ABSTRACT
The purpose of this study was to determine effects of inclusion of Zeolite in lactating dairy cows’ rations on blood Ca, P, and Mg status during periparturient period, as well as on milk yield and composition during early lactation. Forty-two pregnant dry Holstein cows were randomly assigned as Zeolite treated cows (EG) or untreated cows (CG) comprising 21 cows each. The EG group received the same diet as the CG group, but with addition of 200 g/cow/day of Zeolite. There was no treatment effect on milk total solids, milk fat, milk protein, lactose, milk ash, milk Ca, milk P, milk Mg, plasma P, and plasma Mg for CG and EG. Conversely, milk yield, fat corrected milk (FCM), fat yield, protein yield, lactose yield, and plasma Ca were significantly increased by Zeolite addition. These results indicate that Zeolite can be effectively used in the rations of dry and lactating cows with positive effects on milk production and components yields and no deleterious effects on milk composition or blood parameters. Blood Ca was enhanced around calving and at the beginning of lactation. It is suggested that prepartum Zeolite supplementation may alleviate the negative Ca balance and therefore reduce the incidence of subclinical hypocalcaemia during the periparturient period.
1. Introduction
A major challenge for dairy producers, managers, and veterinarians is to maintain a dairy cow's health during the periparturient period (the dry period and the first 3–4 weeks after calving). This transition period is characterized by the appearance of periparturient disorders, such as hypocalcaemia or milk fever. It is a metabolic disease caused by a low blood Ca level close to calving. It occurs when cows are unable to compensate for the dramatic increase in Ca needed for colostrum and milk production at calving (Smith and Risco Citation2005; Charbonneau et al. Citation2006). The most dramatic clinical milk fever is made when Ca blood concentration levels are lower than 60 mg/L (Allen and Sansom Citation1993). The diagnosis of subclinical milk fever, a milder form of hypocalcaemia, using blood Ca concentration values is more difficult, but measured values in the range of 62–75 mg/L have been suggested (Risco et al. Citation1984; Shearer and van Horn Citation1992).
Milk fever or parturient paresis (between 3% and 10% of cows) generally increases the risk for dystocia, retained foetal placenta, ketosis, and mastitis, and tends to increase calving intervals and slightly reduce milk yield (Stevenson and Call Citation1988; Goff Citation2006). Occurrence of milk fever increases when high-calcium feeds are offered during the dry period. Older cows and all cows with high milk production are at greater risk for milk fever. One potential way of preventing parturient hypocalcaemia involves feeding a prepartal diet that is Ca deficient (<20 g/d or 0.5% Ca diet). However, this method of prevention has been almost abandoned because it is difficult to formulate rations sufficiently low in calcium when using commonly available feeds (Thilsing-Hansen et al. Citation2002, Citation2007).
Recent studies have shown, however, that it is possible to prevent milk fever, as well as subclinical hypocalcaemia, by supplementing the dry cow ration with substance that has the capacity to bind Ca in the intestinal tract such as sodium aluminium silicate (Zeolite) and thereby making it unavailable for absorption (Thilsing-Hansen and Jørgensen Citation2001; Wilson Citation2001; Thilsing-Hansen et al. Citation2002, Citation2003, Citation2007), resulting in an activation of the calcium homeostatic mechanisms before calving.
Natural (Clinoptilolite) or synthetic Zeolites (Zeolite A) are crystals formed from a microporous aluminosilicate skeleton of alkali and alkaline earth cations which are encountered worldwide and having an infinite, open, three-dimensional structure. These materials have unique chemical and physical properties and are characterized by their ability to lose and gain water reversibly, to absorb substances with a suitable cross-sectional diameter (adsorption property) and to switch their cations with cations from their environment such as Ca2+, Mg2+, K+, and NH4 +, without major structural change (cation exchange capacity-CEC ≈ 220 mEq/100 g) (Filippidis et al. Citation1996; Bosi et al. Citation2002).
The facility of these supplements to liberate progressively ions in the rumen has created in the past 20 years an interest to use them as feed additives for ruminants, mainly in order to improve performance traits and for the prevention of certain metabolic diseases in dairy cattle. Recently, Zeolite has been approved as an additive in farm animals feeds by the European Committee at the highest inclusion rate of 2% of dry matter (European Commission Regulation Citation2005). The effectiveness of Clinoptilolite against intoxication by mycotoxins, as well as the increased interest for the use of feed additives that do not have residuals on the animal products, are expected to increase the use of Clinoptilolite as a feed additive (Valpotić et al. Citation2017; Benić et al. Citation2018).
Most of the studies concerning the use of Zeolite in the dairy cows ration were conducted during the lactation period and focused on the productive parameters (milk yield and composition) and ruminal environment. Among these studies we can mention, for example, those of Bosi et al. (Citation2002), Dschaak et al. (Citation2010), Sulzberger et al. (Citation2016) and Ðuričić et al. (Citation2017). For more details, an interesting review on the effects of Zeolite supplementation on dairy cow production and ruminal parameters was recently published by Khachlouf et al. (Citation2018).
On the other hand, there is a paucity of published information concerning the use of Zeolite during the critical periparturient period. Therefore, the current study was undertaken to evaluate the effect of introducing the calcium binding substance Zeolite in the dairy cow rations before and after calving on blood Ca, P, and Mg levels, as well as on milk production and composition during early lactation. It is also tested the hypothesis that Zeolite supplementation will benefit periparturient Ca status.
2. Materials and methods
2.1. Animals and diets
This experiment was conducted in a state farm in the region of Sfax (Tunisia). This farm adopts an aboveground farming system (Zero grazing) and applies the loose-housing systems. The trial took place from the 40 d before the expected date of calving until the 60 d of lactation. The cows were evenly allocated into two homogeneous groups: control group (CG) and experimental group (EG) kept in separate pens. Each treatment group consisted of 21 Holstein cows that calved between February and August 2016. The groups were balanced with respect to lactation number (20 primiparous + 22 multiparous), body weight (≈ 550 ± 25 kg), expected date of calving and milk production during the previous lactation (22.40 ± 6.32 kg/d for multiparous). The basic control diet for CG and EG consisted of oat hay, and alfalfa pellets during the dry period and oat hay, oat silage, and alfalfa pellets after calving. This daily ration was formulated to meet the energy (Forage Unit for Lactation: UFL), and the protein (Digestible Protein in the small Intestine: PDI) requirements of the pregnant and lactating cows according to INRA (Citation2007). The offered quantities were calculated to allow 10% orts (feed refused from a daily offering). The diets were supplemented with concentrates at a level of 6 kg per cow/d in dry period, and 12 kg per cow/d in early lactation. During these two periods, the total dry matter intake (DMI) was estimated 11.5, and 19.0 kg/cow per d, respectively. Additionally, cows in the dry period and lactation were supplemented with a commercial mineral balancer to meet the requirements of Ca, P, and Mg.
Cows were allowed ad libitum access to the basal ration and water, individually fed concentrate (06:00 and 16:00 h), and milked twice daily during lactation period (06:00 and 16:00 h).
The experimental diet (EG) was the same control ration, but additionally supplemented with 200 g/cow/d of Zeolite (sodium alumino silicate; Zeoline, B-4480 Engis, Belgium) thoroughly mixed into the morning meal concentrate of each cow. For this group, the adaptation period to the Zeolite diet started one week before launching the experiment. Cows remained in their treatment groups for the entire experiment. The different feed distributed to cows and their quantities are listed in . The chemical composition and nutritive values of the concentrate and basal diet used in this study are also presented in and .
Table 1. Quantities of food (kg/cow/day) distributed to control and experimental groups before and after calving.
Table 2. Ingredients, chemical composition and nutrient values of concentrate of control and experimental diets used in this study.
Table 3. Chemical composition and nutrient values of forages used in cow diet.
2.2. Milk production and composition
After calving, individual milk yield was recorded at days 7, 20, 40, and 60 post-calving during the morning and the evening milking. The controls coincided with those carried out by the Livestock and Grazing Office. Simultaneously, milk samples were collected from each cow at each milking, using aseptic pots of 100 ml capacity. Samples were held at 4°C in a cooler box to prevent milk spoilage during the transport to the laboratory. Representative sub-samples for mineral analysis (20 mL) were stored at −20°C and thawed at 4°C overnight before analysis.
Milk samples were analysed by an external collection centre in duplicate for total solids, fat, protein, and lactose using a near-infrared milk analyser (Milkoscan 6000; FOSS, Denmark) according to method 972.16 of AOAC (Citation1990).
Additionally, the flame atomic absorption spectrometric method was used for the determination of milk minerals (Ca, P, and Mg) after mineralization and dilution of the samples with nitric acid (AFNOR NF ISO 8070 Citation2007).
Finally, 3.5% fat corrected milk (FCM) was calculated by equation: (0.432 × milk yield) + (16.23 × milk fat yield) according to NRC (Citation2001).
2.3. Blood sampling
Throughout the experimental period, individual blood samples (7–8 ml) were collected in the morning before the morning feeding from the jugular vein into Vacutainer tubes, 40 and 20 days before the expected date of calving, and at approximately 72 h and 20 days postpartum. At the laboratory, blood plasma was separated by centrifugation (1500 × g for 15 min at 4°C) and stored at −18°C until the analyses were performed.
The plasma Ca was performed by the colorimetric 5-nitro-5′-methyl-(1,2-bis(o-aminophenoxy) ethan-N,N,N’,N’-tetraacetic acid (NM-BAPTA) method developed by Roche on c701/Cobas Integra 7000® analyser (NM-BAPTA, Roche Diagnostics, Mannheim, Germany). Briefly, the calcium NM-BAPTA is based on the NM-BAPTA molecule which reacts with calcium ions under alkaline conditions to form a complex, modifying the colour of the mixture. This complex reacts in a second step with EDTA and the reaction equilibrium shifts towards the calcium–EDTA complex and free NM-BAPTA. The change in absorbance, proportional to the calcium concentration is measured at 340 nm (Bourguignon et al. Citation2014).
The plasma inorganic P was also assessed on c701/Cobas Integra 7000® analyser. This method utilizes ammonium molybdate as the colour-forming reagent. Measurement of the final product occurs at 340 nm. Inorganic phosphate forms an ammonium phosphomolybdate complex having the formula (NH4)3[PO4 (MoO3)12] with ammonium molybdate in the presence of sulphuric acid. The concentration of phosphomolybdate formed is directly proportional to the inorganic phosphate concentration.
The Mg level was measured by the Chlorophosphonazo III colorimetric assay on Roche Cobas Integra 7000® automate. In this method magnesium reacts with Chlorophosphonazo III (CPZ III) and causes an increase in absorbance. EDTA is added to inhibit calcium interference. Addition of EDTA to the reaction allows for accurate sample blanking. The reaction is a two-point, end-point method that is measured photometrically at 660 nm.
2.4. Statistical analysis
All data were analysed by repeated measures ANOVA using the general linear model (GLM) procedure of SPSS version 18. The main effects included in the initial model were treatment diet (CG, EG), period, and treatment × period interactions. These data were compared at the significance level of P < .05.
3. Results and discussion
Descriptions and summary statistics of relevant measured variables for control (CG) and experiment (EG) groups are provided in . In addition to the treatment effect, period, and the interaction of treatment and period were also studied for all parameters.
Table 4. Milk and blood parameters from cows on each treatment.
3.1. Milk production and composition
The milk yield was significantly affected by treatment, lactation period and their interaction (). The dietary Zeolite addition resulted in a significant increase in milk production during the first two months of lactation (+2.18 kg/cow/d; P < .001; ). Owing to the interactive effect of treatment and period (P = .005; ; ), the difference between both groups was more pronounced at the end of the experimental period. Many researchers have proved that the dietary inclusion of Zeolite improves average of yield of dairy cows (Katsoulos et al. Citation2006; Ilić et al. Citation2011; Ural et al. Citation2013; Cruywagen et al. Citation2015). Generally, an increase in milk production can result from increased ruminal concentrations of propionate, increased postruminal digestion of starch, increased microbial protein synthesis, increased by-pass protein, or from a combination of these factors (Garcia-Lopez et al. Citation1992; Katsoulos et al. Citation2006).
Figure 1. Mean milk yield in Zeolite-treated cows (EG) and untreated control cows (CG).
Note: NS, nonsignificant; **P < .01.
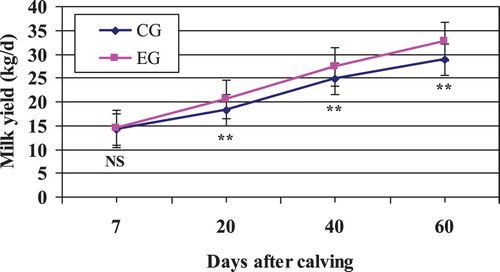
Others authors suggested that cows supplemented with Zeolite were able to convert feed into milk more efficiently (Cassida et al. Citation1988; Ural Citation2014; Sulzberger et al. Citation2016). The results obtained from the present study and aforementioned researchers indicated that the administration of Zeolite improves the energy status of the animals and may have positive influence on milk yield (Katsoulos et al. Citation2006; Karatzia et al. Citation2013).
Another explanation for increases in milk production might be the potential effect of Zeolite on the DCAD (dietary cation-anion difference) of diets fed to the cows. Previous experiments indicate that buffers increased DCAD and that DCAD and milk yield are closely related (Hu et al. Citation2007). It appears that the DCAD of the diet affects the acid-base balance regulation, which in turn increased DMI of dairy cows. This might explain a part of the increase in milk production.
In certain studies, the milk yield was not affected by Zeolite inclusion in the diet (Thilsing-Hansen et al. Citation2002; Katsoulos et al. Citation2006; Migliorati et al. Citation2007; Grabherr et al. Citation2009) although the cows showed a reduced feed intake and hypophosphataemia. It appears that at low level of Zeolite inclusion (1–1.4% on a DM basis), milk yield was not affected.
The discrepancies in results indicate that several factors influence milk production when incorporating Zeolite in the rations and that there may be limitations that need to be considered.
In our study, milk composition (total solids, fat, protein, lactose, and ash) were unaffected by Zeolite inclusion (, and ). These data are in agreement with results of other experiments using different sources of buffers (Cassida et al. Citation1988; Solorzano et al. Citation1989; Migliorati et al. Citation2007; Clark et al. Citation2009; Ðuričić et al. Citation2017).
Figure 2. Mean milk fat content in Zeolite-treated cows (EG) and untreated control cows (CG).
Note: NS, nonsignificant.
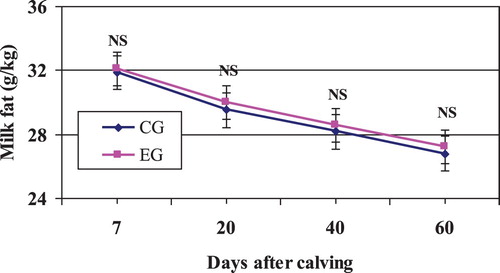
Figure 3. Mean milk protein content in Zeolite-treated cows (EG) and untreated control cows (CG).
Note: NS, nonsignificant.
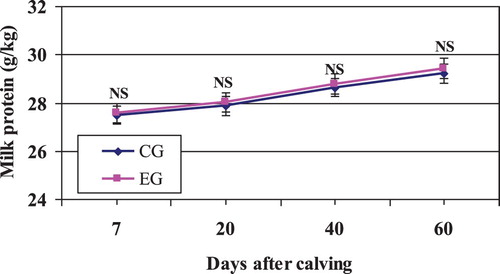
In contrast, other researchers reported that milk fat content increased or tended to increase when 1–2% of sodium sesquicarbonate or Zeolite was added to the diet (Cruywagen et al. Citation2015; Sulzberger et al. Citation2016). Ruminal buffers have been shown to prevent milk fat depression with rations based on corn silage or low-fibre diets (Harrison et al. Citation1989; Kennelly et al. Citation1999) by stabilizing rumen pH and thus offering a more suitable environment for microbial growth. Additionally, the buffer may increase ruminal outflow, increasing the acetate:propionate ratio and thus improving milk fat tests (Snyder et al. Citation1983; Cruywagen et al. Citation2015).
In general, it has been accepted that dietary buffers do not consistently alter protein percentage of milk (Cassida et al. Citation1988; Ghorbani et al. Citation1989; Katsoulos et al. Citation2006; Ðuričić et al. Citation2017) during early or midlactation. This trend was confirmed in our study (; ), suggesting that protein metabolism was unaffected by the addition of Zeolite.
However, Tucker et al. (Citation1994) and Dschaak et al. (Citation2010) reported that milk protein percentage was increased with Zeolite supplementation during the complete lactation. This difference did not appear until midlactation and was most apparent during late lactation. Solorzano et al. (Citation1989) noted that sodium sesquicarbonate increased digestibilities of CP (crude protein) and NDF (neutral detergent fibre). Addition of buffer may have increased AA (amino acid) supply to the mammary gland by promoting digestion of dietary proteins.
Finally, the FCM and the yields of fat, protein, and lactose were significantly affected by feeding system, lactation period, and the interaction of feeding system and lactation period, as a consequence of increasing milk yield in the Zeolite group ().
3.2. Milk minerals
Minerals such as Ca, P, and Mg play an important role in the structure and stability of casein micelles, and the susceptibility of the protein to aggregation during dairy processing (Holt and Jenness Citation1984; Gaucheron Citation2005). Especially, the calcium and phosphate contents are higher in milks rich in proteins. Also, Ca and P have a major influence on processing characteristics, such as rennet coagulation, heat stability, and ethanol stability (Tsioulpas et al. Citation2007; Sandra et al. Citation2012; Horne Citation2016). Apart from their effects on protein aggregation, variation in mineral content can also alter the nutritional value of milk and milk products (Cashman Citation2011; Pirilä et al. Citation2011).
Indeed, cow's milk and dairy products, widely consumed by children, are the best sources of Ca in human diet, helping to achieve adequate dietary Ca intake (Gao et al. Citation2005; Pirilä et al. Citation2011). Consequently, milk with higher Ca, P and Mg concentrations, is thus desirable.
3.2.1. Calcium
In our study, the range of Ca concentration for all milk samples collected over the experimental period (900–1290 mg/kg, data not shown) was lower in magnitude to that (1110–1470 mg/kg) reported by O’Brien et al. (Citation1999), Auldist et al. (Citation1999) and Gulati et al. (Citation2018). Variations in milk Ca content have been clearly related to the breed, season, stage of lactation, parity, and feed strategy (Nogalska et al. Citation2017; Gaignon et al. Citation2018). In addition, it is well established that the secretion of Ca into milk was regulated by mammary gland secretion of parathyroid hormone-related protein (PTHrP), bone accretion and resorption dynamics, the digestive tract, and kidneys (Boudon et al. Citation2016; Gaignon et al. Citation2018).
The lower milk Ca content in Tunisian dairy heard was most probably related to the intensive production system based on conserved grass (such as silage or hay), and high quantities of concentrates (Nogalska et al. Citation2017; Gaignon et al. Citation2018).
Zeolite supplementation had no significant effect on the amount of Ca exported in milk (P > .05; and ). In spite of the fact that blood Ca concentration in experimental cows was enhanced by Zeolite (see mineral blood section and ), it seems that the availability of blood Ca is not a determinant for Ca uptake by the mammary gland, at least under our experimental conditions.
Figure 4. Mean milk Ca content in Zeolite-treated cows (EG) and untreated control cows (CG).
Note: NS, nonsignificant.
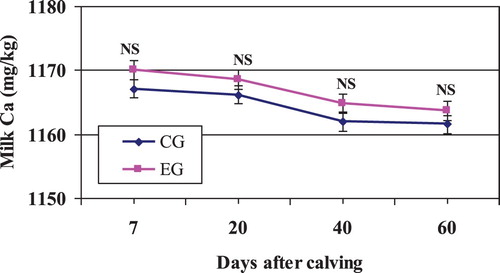
As expected, during the early weeks of lactation, most cows remain under the negative Ca balance (Gabryszczuk et al. Citation2010). Milk Ca content slightly decreased (not significantly) during the first days of lactation for both groups () as a consequence of highest milk production and lowest milk casein content in that period (Litwińczuk et al. Citation2004; Nogalska et al. Citation2017; Gaignon et al. Citation2018). According to Bijl et al. (Citation2013) and Gulati et al. (Citation2018), there is a significant positive correlation between milk Ca and protein content (or casein content: about 66% of Ca is intimately associated with casein). In this study, as it was mentioned before, milk protein was not affected by Zeolite addition (see ). This can explain the non-notable improvement in the milk Ca content observed here.
On the other hand, Boudon et al. (Citation2016) signalled that milk Ca content was highly correlated with DCAD, which was enhanced by Zeolite supplementation (Hu et al. Citation2007). Thus, the slight improvement in the milk Ca content in EG () was probably associated with increased DCAD and with decreased in the amount of Ca excreted in urine.
3.2.2. Phosphorus
The range of P content in all milk samples collected over the experimental period (750–1200 mg/kg, data not shown) was comparable in magnitude to that reported by Sola-Larrañaga and Navarro-Blasco (Citation2009) and Gulati et al. (Citation2018) (550–1120 mg/kg).
The concentration of P was not affected by treatment, period, and their interaction (P > .05; and ). Thus, the Zeolite supplementation was shown to have no significant effect on the milk P concentration over the experimental period. This result was expected because milk protein content was not enhanced in the current study (about 50% of P is intimately associated with casein according to Gaucheron Citation2005 and Petrera et al. Citation2016).
3.2.3. Magnesium
The range of Mg across all milks (98–132 mg/kg, data not shown) was of similar magnitude to that previously reported in the literature (Rodríguez-Rodríguez et al. Citation2001; Gaucheron Citation2005; Gulati et al. Citation2018) and stayed within the reference interval (100–150 mg/kg) suggested by Litwińczuk et al. (Citation2004). The mean Mg content was unaffected neither by treatment system nor by period (), and it remained stable along the experimental period (). The absence of effect of Zeolite on milk Mg content might be related to the non-change in milk protein content in the current study (Bijl et al. Citation2013; Gulati et al. Citation2018). These authors found that the content of this mineral had a significant positive correlation with protein content which was largely due to its association in the casein micelle.
3.3. Blood minerals
In this study, we also investigated whether the chosen calcium binding substance had any effect on the blood constituents normally linked to calcium homeostasis (Ca, P, and Mg) during the dry period and early lactation.
3.3.1. Calcium
Statistical analysis revealed a significant (P < .01) difference in plasma Ca level between the two groups before and after calving. It is also interesting to report that there was a significant interaction between treatment and period on blood Ca content ( and ). Blood analysis showed a linear decrease (P < .05) in concentrations of plasma Ca before and after calving. But, the drop in the plasma Ca level was more pronounced in CG than that in the EG. This is usually due to the feed, especially the dietary Ca, being insufficient to meet the heavy demand due to the rapidly growing foetus in dry period or milk production in early lactation.
Figure 7. Mean plasma Ca in Zeolite-treated cows (EG) and untreated control cows (CG).
Notes: The solid horizontal lines indicate the upper and lower limit of the reference interval. NS, nonsignificant; **P < .01; ***P < .001.
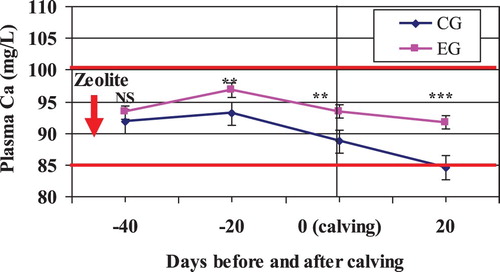
From looking at , it is obvious that Zeolite supplementation had a stabilizing effect on blood Ca around calving. The increase in plasma Ca level by Zeolite supplementation around calving was in agreement with Thilsing-Hansen and Jørgensen (Citation2001) and Thilsing-Hansen et al. (Citation2002, Citation2003).
In this herd, the mean plasma Ca level in both groups did, however, stay above the hypocalcaemia limit of 85.0 mg/L suggested by DesCoteaux et al. (Citation1997) and thus no cases of milk fever were recorded, and no cows were treated with calcium solutions intravenously.
The increased plasma calcium level closing to calving in the Zeolite-treated cows compared to untreated group is thought to result from an activation of the Ca homeostatic mechanisms before calving, rendering the cow ready for the sudden and massive draw on blood calcium around the time of calving (Thilsing-Hansen et al. Citation2002). Briefly, Zeolite supplementation was expected to decrease the availability of ration Ca below the requirements. This places the cow in negative Ca balance before calving. A drop in blood Ca stimulates parathyroid hormone (PTH) secretion from the parathyroid glands, which in turn increases renal reabsorption of Ca, enhances bone resorption and promotes renal vitamin D metabolism towards production of 1,25-dihydroxyvitamin D (1,25(OH)2D). This last also increases the intestinal absorption of Ca (Goff et al. Citation1991; Horst et al. Citation1997).
The activation of the calcium homeostatic mechanisms in Zeolite group before calving could also be related to the increase in the dietary cation-anion difference (DCAD) by Zeolite supplementation. It is now well known that blood Ca concentration is negatively associated with DCAD (Charbonneau et al. Citation2006; Boudon et al. Citation2016; Goff and Koszewski Citation2018).
Combined, our results are a confirmation of prior research that reports the effectiveness of including Zeolite in the rations of dry dairy cows to activate the calcium homeostatic mechanisms before calving and thus to avoid postpartum hypocalacemia.
3.3.2. Phosphorus
In our study, the plasma P content did not differ between treatments before calving as well as post calving ( and ). In both groups, the mean plasma inorganic phosphate was above the lower limit of the reference interval (55–65 mg/L) given by Kaneko et al. (Citation1997).
Figure 8. Mean plasma P in Zeolite treated cows (EG) and untreated control cows (CG).
Notes: The solid horizontal lines indicate the upper and lower limit of the reference interval. NS, nonsignificant.
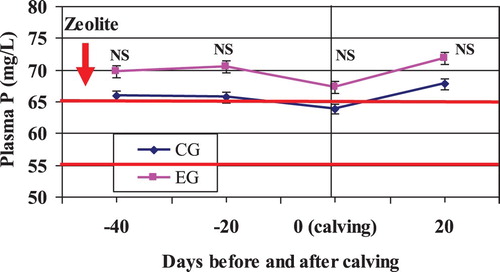
As expected, plasma phosphate in both groups was decreased close to calving (P = .087). The hypophosphatemia normally seen in parturient cows can be explained by a higher level of circulating PTH at this point and thereby an increased salivary and renal excretion of phosphate (Kaneko et al. Citation1997). In the present study, the drop in plasma phosphate in Zeolite-treated cows and the untreated control cows was only transient, since the phosphate level had normalized within 3 weeks after calving ().
Unlike our results, Thilsing-Hansen et al. (Citation2002, Citation2003) saw a lower mean postpartum plasma P concentration in cows fed Zeolite and explained their results by a decreased bioavailability of dietary P by the Zeolite due to formation of insoluble aluminium phosphate complexes in the intestinal lumen.
3.3.3. Magnesium
From and , plasma Mg concentration was not different between treatments during the entire experimental period. The mean plasma Mg level in both groups stayed within the reference interval (18–24 mg/L) suggested by Kaneko et al. (Citation1997). Because blood magnesium concentration usually reflects dietary magnesium intake (Goff Citation2008), it could be concluded that normal plasma magnesium in this study was attributed to the correct feed intake.
Figure 9. Mean plasma Mg in Zeolite-treated cows (EG) and untreated control cows (CG).
Notes: The solid horizontal lines indicate the upper and lower limit of the reference interval. NS, nonsignificant.
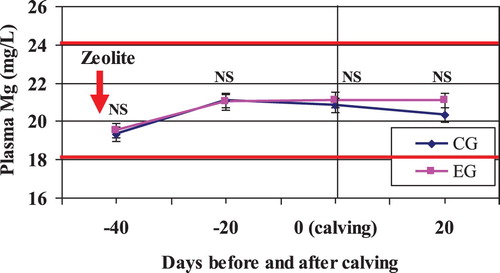
Somewhat surprisingly, the plasma Mg content was significantly higher around calving than that of the beginning of the dry period (period effect). According to Kaneko et al. (Citation1997) and Thilsing-Hansen et al. (Citation2002, Citation2003), the plasma Mg concentration is less well controlled than that of Ca and primarily is a result of balance between ruminal and intestinal absorption and renal excretion. The hypermagnesemia seen around calving may be connected to the concurrent drop in blood Ca observed in this study. The mechanism of hypermagnesemia in cows with hypocalcaemia is steel unknown, but increased renal reabsorption of Mg by the renal tubules induced by PTH (secreted in response to hypocalcaemia) could play a role (Riond et al. Citation1995; Goff and Koszewski Citation2018).
4. Conclusion
These results indicate that 200 g/d of Zeolite can be effectively included in the rations of lactating dairy cows without any adverse effects on milk composition or milk minerals. Our findings, however, indicate that the rations formulated with Zeolite increased milk yield for cows and consequently enhanced FCM, milk fat, milk protein, and lactose yields. Additionally, prepartum Zeolite supplementation significantly increased the plasma Ca level before and after calving. Combined, our results are a confirmation of prior research that reports the effectiveness of including Zeolite in the rations of dry dairy cows to activate the calcium homeostatic mechanisms before calving and thus to prevent against parturient hypocalcaemia. These findings indicate promising prospects for the utilization of Zeolite, which is often more economical than traditional buffer sources.
Disclosure statement
No potential conflict of interest was reported by the authors.
ORCID
Houda Hamed http://orcid.org/0000-0002-0650-3915
Radhouane Gdoura http://orcid.org/0000-0003-1140-3780
Ahmed Gargouri http://orcid.org/0000-0002-9703-2476
References
- AFNOR NF ISO 8070 . 2007. Lait et produits laitiers – Détermination des teneurs en calcium, sodium, potassium et magnésium – Méthode spectrométrie par absorption atomique.
- Allen WM , Sansom BF. 1993. Parturient paresis (milk fever) and hypocalcemia (cows, ewes, and goats). In: Howard JL , editor. Current veterinary therapy: Food animal practice. Philadelphia : WB Saunders; p. 304–309.
- AOAC . 1990. Association of Official Analytical Chemists. Official methods of analysis, AOAC. Helrich K, editor. 15th ed. Arlington, VA, USA.
- Auldist MJ , Johnston KA , White NJ , Fitzsimons WP , Boland MJ. 1999. A comparison of the composition, coagulation characteristics and cheesemaking capacity of milk from Friesian and Jersey dairy cows. J Dairy Res. 71:51–57. doi: 10.1017/S0022029903006575
- Benić M , Maćešić N , Cvetnić L , Habrun B , Cvetnić Ž , Turk R , Đuričić D , Lojkić M , Dobranić V , Valpotić H , et al. 2018. Bovine mastitis: a persistent and evolving problem requiring novel approaches for its control – a review. Veterinarski arhiv. 88:535–557. doi: 10.24099/vet.arhiv.0116
- Bijl E , van Valenberg HJF , Huppertz T , van Hooijdonka CM. 2013. Protein, casein, and micellar salts in milk: current content and historical perspectives. J Dairy Sci. 96:5455–5464. doi: 10.3168/jds.2012-6497
- Bosi P , Creston D , Casin L. 2002. Production performance of dairy cows after the dietary addition of clinoptilolite. Ital J Anim Sci. 1:187–195. doi: 10.4081/ijas.2002.187
- Boudon A , Johan M , Narcy A , Boutinaud M , Lamberton P , Hurtaud C. 2016. Dietary cation-anion difference and day length have an effect on milk calcium content and bone accretion of dairy cows. J Dairy Sci. 99:1527–1538. doi: 10.3168/jds.2015-9664
- Bourguignon C , Dupuy AM , Coste T , Michel F , Cristol JP. 2014. Evaluation of NM-BAPTA method for plasma total calcium measurement on Cobas 8000®. Clin Biochem. 47:636–639. doi: 10.1016/j.clinbiochem.2013.12.027
- Cashman KD. 2011. Milk salts: macroelements, nutritional significance. In: Fuquay JW , Fox PF , McSweeney PLH , editors. Encyclopedia of dairy sciences. Vol. 3. London, UK : Academic Press; p. 925–932.
- Cassida KA , Muller LD , Sweeney TF. 1988. Sodium sesquicarbonate for early lactation dairy cows fed corn silage-based diets. J Dairy Sci. 71:381–387. doi: 10.3168/jds.S0022-0302(88)79567-3
- Charbonneau E , Pellerin D , Oetzel GR. 2006. Impact of lowering dietary cation-anion difference in nonlactating dairy cows: a meta-analysis. J Dairy Sci. 89:537–548. doi: 10.3168/jds.S0022-0302(06)72116-6
- Clark JH , Christensen RA , Bateman HG 2nd , Cummings KR. 2009. Effects of sodium sesquicarbonate on dry matter intake and production of milk and milk components by Holstein cows. J Dairy Sci. 92:3354–3363. doi: 10.3168/jds.2008-1995
- Cruywagen CW , Taylor S , Beya MM , Calitz T. 2015. The effect of buffering dairy cow diets with limestone, calcareous marine algae, or sodium bicarbonate on ruminal pH profiles, production responses, and rumen fermentation. J Dairy Sci. 98:5506–5514. doi: 10.3168/jds.2014-8875
- DesCoteaux L , Krainock R , Lissemore K. 1997. Evaluation of new diagnostic tests to predict hypocalcaemia in mature dairy cows. Bovine Pract. 30:186–187.
- Dschaak CM , Eun JS , Young AJ , Stott RD , Peterson S. 2010. Effects of supplementation of natural zeolite on intake, digestion, ruminal fermentation, and lactational performance of dairy cows. Prof Anim Sci. 26:647–654. doi: 10.15232/S1080-7446(15)30662-8
- Ðuričić D , Benić M , Maćešić N , Valpotić H , Turk R , Dobranić V , Cvetnić L , Gračner D , Vince S , Grizelj J , et al. 2017. Dietary zeolite clinoptilolite supplementation influences chemical composition of milk and udder health in dairy cows. Veterinarska Stanica. 48:257–265.
- European Commission Regulation . 2005. European Commission Regulation No. 1810/2005 of 4 November 2005 concerning a new authorisation for 10 years of an additive in feedingstuffs, the permanent authorisation of certain additives in feedingstuffs and the provisional authorisation of new uses of certain additives already authorised in feedingstuffs. Official Journal of the European Union. L&C L291(5):1–7.
- Filippidis A , Godelitsas A , Charistos D , Misaelides P , Kassoli-Fournaraki A. 1996. The chemical behaviour of natural zeolites in aqueous environments: interactions between low-silica zeolites and 1M NaCl solutions of different initial pH-values. Appl Clay Sci. 11:199–209. doi: 10.1016/S0169-1317(96)00025-7
- Gabryszczuk M , Słoniewski K , Metera E , Sakowski T. 2010. Content of mineral elements in milk and hair of cows from organic farms. J Elem. 15:259–267.
- Gaignon P , Gelé M , Hurtaud C , Boudon A. 2018. Characterization of the nongenetic causes of variation in the calcium content of bovine milk on French farms. J Dairy Sci. 101:4554–4569. doi: 10.3168/jds.2017-14043
- Gao X , Valley ML , Tucker KL. 2005. Prospective studies of dairy product and calcium intakes and prostate cancer risk: a meta-analysis. J Natl Cancer Instut. 97:1768–1777. doi: 10.1093/jnci/dji402
- Garcia-Lopez R , Elias A , Menchaca MA. 1992. The utilization of zeolite by dairy cows. 2. Effect on milk yield. Cuban J Agric Sci. 26:131–133.
- Gaucheron F. 2005. The minerals of milk. Reprod Nutr Dev. 45:473–483. doi: 10.1051/rnd:2005030
- Ghorbani GR , Jackson JA , Hemken RW. 1989. Effects of sodium bicarbonate and sodium sesquicarbonate on animal performance, ruminal metabolism, and systemic acid-base status. J Dairy Sci. 72:2039–2045. doi: 10.3168/jds.S0022-0302(89)79327-9
- Goff JP. 2006. Macromineral physiology and application to the feeding of the dairy cow for prevention of milk fever and other periparturient mineral disorders. Anim Feed Sci Technol. 126:237–257. doi: 10.1016/j.anifeedsci.2005.08.005
- Goff JP. 2008. The monitoring, prevention, and treatment of milk fever and subclinical hypocalcemia in dairy cows. Vet J. 176:50–57. doi: 10.1016/j.tvjl.2007.12.020
- Goff JP , Koszewski NJ. 2018. Comparison of 0.46% calcium diets with and without added anions with a 0.7% calcium anionic diet as a means to reduce periparturient hypocalcemia. J Dairy Sci. 101:1–13. doi: 10.3168/jds.2017-12962
- Goff JP , Reinhardt TA , Horst RL. 1991. Enzymes and factors controlling vitamin D metabolism and action in normal and milk fever cows. J Dairy Sci. 74:4022–4032. doi: 10.3168/jds.S0022-0302(91)78597-4
- Grabherr H , Spolders M , Lebzien P , Huther L , Flachowsky G , Fürll M , Grun M. 2009. Effect of Zeolite A on rumen fermentation and phosphorus metabolism in dairy cows. Arch Anim Nutr. 63:321–336. doi: 10.1080/17450390903020430
- Gulati A , Galvin N , Lewis E , Hennessy D , O’Donovan M , McManus JJ , Fenelon MA , Guinee TP. 2018. Outdoor grazing of dairy cows on pasture versus indoor feeding on total mixed ration: Effects on gross composition and mineral content of milk during lactation. J Dairy Sci. 101:2710–2723. doi: 10.3168/jds.2017-13338
- Harrison JH , Riley RE , Loney KA. 1989. Effect of type and amount of buffer addition to grass silage-based total mixed rations on milk production and composition. J Dairy Sci. 72:1824–1830. doi: 10.3168/jds.S0022-0302(89)79299-7
- Holt C , Jenness R. 1984. Interrelationships of constituents and partition of salts in milk samples from eight species. Comp Biochem Physiol A Comp Physiol. 77:275–282. doi: 10.1016/0300-9629(84)90060-4
- Horne DS. 2016. Ethanol stability and milk composition. In: McSweeney PLH , O’Mahony JA , editors. Advanced dairy chemistry. Vol 1B: proteins: applied aspects. 4th ed. New York, NY : Springer; p. 225–246.
- Horst RL , Goff JP , Reinhardt TA , Buxton DR. 1997. Strategies for preventing milk fever in dairy cattle. J Dairy Sci. 80:1269–1280. doi: 10.3168/jds.S0022-0302(97)76056-9
- Hu W , Murphy MR , Constable PD , Block E. 2007. Dietary cation-anion difference and dietary protein effects on performance and acid-base status of dairy cows in early lactation. J Dairy Sci. 90:3355–3366. doi: 10.3168/jds.2006-514
- Ilić Z , Petrović MP , Pešev S , Stojković J , Ristano B. 2011. Zeolite as a factor in the improvement of some production traits of dairy cattle. Biotech Anim Husb. 27:1001–1007. doi: 10.2298/BAH1103001I
- INRA . 2007. Alimentation des Bovins Ovins Caprins: Besoins des Animaux et Tables des Aliments. Versailles, France : Editions QUAE.
- Kaneko JJ , Harvey JW , Bruss ML. 1997. Clinical biochemistry of domestic animals, 5th ed. San Diego, CA : Academic Press.
- Karatzia MA , Katsoulos PD , Karatzias H. 2013. Diet supplementation with clinoptilolite improves energy status, reproductive efficiency and increases milk yield in dairy heifers. Anim Prod Sci. 53:234–239. doi: 10.1071/AN11347
- Katsoulos PD , Panousis N , Roubies N , Christaki E , Arsenos G , Karatzias H. 2006. Effects of long-term feeding of a diet supplemented with clinoptilolite to dairy cows on the incidence of ketosis, milk yield, and liver function. Vet Rec. 159:415–418. doi: 10.1136/vr.159.13.415
- Kennelly JJ , Robinson B , Khorasani GR. 1999. Influence of carbohydrate source and buffer on rumen fermentation characteristics, milk yield, and milk composition in early-lactation Holstein cows. J Dairy Sci. 82:2486–2496. doi: 10.3168/jds.S0022-0302(99)75500-1
- Khachlouf K , Hamed H , Gdoura R , Gargouri A. 2018. Effects of Zeolite supplementation on dairy cow production and ruminal parameters – a review. Ann Anim Sci. doi:10.2478/aoas-2018-0025.
- Litwińczuk A , Litwińczuk Z , Barłowska J , Florek M. 2004. Animal materials – evaluation and usage. Warszawa : PWRiL; p. 216–233 (in Polish).
- Migliorati L , Abeni F , Cattaneo MP , Tornielli C , Pirlo G. 2007. Effects of adsorbents in dairy cow diet on milk quality and cheese-making properties. Ital J Anim Sci. 6:460–462.
- Nefzaoui A , Chermiti A. 1989. Composition chimique et valeur nutritive pour les ruminants des fourrages et concentrés d’origine tunisienne. Annales de l’INRAT. 62:1–16.
- Nogalska A , Momot M , Sobczuk-Szul M , Pogorzelska-Przybyłek P , Nogalski Z. 2017. Calcium and magnesium contents in the milk of high-yielding cows. J Elem. 22:809–815.
- NRC . 2001. National research council. Nutrient requirements of dairy cattle, 7th rev. ed. Washington, DC : National Academy Press.
- O’Brien B , Mehra R , Connolly J , Harrington D. 1999. Seasonal variation in the composition of Irish manufacturing and retail milks: 4. Minerals and trace elements. Ir J Agric Food Res. 38:87–99.
- Petrera F , Catillo G , Napolitano F , Malacarne M , Franceschi P , Summer A , Abeni F. 2016. New insights into the quality characteristics of milk from Modenese breed compared with Italian Friesian. Ital. J Anim Sci. 15:559–567.
- Pirilä S , Taskinen M , Viljakainen H , Kajosaari M , Turanlahti M , Saarinen-Pihkala UM , Mäkitie O. 2011. Infant milk feeding influences adult bone health: a prospective study from birth to 32 years. PLoS One. 6:e19068. doi: 10.1371/journal.pone.0019068
- Riond JL , Kocabagli N , Spichiger UE , Wanner M. 1995. The concentration of ionized magnesium in serum during the periparturient period of non-paretic dairy cows. Vet Res Comm. 19:195–203. doi: 10.1007/BF01839298
- Risco CA , Reynolds JP , Hird D. 1984. Uterine prolapse and hypocalcemia in dairy cows. J Am Vet Med Assoc. 185:1517–1519.
- Rodríguez-Rodríguez EM , Sanz Alaejos M , Diaz Romero C. 2001. Mineral concentrations in cow’s milk from the Canary Island. J Food Com Anal. 14:419–430. doi: 10.1006/jfca.2000.0986
- Sandra S , Ho M , Alexander M , Corredig M. 2012. Effect of soluble calcium on the renneting properties of casein micelles as measured by rheology and diffusing wave spectroscopy. J Dairy Sci. 95:75–82. doi: 10.3168/jds.2011-4713
- Shearer JK , van Horn HH. 1992. Metabolic diseases of dairy cattle. In: van Horn HH , Wilcox CJ , editors. Large dairy herd management. Champaign, IL : American Dairy Science Association; p. 358–372.
- Smith BI , Risco CA. 2005. Management of periparturient disorders in dairy cattle. Vet Clin North Am Food Anim Pract. 21:503–521. doi: 10.1016/j.cvfa.2005.02.007
- Snyder TJ , Rogers JA , Muller LD. 1983. Effects of 1.2 percent sodium bicarbonate with two ratios of corn silage:grain on milk production, rumen fermentation and nutrient digestion by lactating cows. J Dairy Sci. 66:1290–1297. doi: 10.3168/jds.S0022-0302(83)81937-7
- Sola-Larrañaga C , Navarro-Blasco I. 2009. Chemometric analysis of minerals and trace elements in raw cow milk from the community of Navarra, Spain. Food Chem. 112:189–196. doi: 10.1016/j.foodchem.2008.05.062
- Solorzano LC , Armentano LE , Grummer RR , Dentine MR. 1989. Effects of sodium bicarbonate or sodium sesquicarbonate on lactating Holsteins fed a high grain diet. J Dairy Sci. 72:453–461. doi: 10.3168/jds.S0022-0302(89)79127-X
- Stevenson JS , Call EP. 1988. Reproductive disorders in the periparturient dairy cow. J Dairy Sci. 71:2572–2583. doi: 10.3168/jds.S0022-0302(88)79846-X
- Sulzberger SA , Kalebich CC , Melnichenko S , Cardoso FC. 2016. Effects of clay after a grain challenge on milk composition and on ruminal, blood, and fecal pH in Holstein cows. J Dairy Sci. 99:8028–8040. doi: 10.3168/jds.2016-11030
- Thilsing-Hansen T , Jørgensen RG. 2001. Prevention of parturient paresis and subclinical hypocalcaemia in dairy cows by zeolite A administration in the dry period. J Dairy Sci. 84:691–693. doi: 10.3168/jds.S0022-0302(01)74523-7
- Thilsing-Hansen T , Jørgensen RJ , Enemark JMD , Larsen T. 2002. The effect of zeolite A supplementation in the dry period on periparturient calcium, phosphorus and magnesium homeostasis. J Dairy Sci. 85:1855–1862. doi: 10.3168/jds.S0022-0302(02)74259-8
- Thilsing-Hansen T , Jørgensen RJ , Enemark JMD , Zelvyte R , Sederevicius A. 2003. The effect of zeolite A supplementation in the dry period on blood mineral status around calving. Acta Vet Scand Suppl. 97:87–95.
- Thilsing-Hansen T , Larsen T , Jørgensen RJ , Houe H. 2007. The effect of dietary calcium and phosphorus supplementation in zeolite A treated cows on parturient calcium and phosphorus homeostasis. J Vet Med A. 54:82–91. doi: 10.1111/j.1439-0442.2007.00887.x
- Tsioulpas A , Lewis MJ , Grandison AS. 2007. Effect of minerals on casein micelle stability of cows’ milk. J Dairy Res. 74:167–173. doi: 10.1017/S0022029906002330
- Tucker WB , Shin IS , Hogue JF , Aslam M , Adams GD , Van Koevering MT , Vernon RK , Cummings KR. 1994. Natural sodium sesquicarbonate fed for an entire lactation: influence on performance and acid-base status of dairy cows. J Dairy Sci. 77:3111–3117. doi: 10.3168/jds.S0022-0302(94)77253-2
- Ural DA. 2014. Efficacy of clinoptilolite supplementation on milk yield and somatic cell count. Rev MVZ Córdoba. 19:4242–4248. doi: 10.21897/rmvz.86
- Ural DA , Cengiz O , Ural K. 2013. Dietary clinoptilolite addition as a factor for the improvement of milk yield in dairy cows. J Anim Vet Adv. 12:140–145.
- Valpotic H , Valpotić H , Gračner D , Turk R , Đuričić D , Vince S , Folnožić I , Lojkić M , Žura Žaja I , Bdrica L , et al. 2017. Zeolite clinoptilolite nanoporous feed additive for animals of veterinary importance: potentials and limitations. Period biol. 119:159–172. doi: 10.18054/pb.v119i3.5434
- Wilson GF. 2001. A novel nutritional strategy to prevent milk fever and stimulate milk production in dairy cows. N Z Vet J. 49:78–80. doi: 10.1080/00480169.2001.36207