ABSTRACT
The efficacy of chitosan (CS) to enhance performance, immune response and reduce diarrhea rate in weaned piglets was evaluated. A total of 24 piglets were individually housed in pens. Animals received 1 of 2 dietary treatments: a control diet to which 500 mg/kg CS was added. The experiment lasted for 14 days. Body weight was recorded and daily feed intake was calculated. Fecal consistency was monitored throughout the experiment. At the end of the trial, blood and feces samples were collected to determine immune parameters, nutrient apparent digestibility and fecal microbial populations. Results showed that CS improved daily weight gain (p < .01) and feed conversion ratio (p < .01) during the overall period. Fecal score values were improved by CS compared to the control diet (p < .001) and showing decreased values in the entire period. The CS addition decreased E.coli (p = .02), Salmonella (p < .01) and Staphylococcus aureus (p < .01) counts, and increased the ratio of Lactobacillus to E.coli (p = .04) in the feces. Serum IL-2 (p = .02) and IgG (p = .07) levels were increased and serum cortisol (p < .05) was lowered in CS group compared to control group. The results suggested that the use of CS could improve performance and immune response of weaned piglets by promoting nutrient apparent digestibility, regulating the fecal microbiota as well as increasing serum IL-2 and IgG concentrations and decreasing serum cortisol level.
1. Introduction
Weaning is one of the most stressful events pigs encounter in life, as they are suffering from a series of great changes in diets (changing from liquid milk to a dry diet), physical (relocation to new housing, transportation and novel handling) and social (maternal separation, mixing with other unfamiliar mates and establishment of the social hierarchy within the group) environments (Ekkel et al. Citation1995; Pluske et al. Citation1997). The combined effects of these challenges can lead to imbalance in intestinal homoeostasis (Pluske et al. Citation1997). Specifically, abrupt weaning practices could result in intestine morphological changes (Pluske et al. Citation1997), endocrine disorders (Zhu et al. Citation2012), compromised immune system (Kick et al. Citation2012), increased occurrence of diarrhea, morbidity or mortality (Kelly et al. Citation1990) as well as reduced growth performance.
The use of antibiotics and zinc oxide in feed were popular as means of relieving the stress in piglets to overcome the disruption of growth performance after weaning. But there is a concern that the use of antibiotics may cause their residual in livestock products, and the microflora in animals might become antibiotic resistant, and this resistance could be transmitted to human pathogens (Barton Citation2000). And, the continuous supplementation of zinc leads to antibiotic resistance in Gram-negative bacteria and environmental contamination (Rincker et al. Citation2005; Vahjen et al. Citation2015). Thus, consistent efforts have been made to seek suitable natural alternatives to promote growth performance. Among these agents, chitosan (CS) has been demonstrated to have positive effects on pig health. Chitosan is a polymer consisting of β-(1-4)-2-acetamido-2-deoxy-D-glucose and β-(1-4)-2-amino-2-deoxy-D-glucose units (Arvanitoyannis et al. Citation1998). It is obtained by deacetylation of its parent polymer chitin, which is one of the widely available natural polymers distributing in nature. Chitosan is present in exoskeletons of arthropods like crustaceans, insects, cephalopod molluscs, or in the outer cell wall of yeast and fungi (Jayakumar et al. Citation2010; Dash et al. Citation2011). Compared with other natural polysaccharides, a plethora of applications of this biopolymer has been found due to its high biocompatible, biodegradable and low toxicity nature (Jayakumar et al. Citation2010; Dash et al. Citation2011). Chitosan has been reported to modify the gastrointestinal physiology of pigs, influence the intestinal mucosal morphology and endocrine system during weaning (Xu et al. Citation2013; Xu et al. Citation2014; Wan et al. Citation2017). In addition, CS has antibacterial potential in vivo (Han et al. Citation2007; Wan et al. Citation2017) and in vitro (Tsai and Hwang Citation2004). Overall, CS may offer a dietary means to modulate gut morphology and intestinal microbiota, thereby promote the productivity of farm animals.
The objective of this work was to evaluate the effects of dietary CS supply on growth performance, nutrient digestibility, selected fecal microbiota populations and immune status of weaned piglets. It is hypothesized that the inclusion of CS will enhance the selected indices of gut health in weaned piglets due to the biological properties of CS. This should result in an altered population of selected microbiota in feces as well as the improvement of pig’s immune status due to the immune enhancing effects of CS.
2. Materials and methods
2.1. Materials
Chitosan powder used in this experiment was supplied by Haidebei Marine Bioengineering Co., Ltd, China. It is a natural product extracted from shrimp shells and has active amino and hydroxyl group, which contribute to its many biological activities. The deacetylation degree (DD) of CS was measured via acid–base titration (Yuan et al. Citation2011) and its average molecular weight (Mw) was calculated via determination of intrinsic viscosity using the Mark-Houwink equation (Costa et al. Citation2015). The DD of CS was approximately 85% and average Mw was 232 kDa.
2.2. Animals, diets and experimental treatments
A total of 24 piglets (Duroc × Yorkshire × Landrace) weighing 10.20 (SD = 0.76) kg were selected and assigned to one of two experimental groups (n = 12 piglets/ experimental group, six males and six females). The dietary treatments were: 1) a control diet formulated to meet NRC (Citation2012) (); 2) control diet plus 500 mg/kg CS. Animals were individually housed in pens (2.0 × 2.2 m), and allowed ad libitum access to feed and water throughout the experiment.
Table 1. Composition and chemical analysis of basal experimental diets
2.3. Performance and collection procedures
The feeding trial lasted 14 days. Feed intake of per piglet was recorded daily. Piglets were weighed individually at the start and end of the feeding trial, and daily weight gain, daily feed intake and feed conversion ratio were calculated.
2.4. Fecal consistency
All piglets were individually observed for clinical signs of diarrhea from d 1 to d 14 of the experiment by a single operator with no prior knowledge of experimental groups. A scoring system was applied to indicate presence and extent of severity (Pierce et al. Citation2005). The following scoring system was used: 1 = hard firm feces; 2 = slightly soft feces; 3 = soft, partially formed feces; 4 = loose, semi-liquid feces (diarrhea); and 5 = watery, mucous-like feces (severe diarrhea).
2.5. Sample collection and preparation
Representative feed samples were collected and stored at -20 °C until chemical analysis. Fecal samples were collected twice daily (08:00 and 15:00) from d 13 to d 14 during the trial. After each collection, 10% H2SO4 was added to fix excreta nitrogen. Feed and feces samples were dried at 65 °C for at least 48 h till constant weight, then ground to pass through a 40-mesh screen and stored at –20 °C until analyzing the apparent nutrient digestibility (dry matter, crude protein, crude fat, crude ash, calcium and phosphorus).
On d 14 of the trial, fresh fecal samples were collected directly from the rectum of piglets in two sterile polyethylene tubes to avoid any additional contamination. Feces sample for microbial analysis was frozen at –20 °C. The rest fecal sample was kept on ice, and made 10% fecal homogenates by weighing 0.5 g of feces, and adding 4.5 mL of PBS (pH = 7.4), then mixed this mixture on a vortex for 10 min. The supernatant was collected from the suspensions after centrifugation (2,500 g, 10 min) and stored at –20 °C until the analysis of secretory Immunoglobulin A (sIgA).
At the end of the feeding trial, after an overnight fast, blood samples were collected from the precaval vein into 5 ml vacuum tubes and centrifuged (1,200 × g, 10 min) to separate serum. Serum samples were stored at –20 °C until further assays.
2.6. Chemical analyzes
The apparent nutrient digestibility was measured using endogenous indicator (4N-hydrochloric acid insoluble ashes, AIA) protocol. The AIA in feed and fecal samples was determined as method described by the Standards Press of China (Citation2009). All feed and fecal samples were analyzed for dry matter (method 930.15), crude protein (method 984.13), crude fat (method 920.39), crude ash (method 942.05), calcium (method 984.01) and phosphorus (method 965.17) as described by the AOAC (Citation2000). The calculation was made as follows:
Nutrient apparent digestibility (%) =100 - A1N2/A2N1×100,
Where A1 represents the AIA content of the feed; A2 represents the AIA content of feces; N1 represents the nutrient content of the feed and N2 represents the nutrient content of feces.
2.7. Microbial population determination
For microbiological analysis, fecal samples were collected sterilely and then serially diluted 101 to 108 folds with 0.9% sterile saline. From appropriate dilutions, 0.3 mL aliquots were inoculated into anaerobic roll tubes for determining Lactobacillus and dispensed onto agar plates for determining total aerobes, E. coli, Salmonella and Staphylococcus aureus. Microbial plates and roll tubes were inoculated with three dilutions, each in triplicate. The culture media for total aerobes, E. coli, Lactobacillus, Salmonella and Staphylococcus aureus are nutrient agar, eosin methylene blue agar (EMB), man rogosa and sharpe medium (MRS), salmonella-shigellaagar (SS), and mannitol salt agar (Huankai Microbial Sci. Tech. Co., Ltd, Guangzhou, China), respectively. After incubation for 24 h at 37 °C, the microbial colonies were immediately counted. The results are expressed as the log10 of a CFU per gram weight of wet fecal samples.
2.8. Fecal siga assay
The fecal sIgA content was measured using a porcine ELISA assay kit (Beijing 4A Biotech Co., Ltd., Beijing, China). The procedures were carried out according to the manufacturer’s instructions. The results were presented as μg sIgA/g feces.
2.9. Biochemical analysis of serum
Commercial porcine specific ELISA kits (Nanjing Jiancheng Bioengineering Institute, Nanjing, China) were used to quantify the serum cytokines (interleukin (IL)–1, IL-2, IL-6 and tumour necrosis factor (TNF)-a), the immunoglobulin (IgA, IgG and IgM), adrenocorticotropic hormone (ACTH) and cortisol concentrations according to the manufacturer’s instructions.
2.10. Statistical analysis
Each piglet was considered as an experimental unit for analysis. The effect of diet on fecal consistency was tested with an ANOVA using the GLM procedures (SAS Inst. Inc., Cary, NC) and including the effect of sampling day in the model. Other data were analyzed using a Student’s t-test in SAS. A value of p < .05 was considered statistically significant, whereas probability levels of .05 < p < .10 was considered as the tendency.
3. Results
3.1. Growth performance
As shown in , the final body weight (p = .04), daily weight gain (p < .01) and feed conversion ratio (p < .01) were improved by the addition of CS compared with the piglets fed the control diet, whereas no significance was attained concerning daily feed intake (p > .05).
Table 2. Effect of chitosan inclusion on growth performance of weaned piglets.
3.2. Fecal consistency and fecal moisture
Fecal consistency was scored during the overall experimental period (). There was an increase in fecal score values from d 0 to d 4 and then decrease from d 4 to d 14 for all of the diets (p < .01). During d 11, piglets consuming CS showed lower fecal score values compared with control (p = .021). In the entire period, fecal score was improved by CS compared with control (2.47 v.s. 2.26 for control and CS respectively; p < .01). However, there was no significant effect (p > .05) of CS inclusion on fecal moisture from d 13 to d 14 ().
Figure 1. Fecal consistency in piglets receiving a control diet, or the same diet supplemented with 500 mg/kg chitosan overall the 14 days (1 = hard feces (rarely seen), 2 = normal consistency of feces formed (no scours), 3 = soft, partially formed feces (mild scours), 4 = loose, semi-liquid feces (moderate scours), and 5 = watery feces (severe scours)). Values represent means ± SEM. Different letters in the same sampling day denote significant differences between diets. p-values for diet, day, and diet × day, as well as SEM values are from the ANOVA for the overall 14 days period.
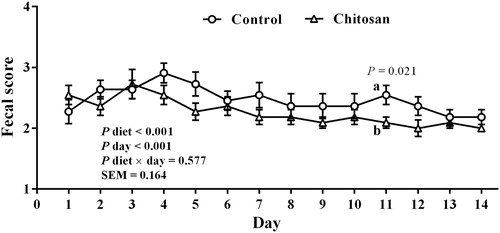
3.3. Fecal microbial populations
As shown in , piglets fed the CS diet had decreased fecal populations of E. coli (p = 0.02), Salmonella (p < .01) and Staphylococcus aureus (p < .01) compared with those fed the control diet. Expressed as a ratio of Lactobacillus to E.coli, the CS diet increased the ratio compared with control (p = .04). But, there was no effect of the diet containing CS on the fecal populations of total aerobes and Lactobacillus compared with the control diet.
Table 3. Effect of chitosan inclusion on fecal microbial populations of weaned piglets (log10 CFU/g feces).
3.4. Nutrient apparent digestibility
As shown in , piglets fed the diet containing CS had a greater apparent digestibility of crude protein (p = .07), calcium (p = .03) and phosphorus (p = .03) compared with those fed the control diet. However, when piglets were fed the diet containing CS, the apparent digestibility of crude fat was significantly decreased (p = .03).
Table 4. Effect of chitosan inclusion on nutrition apparent digestibility of weaned piglets
3.5. Serum ACTH and cortisol
As shown in , piglets fed diets containing CS had decreased concentration of serum cortisol when compared with those fed the control diet (p < .05). However, this effect was not observed on serum ACTH concentration (p > .05).
3.6. Cytokines and immunoglobulins
As shown in and , piglets fed diets supplemented with CS had an increased serum IL-2 (p = .02) and IgG (p = .07) concentration when compared with those fed the control diet. However, there were no significant effects (p > .05) of CS inclusion on serum IL-1, IL-6, TNF-α, IgM and IgA concentrations or fecal sIgA level.
Figure 4. Effect of chitosan inclusion on the concentration of fecal secretory IgA of weaned piglets. Values are expressed as the mean ± standard error (n = 12).
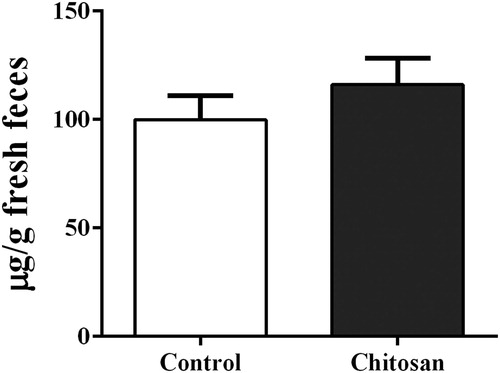
Table 5. Effect of chitosan inclusion on serum cytokines and immunoglobulins of weaned piglets
4. Discussion
Weaning under commercial conditions at an early age (3 to 4 weeks after birth) is an abrupt and stressful process in the whole life of the pig. Interestingly, several studies had testified that such weaning stress problems could be alleviated by supplementation with certain bioactive substances (Yin et al. Citation2008; Zhu et al. Citation2012). Therefore, we explored whether CS supplementation could mitigate weaning stress in weaned piglets. Diarrhea is the first and most clinical sign of illness after early weaning. In the current study, we provided evidence of a significant decrease in fecal score in CS-treated piglets, which indicated that dietary CS supplementation reduced the diarrhea rate. Several pathogenic microorganisms are considered to be associated with diarrhea, in which E.coli comes first (Sarmiento et al. Citation1988). A decline of E.coli could decrease the severity of diarrhea that appears after weaning (Melin et al. Citation2004). Enteropathogenic Salmonella can cause inflammation and damage of the intestine, resulting in diarrhea that may be accompanied by generalized sepsis, and all ages are susceptible in pigs (Meurens et al. Citation2009). Staphylococcus aureus can cause bacteraemia and sepsis (Nielsen et al. Citation2009), and the ratio of Lactobacillus to E.coli has been used as an indicator of gut health in pigs, with a decrease in the ratio considered harmful for gut health (Muralidhara et al. Citation1977). Chitosan showed antibacterial potential in vitro (Tsai and Hwang Citation2004; Jayakumar et al. Citation2010) and in vivo (Tsai and Hwang Citation2004). The present study showed a reduction of E.coli, Salmonella and Staphylococcus aureus populations in feces of piglets fed with CS. Likewise, previous study showed a lower population of E. coli in the caecum and colon of pigs fed diets with CS (Wan et al. Citation2017). These results suggested that CS could inhibit the proliferation of intestinal pathogens. However, the exact mechanism for the antibacterial activity remains unknown. One possible explanation may be effect of N-acetylglucosamine which is a basic component of CS (Klemm and Schembri Citation2000) as well as many mammalian glycoconjugates, e.g. mucins (Podolsky Citation1985). N-acetylglucosamine causes binding of CS to certain types of bacteria, thereby used as receptor preventing pathogens from binding to the intestinal mucosa and interfering with their adhesion and colonization in the gut of host animals (Klemm and Schembri Citation2000). Another possible mechanism is the electrostatic interaction between amino group of CS and phosphoryl group of bacterial outer membrane. The positive charge on the amino group of CS interacts with negative charge of bacterial cell membranes, which increases the permeability of the membrane and ultimately disrupts bacterial cell integrity, with the leakage of intracellular contents (Liu et al. Citation2004). Furthermore, the previous study showed that the decreased pH of intestinal contents might contribute to minimize intestinal pathogen generation in young animals (Mourao et al. Citation2006). It is unreasonable to assume the decreased pH is attributed to the dietary CS because CS is a natural weak alkaline compound, and it increases pH of gastric digesta (unpublished data). In addition, Beers-Schreurs van (Citation1996) described a lower fluid absorption in the large intestine shortly after weaning. However, the current experiment did not show up any decrease in the fecal moisture, which indicated that CS did not affect water absorption in the large intestine.
Recently, studies have shown that dietary provision of CS resulted in improved nutrient digestibility (Han et al. Citation2007; Chen et al. Citation2009; Xu et al. Citation2014). Consistent with above studies, the present data revealed an increase in apparent digestibility of crude protein, calcium and phosphorus in CS-fed pigs. Furthermore, our previous study showed that CS supplementation after weaning improved nutrient digestibility by enhancing digestive enzyme activities, e.g. amylase (Xu et al. Citation2014). Meanwhile, our previous work also verified an improvement in the small intestinal morphology in CS-fed piglets, which could contribute to a higher nutrient digestibility. On the other hand, a higher growth performance in the present study inversely supported the improved nutrient digestibility. However, in this study, the reduction of fat digestibility has no negative effect on growth performance of weaned piglets. But, massive ingestion of CS might resist fat-soluble vitamins such as vitamin A, vitamin D and vitamin E.
Weaning stress is a critical factor in relation to potential changes in the endocrine system (Boudry et al. Citation2004; Zhu et al. Citation2012). It is known that weaned piglets showed increased corticotrophin-releasing factor and cortisol release in serum (Moeser et al. Citation2007), suggesting that weaning induced activation of central stress pathways. As a glucocorticoide, cortisol is the main active hormone of the hypothalamic–pituitary–adrenal axis and has a potential metabolism and immune regulatory effect. When animals are suffering from stress, cortisol would be released from the adrenal gland, increasing the gluconeogenesis and the metabolism of carbohydrates, fats and proteins to produce energy, and ultimately protecting the body against stress (Fantidis Citation2010). Therefore, it is a sensitive parameter to monitor stress status of farm animals (Mormède et al. Citation2007). In the current study, the CS treatment caused decreased serum cortisol concentration, suggesting that CS could alleviate stress by regulating endocrine system in piglets.
Early weaning often is perceived to have potential changes in the immune system (Yin et al. Citation2008; Kick et al. Citation2012). The rapid dietary transition from liquid milk to solid feed principally disrupts passive immunity from sow’s milk, when innate and adaptive immune system of weaned piglets are yet to be fully developed and specialized (Gallois et al. Citation2009). The sudden cessation in the supply of passive immunity and an immature immune system makes piglets vulnerable to disease. CS, as an immunomodulator, enhanced immune system by providing protection against bacterial (Han et al. Citation2007; Moon et al. Citation2007), viral (Bacon et al. Citation2000) and endotoxin (Chen et al. Citation2009) diseases. Mechanisms of action include activation of monocyte or macrophages, and neutrophils, along with lymphocytes and increased cytokine production in macrophages (Moon et al. Citation2007; Chen et al. Citation2009). The results of our experiment also confirmed that CS regulated the production of cytokines and immunoglobulins. For example, serum IL-2 and IgM concentrations were all elevated as the result of CS supplementation. In agreement, other researchers reported that supplementation of CS favoured an improvement of immunity in piglets by increasing secretion of immunoglobulins (Yin et al. Citation2008).
Collectively, CS could promote growth performance and prevent diarrhea in weaned piglets, which was probably mediated by increasing nutrient apparent digestibility, regulating intestinal microbiota and modulating the production of cytokines and antibodies.
5. Conclusions
In summary, our findings identified chitosan as a potent natural compound could relieve weaning stress and prevent diarrhea in weaned piglets. Moreover, the improvement in growth performance was partly achieved by improving nutrient digestibility, decreasing fecal pathogens populations and modulating immune response.
Disclosure statement
No potential conflict of interest was reported by the authors.
Acknowledgments
The author thanks their laboratory colleagues Zhe Qin, Shiwei Guo, and Pengfei Zhang for their assistance in the data and sample collection, and laboratory analysis.
ORCID
Sumei Yan http://orcid.org/0000-0002-4107-2858
Additional information
Funding
References
- AOAC. 2000. Official methods of analysis, 17th ed. Arlington, VA, USA: AOAC International.
- Arvanitoyannis IS, Nakayama A, Aiba S. 1998. Chitosan and gelatin based edible films: state diagrams, mechanical and permeation properties. Carbohydr Polym. 37:371–382. doi: 10.1016/S0144-8617(98)00083-6
- Bacon A, Makin J, Sizer PJ, Jabbal-Gill I, Hinchcliffe M, Illum L, Chatfield S, Roberts M. 2000. Carbohydrate biopolymers enhance antibody responses to mucosally delivered vaccine antigens. Infect Immun. 68:5764–5770. doi: 10.1128/IAI.68.10.5764-5770.2000
- Barton MD. 2000. Antibiotic use in animal feed and its impact on human health. Nutr Res Rev. 13:279–299. doi: 10.1079/095442200108729106
- Beers-Schreurs van HMG. 1996. The changes in the function of the large intestine of weaned pigs. Ph.D. Utrecht: University Utrecht.
- Boudry G, Peron V, Le Huerou-Luron I, Lalles JP, Seve B. 2004. Weaning induces both transient and long-lasting modifications of absorptive, secretory, and barrier properties of piglet intestine. J Nutr. 134:2256–2262. doi: 10.1093/jn/134.9.2256
- Chen YJ, Kim IH, Cho JH, Yoo JS, Wang Y, Huang Y, Kim HJ, Shin SO. 2009. Effects of chitooligosaccharide supplementation on growth performance, nutrient digestibility, blood characteristics and immune responses after lipopolysaccharide challenge in weanling pigs. Livest Sci. 124:255–260. doi: 10.1016/j.livsci.2009.02.006
- Costa CN, Teixeira VG, Delpech MC, Souza JVS, Costa MAS. 2015. Viscometric study of chitosan solutions in acetic acid/sodium acetate and acetic acid/sodium chloride. Carbohydr Polym. 133:245–250. doi: 10.1016/j.carbpol.2015.06.094
- Dash M, Chiellini F, Ottenbrite RM, Chiellini E. 2011. Chitosan-A versatile semi-synthetic polymer in biomedical applications. Prog Polym Sci. 36:981–1014. doi: 10.1016/j.progpolymsci.2011.02.001
- Ekkel ED, van Doorn CE, Hessing MJ, Tielen MJ. 1995. The specific-stress-free housing system has positive effects on productivity, health, and welfare of pigs. J Anim Sci. 73:1544–1551. doi: 10.2527/1995.7361544x
- Fantidis P. 2010. The role of the stress-related anti-inflammatory hormones ACTH and cortisol in atherosclerosis. Curr Vasc Pharmacol. 8:517–525. doi: 10.2174/157016110791330889
- Gallois M, Rothkotter HJ, Bailey M, Stokes CR, Oswald IP. 2009. Natural alternatives to in-feed antibiotics in pig production: can immunomodulators play a role? Animal. 3:1644–1661. doi: 10.1017/S1751731109004236
- Han KN, Kwon IK, Lohakare JD, Heo S, Chae BJ. 2007. Chito-oligosaccharides as an alternative to antimicrobials in improving performance, digestibility and microbial ecology of the gut in weanling pigs. Asian-Australas J Anim Sci. 20:556–562. doi: 10.5713/ajas.2007.556
- Jayakumar R, Menon D, Koyakutty M, Nair SV, Tamura H. 2010. Biomedical applications of chitin and chitosan based nanomaterials-A short review. Carbohydr Polym. 82:227–232. doi: 10.1016/j.carbpol.2010.04.074
- Kelly D, O’Brien JJ, McCracken KJ. 1990. Effect of creep feeding on the incidence, duration and severity of postweaning diarrhoea in pigs. Res Vet Sci. 49:223–228. doi: 10.1016/S0034-5288(18)31082-8
- Kick AR, Tompkins MB, Flowers WL, Whisnant CS, Almond GW. 2012. Effects of stress associated with weaning on the adaptive immune system in pigs. J Anim Sci. 90:649–656. doi: 10.2527/jas.2010-3470
- Klemm P, Schembri MA. 2000. Bacterial adhesins: structure and function. Int J Med Microbiol. 290:27–35. doi: 10.1016/S1438-4221(00)80102-2
- Liu H, Du HY, Wang XH, Sun LP. 2004. Chitosan kills bacteria through cell membrane damage. Int J Food Microbiol. 95:147–155. doi: 10.1016/j.ijfoodmicro.2004.01.022
- Melin L, Mattson S, Katouli M, Wallgren P. 2004. Development of post-weaning diarrhoea in piglets. relation to presence of escherichia coli strains and rotavirus. J Vet Med B Infect Dis Vet Public Health. 51:12–22. doi: 10.1111/j.1439-0450.2003.00723.x
- Meurens F, Berri M, Auray G, Melo S, Levast B, Virlogeux-Payant I, Chevaleyre C, Gerdts V, Salmon H. 2009. Early immune response following salmonella enterica subspecies enterica serovar typhimurium infection in porcine jejunal gut loops. Vet Res. 40:05. doi: 10.1051/vetres:2008043
- Moeser AJ, Klok CV, Ryan KA, Wooten JG, Little D, Cook VL, Blikslager AT. 2007. Stress signaling pathways activated by weaning mediate intestinal dysfunction in the pig. Am J Physiol Gastrointest Liver Physiol. 292:G173–G181. doi: 10.1152/ajpgi.00197.2006
- Moon JS, Kim HK, Koo HC, Joo YS, Nam HM, Park YH, Kang MI. 2007. The antibacterial and immunostimulative effect of chitosan-oligosaccharides against infection by staphylococcus aureus isolated from bovine mastitis. Appl Microbiol Biotechnol. 75:989–998. doi: 10.1007/s00253-007-0898-8
- Mormède P, Andanson S, Aupérin B, Beerda B, Guémené D, Malmkvist J, Manteca X, Manteuffel G, Prunet P, van Reenen CG, et al. 2007. Exploration of the hypothalamic-pituitary-adrenal function as a tool to evaluate animal welfare. Physiol Behav. 92:317–339. doi: 10.1016/j.physbeh.2006.12.003
- Mourao JL, Pinheiro V, Alves A, Guedes CM, Pinto L, Saavedra MJ, Spring P, Kocher A. 2006. Effect of mannan oligosaccharides on the performance, intestinal morphology and cecal fermentation of fattening rabbits. Anim Feed Sci Technol. 126:107–120. doi: 10.1016/j.anifeedsci.2005.06.009
- Muralidhara KS, Sheggeby GG, Elliker PR, England DC, Sandine WE. 1977. Effect of feeding lactobacilli on the coliform and lactobacillus flora of intestinal tissue and feces from piglets. J Food Prot. 40:288–295. doi: 10.4315/0362-028X-40.5.288
- Nielsen OL, Iburg T, Aalbaek B, Leifsson PS, Agerholm JS, Heegaard P, Boye M, Simon S, Jensen KB, Christensen S, et al. 2009. A pig model of acute staphylococcus aureus induced pyemia. Acta Vet Scand. 51:14. doi: 10.1186/1751-0147-51-14
- NRC. 2012. Nutrient requirements of swine, 11th ed. Washington, DC, USA: National Academy Press.
- Pierce KM, Callan JJ, McCarthy P, O’Doherty JV. 2005. Performance of weanling pigs offered low or high lactose diets supplemented with avilamycin or inulin. Anim Sci. 80:313–318. doi: 10.1079/ASC40900313
- Pluske JR, Hampson DJ, Williams IH. 1997. Factors influencing the structure and function of the small intestine in the weaned pig: a review. Livest Prod Sci. 51:215–236. doi: 10.1016/S0301-6226(97)00057-2
- Podolsky DK. 1985. Oligosaccharide structures of human colonic mucin. J Biol Chem. 260:8262–8271.
- Rincker MJ, Hill GM, Link JE, Meyer AM, Rowntree JE. 2005. Effects of dietary zinc and iron supplementation on mineral excretion, body composition, and mineral status of nursery pigs. J Anim Sci. 83:2762–2774. doi: 10.2527/2005.83122762x
- Sarmiento JI, Casey TA, Moon HW. 1988. Postweaning diarrhea in swine: experimental model of enterotoxigenic escherichia coli infection. Am J Vet Res. 49:1154–1159.
- Standards Press of China. 2009. Measurement of the acid insoluble Ash in feed, GB/T 23742. Beijing: Standardization Administration of the People's Republic of China.
- Tsai GJ, Hwang SP. 2004. In vitro and in vivo antibacterial activity of shrimp chitosan against some intestinal bacteria. Fish Sci. 70:675–681. doi: 10.1111/j.1444-2906.2004.00856.x
- Vahjen W, Pietruszynska D, Starke IC, Zentek J. 2015. High dietary zinc supplementation increases the occurrence of tetracycline and sulfonamide resistance genes in the intestine of weaned pigs. Gut Pathog. 7:23. doi: 10.1186/s13099-015-0071-3
- Wan J, Jiang F, Xu QS, Chen DW, Yu B, Huang ZQ, Mao XB, Yu J, He J. 2017. New insights into the role of chitosan oligosaccharide in enhancing growth performance, antioxidant capacity, immunity and intestinal development of weaned pigs. RSC Adv. 7:9669–9679. doi: 10.1039/C7RA00142H
- Xu YQ, Shi BL, Yan SM, Li TY, Guo YW, Li JL. 2013. Effects of chitosan on body weight gain, growth hormone and intestinal morphology in weaned pigs. Asian-Australas J Anim Sci. 26:1484–1489. doi: 10.5713/ajas.2013.13085
- Xu Y, Shi B, Yan S, Li J, Li T, Guo Y, Guo X. 2014. Effects of chitosan supplementation on the growth performance, nutrient digestibility, and digestive enzyme activity in weaned pigs. Czech J Anim Sci. 59:156–163. doi: 10.17221/7339-CJAS
- Yin YL, Tang ZR, Sun ZH, Liu ZQ, Li TJ, Huang RL, Ruan Z, Deng ZY, Gao B, Chen LX, et al. 2008. Effect of galacto-mannan-oligosaccharides or chitosan supplementation on cytoimmunity and humoral immunity response in early-weaned piglets. Asian-Australas J Anim Sci. 21:723–731. doi: 10.5713/ajas.2008.70408
- Yuan Y, Chesnutt BM, Haggard WO, Bumgardner JD. 2011. Deacetylation of chitosan: material characterization and in vitro evaluation via albumin adsorption and pre-osteoblastic cell cultures. Materials (Basel). 4:1399–1416. doi: 10.3390/ma4081399
- Zhu LH, Zhao KL, Chen XL, Xu JX. 2012. Impact of weaning and an antioxidant blend on intestinal barrier function and antioxidant status in pigs. J Anim Sci. 90:2581–2589. doi: 10.2527/jas.2011-4444