous C. 2001. Characterization of an insulin receptor-related receptor in Biomphalaria glabrata embryonic cells. Biochim Biophys Acta. 1510:321–329. doi: 10.1016/S0005-2736(00)00364-3
- Li N, Zhang Z, Zhang L, Wang S, Zou Z, Wang G, Wang Y. 2012. Insulin-like growth factor binding protein 7, a member of insulin-like growth factor signal pathway, involved in immune response of small abalone Haliotis diversicolor. Fish Shellfish Immunol. 33:229–242. doi: 10.1016/j.fsi.2012.04.016
- Lichtor T, Kurpakus MA, Gurney ME. 1991. Differential expression of insulin-like growth factor II in human meningiomas. Neurosurgery. 29:405–410. doi: 10.1227/00006123-199109000-00011
- Livak KJ, Schmittgen TD. 2001. Analysis of relative gene expression data using real-time quantitative PCR and the 2−ΔΔCT method. Methods. 25:402–408. doi: 10.1006/meth.2001.1262
- Luther GA, Lamplot J, Chen X, Rames R, Wagner ER, Liu X, Parekh A, Huang E, Kim SH, Shen J, Haydon RC. 2013. IGFBP5 domains exert distinct inhibitory effects on the tumorigenicity and metastasis of human osteosarcoma. Cancer Letters. 336(1):222–230. doi: 10.1016/j.canlet.2013.05.002
- Miyazaki Y, Nishida T, Aoki H, Samata T. 2010. Expression of genes responsible for biomineralization of Pinctada fucata during development. Comp Biochem Physiol B Biochem Mol Biol. 155:241–248. doi: 10.1016/j.cbpb.2009.11.009
- Moaeen-Ud-Din M, Bilal G, Reecy JM. 2015. Evolution of hypothalamus-pituitary growth axis among fish, amphibian, birds and mammals. Genetika. 47:665–677. doi: 10.2298/GENSR1502665M
- Mount AS, Wheeler A, Paradkar RP, Snider D. 2004. Hemocyte-mediated shell mineralization in the eastern oyster. Science. 304:297–300. doi: 10.1126/science.1090506
- Nakayama S, Suzuki M, Endo H, Iimura K, Kinoshita S, Watabe S, Kogure T, Nagasawa H. 2013. Identification and characterization of a matrix protein (PPP-10) in the periostracum of the pearl oyster, Pinctada fucata. Febs Open Bio. 3(1):421–427. doi: 10.1016/j.fob.2013.10.001
- Renteria ME, Gandhi NS, Vinuesa P, Helmerhorst E, Mancera RL. 2008. A comparative structural bioinformatics analysis of the insulin receptor family ectodomain based on phylogenetic information. Plos One. 3:e3667. doi: 10.1371/journal.pone.0003667
- Riehle MA, Brown MR. 2002. Insulin receptor expression during development and a reproductive cycle in the ovary of the mosquito Aedes aegypti. Cell Tissue Res. 308:409–420. doi: 10.1007/s00441-002-0561-8
- Schlueter PJ, Peng G, Westerfield M, Duan C. 2007. Insulin-like growth factor signaling regulates zebrafish embryonic growth and development by promoting cell survival and cell cycle progression. Cell Death Differ. 14:1095–1105. doi: 10.1038/sj.cdd.4402109
- Schneider M, Wolf E, Hoeflich A, Lahm H. 2002. IGF-binding protein-5: flexible player in the IGF system and effector on its own. J Endocrinol. 172:423–440. doi: 10.1677/joe.0.1720423
- Sudo S, Fujikawa T, Nagakura T, Ohkubo T, Sakaguchi K, Tanaka M, Nakashima K, Takahashi T. 1997. Structures of mollusc shell framework proteins. Nature. 387:563–564. doi: 10.1038/42391
- Takeuchi T, Kawashima T, Koyanagi R, Gyoja F, Tanaka M, Ikuta T, Shoguchi E, Fujiwara M, Shinzato C, Hisata K, et al. 2012. Draft genome of the pearl oyster Pinctada fucata: a platform for understanding bivalve biology. DNA Res. 19:117–130. doi: 10.1093/dnares/dss005
- Taylor J. 2002. Producing golden and silver south sea pearls from Indonesian hatchery reared Pinctada maxima. World Aquaculture 2002, 23rd-27th.
- Tripathi G, Salih DA, Drozd AC, Cosgrove RA, Cobb LJ, Pell JM. 2009. IGF-independent effects of insulin-like growth factor binding protein-5 (Igfbp5) in vivo. FASEB J. 23:2616–2626. doi: 10.1096/fj.08-114124
- Wada, K. 1999. Science of the pearl oyster. Tokyo: Shinju Shinbunsha. 336p (in Japanese).
- Wang GL, Wang Q, Xu ZC, Wang Y-Y, Li J-L. 2017. Identification, structural characterization and expression analysis of a novel carbonic anhydrase from freshwater mussel Hyriopsis cumingii. Gene. 636:78–86. doi: 10.1016/j.gene.2017.08.031
- Wanninger A, Ruthensteiner B, Dictus WJ, Haszprunar G. 1999. The development of the musculature in the limpet Patella with implications on its role in the process of ontogenetic torsion. Invertebr Repr Dev. 36:211–215. doi: 10.1080/07924259.1999.9652702
- Wanscher ASM, Williamson M, Ebersole TW, Streicher W, Wikström M, Cazzamali G. 2015. Production of functional human insulin-like growth factor binding proteins (IGFBPs) using recombinant expression in HEK293 cells. Protein Expr Purif. 108:97–105. doi: 10.1016/j.pep.2014.10.017
- Xie S, Niu D, Li J. 2015. Molecular characterization of IGFBP5 and accociation analysis with growth traits in the razor clam Sinonovacula constricta. J Fish China. 6, 004. (in Chinese).
- Zhu W, Fan S, Huang G, Zhang D, Liu B, Bi X, Yu D. 2015. Highly expressed EGFR in pearl sac may facilitate the pearl formation in the pearl oyster, Pinctada fucata. Gene. 566:201–211. doi: 10.1016/j.gene.2015.04.046