ABSTRACT
The immunoglobulin G (IgG) antibody is important for detecting and evaluating immune responses. Despite increasing opportunities for tigers to be exposed to emerging diseases due to global changes coupled with increasing interaction with humans, there is no available antibody specific to tigers. Aims of this study were to produce a rabbit anti-Bengal tiger (Panthera tigris tigris) IgG polyclonal antibody and to determine its specificity. The molecular weight of the purified Bengal tiger IgG was approximately 170 kDa. The cross-reactivity of rabbit anti-Bengal tiger IgG to IgG of other wild felid species and other domestic animals was analyzed by indirect enzyme-linked immunosorbent assay. The cross reactivity to other related wild animals ranged from 53.69% to 75.63%. There was high cross-reactivity with domestic cats (63.58%) and pigs (38.45%) and low cross-reactivity with domestic cows (10.46%), goats (8.60%), sheep (7.25%), and chickens (6.91%). To confirm cross-reactivity with domestic animals’ IgG, western blotting was performed. These results indicated that the purified rabbit anti-Bengal tiger IgG polyclonal antibody strongly reacted with species in the family Felidae. The polyclonal antibody generated in this study has the potential to aid in the development of useful tools for further novel immunological investigations on tigers and related felid species.
Introduction
Tigers (Panthera tigris) are important members of their ecosystem, and their state indicates the health of the ecosystem (Sanderson et al. Citation2010). The International Union for Conservation of Nature (IUCN) Red List of Threatened Species divides the tiger into six extant subspecies based on the distinctive molecular genetic markers identified by Luo et al (Citation2004). It has been estimated that there are only approximately 3200 tigers remaining in the wild globally (Goodrich et al. Citation2015). Despite significant regional and international support for conservation of these magnificent beasts, the tiger population in the wild has been decreasing due to habitat loss and prey depletion caused by intense poaching (Dinerstein et al. Citation2007). Consequently, the tiger has been listed not only as an endangered species by the IUCN but also as being at a crisis point (Sanderson et al. Citation2010; Goodrich et al. Citation2015).
Presently, tigers in zoological parks are susceptible to several infectious diseases, including canine distemper (CDV) (Appel et al. Citation1994; Nagao et al. Citation2012), feline panleukopenia virus (FPV) (Duarte et al. Citation2009; Dissanayake et al. Citation2017), feline calicivirus (FCV) (Tian et al. Citation2016), Cytauxzoon felis (Jakob and Wesemeier Citation1996; Lewis et al. Citation2012), Toxoplasma gondii (Yang et al. Citation2017) and even avian influenza (Keawcharoen et al. Citation2004; Hu et al. Citation2016). One explanation for the recent emergence of infectious diseases is that global changes coupled with human activities have potentially altered the interactions between the disease components (Daszak et al. Citation2000; de La Rocque et al. Citation2008; Harvell et al. Citation2009; Gallana et al. Citation2013; Lindahl and Grace Citation2015). Hence, endangered species will have a tendency to acquire virulent pathogens, which would cause a decline in their number or an increase in the number of species becoming extinct (McCallum and Dobson Citation1995). Vaccination could protect such animals from infectious diseases. Moreover, it could prevent disease transmission to humans or animals, protect animal health, and prevent economic losses (Cross et al. Citation2007; Plumb et al. Citation2007).
In the past several decades, vaccination of tigers has been practiced in zoological parks in accordance with the vaccinations recommended for exotic cats by Bittle (Citation1993). A previous study, however, reported the deaths of captive tigers despite vaccination (Wang et al. Citation2017). However, evidence with regard to the efficacy of the vaccine was not demonstrated in that case. Antibody titres of the vaccine might help to resolve the issue. Likewise, antibody titres of pathogens might also help in the detection of disease in future surveillance and epidemiological studies. Unfortunately, current serological assays to evaluate immune responses in tigers are restricted to seroneutralization (Nagao et al. Citation2012; Risi et al. Citation2012; Sadler et al. Citation2016) and hemagglutination assays (Hu et al. Citation2016; Yang et al. Citation2017) due to a lack of suitable detecting antisera (Anti-tiger immunoglobulin: Ig). It is interesting to note that an anti-Asian elephant IgG antibody was recently produced for endangered Asian elephants (Elephas maximus) for the development of serological diagnostic tests (Kania et al. Citation1997; Vongchan Citation2013; Humphreys et al. Citation2015). To address the limitations of serological diagnostic tools for tigers, alternative serological assays urgently need to be developed. To the best of our knowledge, specific serology assays for the detection of tiger IgG antibody have not yet been reported. Therefore, the present study aimed to produce a rabbit polyclonal anti-tiger IgG antibody and to determine its specificity against the IgG of Bengal tigers and other species.
Materials and methods
Animal sera
Sera of wild felid species, including the orange-haired and white-haired Bengal tiger (Panthera tigris tigris), snow-white tiger (Panthera tigris), golden tabby tiger (Panthera tigris), Siberian tiger (Panthera tigris altaica), panther (Panthera pardus), leopard (P. pardus), jaguar (Panthera onca), lion (Panthera leo), white lion (P. leo), clouded leopard (Neofelis nebulosa), Asian golden cat (Catopuma temminckii), leopard cat (Prionailurus bengalensis), and jungle cat (Felis chaus), were obtained from the sera bank of Tiger Kingdom, Chiang Mai Night Safari, and Chiang Mai Zoo, Thailand. Furthermore, sera of the domestic species provided by the sera bank of the Veterinary Diagnostic Laboratory Center, Faculty of Veterinary Medicine, Chiang Mai University were also used; these were sera of the domestic cat (Felis catus), pig (Sus scrofa domesticus), cow (Bos taurus), chicken (Gallus gallus domesticus), sheep (Ovis aries), and goat (Capra hircus).
Animal immunoglobulin G (IgG) preparation
Pooled sera of each animal species were subjected to IgG isolation and purification using the MelonTM Gel IgG Spin Purification Kit (Pierce Biotechnology, Rockford, USA) according to the manufacturer's instructions. The concentration of IgG was measured by UV spectrophotometry at 280 nm and analyzed by sodium dodecyl sulfate polyacrylamide gel electrophoresis (SDS-PAGE). The purified IgG was adjusted to a concentration of 2 mg/ml and then kept as an aliquot at −20°C until further analysis.
Rabbit anti-Bengal tiger IgG polyclonal antibody production
The purified orange-haired Bengal tiger IgG from the previous step was mixed with an equal volume of Montanide (Seppic, Paris, France; 1:1 v/v). Three New Zealand White rabbits (mixed gender and age) were subcutaneously immunized with purified tiger IgG in Montanide (100 µg/dose 1 ml) at one-week intervals. Before each immunization, 2 ml of blood was collected from the saphenous veins, and the antibody titre was determined weekly using an indirect enzyme-linked immunosorbent assay (ELISA). The immunization was terminated when the optical density (OD) values reached 2.5000. The indirect ELISA assay was carried out as previously described by Vongchan (Citation2013). Briefly, 96-well immunoplates (Nunc-Immuno Plate MaxiSorp, Intermed, Roskildes, Denmark) were coated with purified orange-haired Bengal tiger IgG (10 µg/ml in 0.05 M carbonate/bicarbonate buffer pH 9.6) and then incubated overnight at 4°C. The plates were washed three times with phosphate buffer saline (PBS) containing 0.05% Tween-20 (PBST) and blocked with a blocking buffer (5% skim milk in PBS). Serial 10-fold dilutions of pre-immunized and post-immunized rabbit sera were added to the blocking buffer and incubated at 37°C for 1 hr. The plates were developed with secondary goat anti-rabbit IgG horseradish peroxidase (HRP) conjugated antibody (Dako Cytomation, Glostrup, Denmark; 1:2000 dilution) and incubated again at 37°C for 1 hr. The reaction was revealed by the addition of 3,3′,5,5′-tetramethybenzidine (TMB) substrate (SeraCare Life Sciences, Gaithersburg, MD, USA), and the plates were incubated at room temperature for 15 min away from the light. Finally, the reaction was stopped with 2 N H2SO4, and the optical density (OD) was measured spectrophotometrically by using the Accu Reader Microplate reader M965 (Metertech, Taipei, Taiwan R.O.C.) at 450 nm.
One week after the last immunization, the final blood sample was collected by exsanguination from the jugular vein. Then, the rabbit antisera were isolated and purified using the MelonTM Gel IgG Purification Kit according to the manufacturer's instructions. The rabbit anti-Bengal tiger IgG polyclonal antibody concentration was measured by the BCA method and analyzed by SDS-PAGE. The purified rabbit anti-Bengal tiger IgG polyclonal antibody was adjusted to 2 mg/ml, and then the aliquot was stored at −20°C for further analysis.
Sodium dodecyl sulfate polyacrylamide gel electrophoresis (SDS-PAGE) analysis for immunoglobulin G detection
Either purified animals’ IgG antibody or rabbit anti-Bengal tiger IgG antibody were subsequently analyzed by SDS-PAGE according to Laemmli (Citation1970). Briefly, the samples were solubilized in SDS-PAGE sample buffer (50 mM Tris, 20% glycerol, 0.005% bromophenol blue, 4% SDS) with or without 5% β-mercaptoethanol as a reducing agent, boiled at 95°C for 5 min, and then separated electrophoretically on a 10% SDS-PAGE gel using the Mini-PROTEAN® electrophoresis system (Bio-Rad Laboratories, Hercules, CA, USA). The separated proteins were stained with Coomassie Brilliant Blue R-250 (Sigma-Aldrich) for protein band detection.
Evaluation of binding capacity of rabbit anti-Bengal tiger IgG using western blot analysis
The separated proteins of purified animal species’ IgG were electrically transferred onto a nitrocellulose membrane (Merck Millipore™, Merck KGaA©, Darmstadt, DEU) using a western blot apparatus as described by Towbin et al. (Citation1979). The blotting time was 60 min at a constant voltage of 10 V. The membranes were blocked with a blocking buffer (1% bovine serum albumin (BSA) in PBS) for 1 hr at room temperature. After washing three times with PBST, the membrane was probed with rabbit anti-Bengal tiger IgG polyclonal antibody and incubated with gentle rocking at room temperature for 1 hr. The membrane was washed three times again and subsequently incubated with HRP-conjugated goat anti-rabbit IgG (1:2000 dilution) for 1 hr at room temperature. Finally, the reactions were visualized using a solution containing 3,3′-diaminobenzidine tetrahydrochloride (DAB; Invitrogen, Carlsbad, CA, USA) and hydrogen peroxide (H2O2; Merck, Germany).
Optimization of concentration of reagents for indirect ELISA testing
To determine the optimum concentration of the coating antigen, sera and conjugate, a checkerboard titration was performed according to Crowther (Citation2001). Ninety-six well immunoplates (Nuncimmuno™ plate, Denmark) were coated with different concentrations of purified orange-haired Bengal tiger IgG (2.0, 1.0 and 0.5 µg/ml) in 0.05 M carbonate/bicarbonate buffer (pH 96). The plates were incubated overnight at 4°C, washed with 150 µl/well of PBST (pH 72) three times, and blocked with 200 µl/well of the blocking buffer followed by incubation at 37°C for 1 hr and subsequent washing three times with PBST.
Thereafter, purified rabbit anti-Bengal tiger IgG was serially diluted ten-fold in the blocking buffer 1:108 A volume of 100 µl of each diluted serum was added to each well as a primary antibody and then incubated at 37°C for 1 hr. After washing three times with PBST, the plate was incubated with 50 µl/well of HRP-conjugated goat anti-rabbit IgG as a secondary antibody at dilutions of 1:2000, 1:3000, 1:4000, 1:5000 and 1:6000, and then incubated at 37°C for 1 hr. The plate was washed with PBST three times, and 100 µl/well of the TMB substrate was added to each well and incubated at room temperature under light protection conditions for 10–30 min. Finally, 2 N H2SO4 was added to the plates to stop the reaction, and the OD value at 450 nm was read by an automatic ELISA plate reader.
Determination of rabbit anti-Bengal tiger IgG polyclonal antibody species specificity and cross-reactivity to IgG of wild felid species and other domestic animals
To determine the species specificity and cross-reactivity of the rabbit anti-Bengal tiger IgG antiserum, an indirect ELISA assay and western blot were performed as described above. For indirect ELISA, the optimal reagent concentrations were used. Briefly, a flat-bottom 96-well plate was coated in duplicate overnight at 4°C with purified orange-haired Bengal tiger IgG and IgG from each of the animals (05 µg/ml). The negative control wells consisted of no protein coating. The rabbit anti-Bengal tiger IgG polyclonal antibody at a dilution of 1:100 (20 µg/ml) was added and incubated at 37°C for 1 hr. After washing three times with PBST, the rabbit anti-Bengal tiger IgG was detected using HRP-conjugated goat anti-rabbit IgG at a 1:4000 dilution at 37°C for 1 hr. The reaction was developed by adding TMB peroxidase substrate for 30 min at room temperature under light protection conditions and subsequently stopped with 2 N H2SO4. The absorbance was read at 450 nm by using an automatic ELISA plate reader.
Results
Generation of rabbit polyclonal antibody against purified orange-haired Bengal tiger IgG
To produce rabbit polyclonal antibody against purified Bengal tiger IgG, the orange-haired Bengal tiger IgG needed to first be isolated and purified. The analysis of purified orange-haired Bengal tiger IgG using SDS-PAGE is shown in (A). The results revealed a protein band of approximately 170 kDa under non-reducing conditions as the expected size of the IgG. Additionally, protein bands of approximately 50 and 25 kDa were observed under reducing conditions corresponding to the γ heavy and κ/λ light chains of immunoglobulin, respectively. The purified IgG antibody with Montanide adjuvant was used to immunize the rabbits. The purified rabbit antisera were analyzed under non-reducing conditions, revealing a protein band of approximately 170 kDa on the Coomassie Blue-stained SDS-PAGE gel ((B)). Subsequently, the binding capacity of rabbit anti-Bengal tiger IgG antibody was evaluated using western blotting. The reactivity was observed at approximately 170 kDa () on the membrane, demonstrating the capturing ability and specificity of the antibody. The rabbit anti-Bengal tiger polyclonal antisera were subjected to further analysis.
Figure 1. Analysis of orange-haired Bengal tiger IgG. (A) The purified orange-haired Bengal tiger IgG was analyzed under reducing and non-reducing conditions using SDS-PAGE and stained with Coomassie Brilliant Blue. Lanes M: molecular mass standards; 1: Non-reducing conditions; 2: Reducing conditions. (B) The non-purified and purified rabbit anti-Bengal tiger IgG polyclonal antibody were analyzed using SDS-PAGE and stained with Coomassie Brilliant Blue. Lanes M: molecular mass standards; 1–2: Non-purified rabbit anti-tiger IgG; 3–4: Purified rabbit anti-tiger IgG.
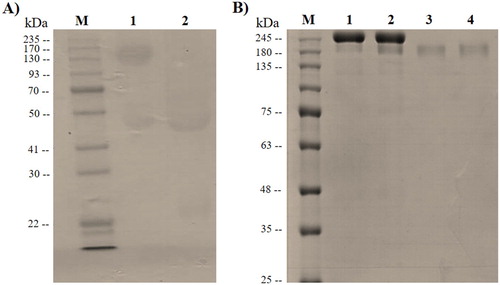
Optimization of indirect ELISA for development of alternative diagnostic tool
To the authors’ knowledge, there are no available specific serology assays for detection of the tiger IgG antibody. Therefore, an indirect ELISA was conducted with serial dilutions of the rabbit anti-Bengal tiger IgG serum against orange-haired Bengal tiger IgG-coated immunoplate. The optimal dilution volumes of the ELISA reagents were determined and were chosen using the OD values as summarized in supplemental Table S1. Considering the dilution of the plate-coating antigens, the OD values did not have significant differences with regard to OD profiles between dilutions ((A-C)). Consequently, the lowest dilution of 05 µg/ml was chosen for the coating antigen. The rabbit anti-Bengal tiger IgG showed saturated binding to Bengal tiger serum IgG at dilutions of 1:10, 1:100, and 1:1000. As a result, dilutions of 1:10 and 1:1000 showed a reduction in the OD values detected by the 1:6000 dilution of HRP-conjugated goat anti-rabbit IgG. Accordingly, this study selected the dilution of 1:100 for rabbit anti-Bengal tiger IgG and the dilution of 1:4000 for HRP-conjugated goat anti-rabbit IgG ((A)).
Figure 3. Determinations of optimal dilution of indirect ELISA reagents as presented by mean OD values at different coating antigen concentration of 0.5 µg/ml (A) 1 µg/ml (B) 2 µg/ml (C).
Note: aOrange-haired Bengal tiger IgG. bHRP-conjugated goat anti-rabbit IgG. cRabbit anti-Bengal tiger IgG polyclonal antibody generated in this study. ‘*’ indicates the suitable OD value from this study.
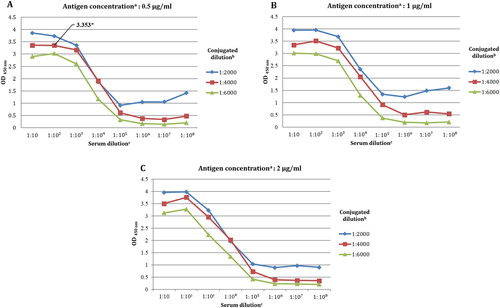
Species specificity and cross-reactivity of purified rabbit anti-Bengal tiger IgG
For the wild felid species, the specificity of rabbit anti-Bengal tiger IgG was examined using an indirect ELISA. The optimal dilution quantities of the ELISA regents were applied in this analysis. The OD values are shown in . The rabbit anti-Bengal tiger IgG showed strong reactivity with the P. tigris subspecies IgG. Furthermore, the rabbit anti-Bengal tiger IgG also cross-reacted strongly with other wild felid species.
Table 1. Species specificity of rabbit anti-Bengal tiger immunoglobulin G and cross-reactivity among wild felid species (family Felidae).
In addition, cross-reactivity of rabbit anti-Bengal tiger IgG was observed among domestic species, as shown in . Relative to the homologous reactivity of rabbit anti-Bengal tiger IgG with Bengal tiger IgG, the highest percentage cross-reactivity was with the domestic cat (63.58%), followed by the pig (38.45%). In contrast, low cross-reactivity of rabbit anti-Bengal tiger IgG was observed with cow, goat, sheep, and chicken. To confirm the cross-reactivity, western blotting was performed (). As expected, the results showed that rabbit anti-Bengal tiger IgG cross-reacted strongly with domestic cat. In addition, slight cross-reactivity was observed with the pig, while no cross-reactivity was detected with cow, goat, sheep or chicken.
Figure 4. The immunoblotting analysis of rabbit anti-Bengal tiger IgG polyclonal antibody cross-reactivity with domestic animals. The cross-reactivity was strong with the cat IgG and faint with the pig IgG.
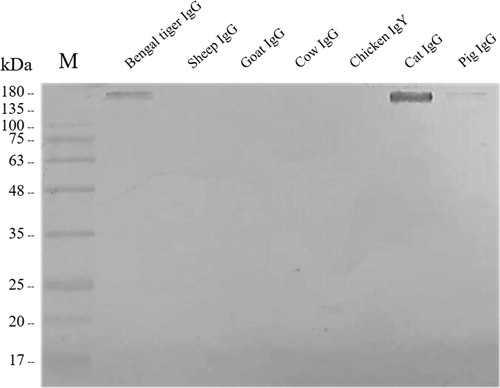
Table 2. Cross-reactivity of purified rabbit anti-Bengal tiger immunoglobulin G with domestic animals’ immunoglobulin G.
Discussion
Due to the combination of novel diseases emerging in the tiger coupled with the lack of serological assays to detect either seroconversion from pathogen exposure or vaccination, this study aimed to develop new reagents to detect and characterize tiger immune responses. To the best of the researchers’ knowledge, this study presents the first attempt at development of rabbit anti-tiger IgG polyclonal antibody for further immunological research or laboratory investigation. Not only the anti-tiger IgG can be applied to evaluate the antibody titre against vaccines in tigers and related wild felid species including FPV, FCV, feline herpes virus (FHV), and feline leukemia virus (FeLV) but also to detect certain disease such as feline infectious peritonitis (FIP), feline immunodeficiency virus (FIV) infection (Risi et al. Citation2012; Lamberski Citation2015).
IgG is the predominant immunoglobulin in humoral immunity and has the longest serum half-life of all immunoglobulin isotypes (Leusen and Nimmerjahn Citation2013). There may be a relationship between IgG level and severity of diseases or immune responses to vaccines (Kumagai et al. Citation2001; Isa et al. Citation2002; Oliveira et al. Citation2009; de la Torre et al. Citation2016; Mbengue et al. Citation2016). Therefore, IgG plays a critical role in the protection against diseases, and it can be of value in revealing immunological responses (Kinyanjui et al. Citation2004; Stanisic et al. Citation2009). Presently, little is known concerning the Bengal tiger's immune responses. Generally, an intact IgG shows variation in the molecular mass, depending upon the species, with an average of approximately 150 kDa (Janeway et al. Citation2001). Consistent with previous reports, the molecular weight of Bengal tiger IgG in the present study was approximately 170 kDa (Mandal et al. Citation2013). These results indicated that purification of orange-haired Bengal tiger IgG using the Melon™ Gel IgG Purification Kit was successful in the present study.
Cross-reactivity of anti-immunoglobulin with immunoglobulins of different species has been reported previously (Neoh et al. Citation1973; Jefferis et al. Citation1982; Lastras et al. Citation2000; Nollens et al. Citation2008). In the current study, the cross-reactivity to IgG of wild felid species was determined by indirect ELISA. The observed cross-reactivity could be explained by the fact that the rabbit anti-Bengal tiger IgG produced in this study is a polyclonal antibody that displays diverse antibody specificity against many antigenic epitopes. Thus, the cross-reactivity often occurs more readily than in the case of monoclonal antibodies (Frank Citation2002). However, there are other factors involved. Evolutionary history could be one explanation for the cross-reactivity. Consequently, it is not surprising that the anti-Bengal tiger IgG produced from the orange-haired Bengal tiger IgG in this study showed a relatively strong reaction to the homologue IgG derived from other pantherine subspecies (white, golden tabby, and snow-white tigers). Furthermore, the cross-reactivity with the Panthera lineage (lion, jaguar, leopard, panther, and clouded leopard) IgG may be due to the evolutionary relationship within the monophyly of the Panthera genus (Johnson et al. Citation1996, Citation2006; Yu and Zhang Citation2005; Christiansen Citation2008; Davis et al. Citation2010). Interestingly, the anti-Bengal tiger polyclonal antibody was able to strongly cross-react with purified sera IgG from other wild felid species beyond the Panthera lineage. This implies that the polyclonal antibody has the ability to detect wild felid species’ IgG. Remarkably, these results reflect the phylogenetic relationship within the family Felidae. Fossil records reveal that the common ancestor of the modern felid species came into existence approximately 11 million years ago in the late Miocene. With the Panthera lineage began the first divergence, followed by further diversification into seven lineages (Johnson et al. Citation2006). In support of the evolutionary relationships, the family Felidae shows high levels of genomic similarity, with very low levels of genetic diversity (Kim et al Citation2016). Therefore, it is probable that immunoglobulins are related to each other because they originated from a common ancestral precursor gene (Hill et al. Citation1966), allowing related felid species’ IgG to be definitively assigned as a homologue of Bengal tiger IgG.
Western blotting was used to confirm the observed reactive bands with domestic cat and pig serum IgG. In comparative phylogenetic relationships, the domestic cat is obviously more closely related to the tiger than other domestic species (Graphodatsky et al. Citation2011). In addition, a previous comparison of genomic sequences revealed 95.6% similarity between the tiger and the domestic cat (Cho et al. Citation2013). Therefore, it is not surprising that cross-reactivity was observed with domestic cat IgG. A close phylogenetic relationship is one possible explanation for the occurrence of the cross-reactivity. The results from this study indicate that the rabbit anti-Bengal tiger IgG polyclonal antibody generated in this study was highly specific to the Felidae species.
Although the domestic pig is an outgroup to the Felidae, low-level cross-reactivity was observed on western blotting, indicating that the rabbit anti-Bengal tiger polyclonal IgG antibody was also somewhat specific to the Suidae species. Previously, the cytochrome b gene was used for identifying and investigating the evolutionary relationships among endangered species (Irwin et al Citation1991; Janczewski et al Citation1995; Masuda et al Citation1996). The construction of a phylogenetic tree based on cytochrome b revealed that the pig has a relatively closer relationship to felid species than to other Cetartiodactyla species such as cows, sheep, and goats, based on lower genetic distance (Hsieh et al. Citation2001). Therefore, it is possible that this evolutionary relationship may explain the immunological cross-reactivity. Unfortunately, the whole-genome reference sequence is limited for the tiger in regard to investigating the evolutionary relationships or carrying out genetic analyses (Cho et al Citation2013). Nevertheless, it is an interesting observation that the IgG from the cat and the pig exhibited binding to the HCMV FcR, in contrast to the IgG from sheep, goats, cows, and chickens (Antonsson and Johansson Citation2001). It is possible that the antigenic determinants of both the cat and the pig IgG Fc regions are identical by way of sharing homologies in the amino acid sequence of the antigen (Hill et al. Citation1966; Esteves and Binaghi Citation1972). These results might support for the fact that both the pig and the cat IgG could cross-react with the rabbit anti-Bengal tiger IgG in this study. However, because whole orange-haired Bengal tiger IgG was used to immunize, it is difficult to know whether the cross-reactivity occurred with the antigenic epitopes on the heavy chains or those on the light chains. To gain a more comprehensive understanding of these phenomena, further studies on the antigenic determinants of the immunoglobulin responsible for cross-reactivity will be needed.
Conclusions
In conclusion, the rabbit anti-Bengal tiger IgG polyclonal antibody was successfully produced, and the antibody was highly specific to the P. tigris tigris IgG. Interestingly, the antibody could strongly cross-react with other animals’ IgG, particular those of the family Felidae. The importance of the characteristics of cross-reactivity for this polyclonal antibody might lie in the fact that it has the potential to be a useful research tool for further application in qualitative and quantitative analyses of IgG in felid species.
TAAR_1629937_Supplemental_Table
Download MS Word (19.2 KB)Acknowledgments
The authors would like to express their appreciation to Tiger Kingdom, Chiang Mai Night Safari, and Chiang Mai Zoo for the animal sera support. The Department of Medical Technology, Faculty of Associated Medical Sciences, Chiang Mai University, is also acknowledged for the laboratory facilities. The authors extend their gratitude to Pallop Tankaew and Melanee Dokden for their assistance in sample processing in laboratory.
Disclosure statement
No potential conflict of interest was reported by the authors.
Additional information
Funding
References
- Antonsson A , Johansson PH. 2001. Binding of human and animal immunoglobulins to the IgG Fc receptor induced by human cytomegalovirus. J Gen Virol. 82:1137–1145. doi: 10.1099/0022-1317-82-5-1137
- Appel MJ , Yates RA , Foley GL , Bernstein JJ , Santinelli S , Spelman LH , Miller LD , Arp LH , Anderson M , Barr M , et al. 1994. Canine distemper epizootic in lions, tigers, and leopards in North America. J Vet Diagn Invest. 6:277–288. doi: 10.1177/104063879400600301
- Bittle JL. 1993. Use of vaccines in exotic animals. J Zoo Wildl Med. 41:352–356.
- Cho YS , Hu L , Hou H , Lee H , Xu J , Kwon S , Oh S , Kim HM , Jho S , Kim S , et al. 2013. The tiger genome and comparative analysis with lion and snow leopard genomes. Nat Commun. 4:3433.
- Christiansen P. 2008. Phylogeny of the great cats (Felidae: Pantherinae), and the influence of fossil taxa and missing characters. Cladistics. 24:977–992. doi: 10.1111/j.1096-0031.2008.00226.x
- Cross ML , Buddle BM , Aldwell FE. 2007. The potential of oral vaccines for disease control in wildlife species. Vet J. 174:472–480. doi: 10.1016/j.tvjl.2006.10.005
- Crowther JR. 2001. The ELISA guidebook. Methods Mol Biol. 149:1–413.
- Daszak P , Cunningham AA , Hyatt AD. 2000. Emerging infectious diseases of wildlife–threats to biodiversity and human health. Science. 287:443–449. doi: 10.1126/science.287.5452.443
- Davis BW , Li G , Murphy WJ. 2010. Supermatrix and species tree methods resolve phylogenetic relationships within the big cats, Panthera (Carnivora: Felidae). Mol Phylogenet Evol. 56:64–76. doi: 10.1016/j.ympev.2010.01.036
- de La Rocque S , Rioux JA , Slingenbergh J. 2008. Climate change: effects on animal disease systems and implications for surveillance and control. Rev Sci Tech. 27:339–354. doi: 10.20506/rst.27.2.1807
- de la Torre MC , Torán P , Serra-Prat M , Palomera E , Güell E , Vendrell E , Yébenes JC , Torres A , Almirall J. 2016. Serum levels of immunoglobulins and severity of community- acquired pneumonia. BMJ Open Respir Res. 3:e000152. doi: 10.1136/bmjresp-2016-000152
- Dinerstein E , Loucks C , Wikramanayake E , Ginsberg J , Sanderson E , Seidensticker J , Forrest J , Bryja G , Heydlauff A , Klenzendorf S , et al. 2007. The fate of wild tigers. BioScience. 57:508–514. doi: 10.1641/B570608
- Dissanayake DRA , Silva ID , Gamage S , Sonnadara D , Bandara MRBN , Alokabandara SS , Jayapani VP , Jayaweera W. 2017. Feline panleukopenia virus infection in a captive- bred Bengal tiger (Panther tigris tigris) and a leopard (Panthera pradus). S L Vet J. 63:23–26. doi: 10.4038/slvj.v63i2.12
- Duarte MD , Barros SC , Henriques M , Fernandes TL , Bernardino R , Monteiro M , Fevereiro M. 2009. Fatal infection with feline panleukopenia virus in two captive wild carnivores (Panthera tigris and Panthera leo). J Zoo Wildl Med. 40:354–359. doi: 10.1638/2008-0015.1
- Esteves MB , Binaghi RA. 1972. Antigenic similarities among mammalian immunoglobulins. Immunology. 23:137.
- Frank SA. 2002. Immunology and evolution of infectious disease. New Jersey (NJ ): Princeton University Press; 348 p.
- Gallana M , Ryser-Degiorgis MP , Wahli T , Segner H. 2013. Climate change and infectious diseases of wildlife: altered interactions between pathogens, vectors and hosts. Curr Zool. 59:427–437. doi: 10.1093/czoolo/59.3.427
- Goodrich J , Lynam A , Miquelle D , Wibisono H , Kawanishi K , Pattanavibool A , Htun S , Tempa T , Karki J , Jhala Y , Karanth U. 2015. Panthera tigris. The IUCN Red List of Threatened Species 2015:e.T15955A50659951. Available from http://dx.doi.org/10.2305/IUCN.UK.2015-2.RLTS.T15955A50659951.en .
- Graphodatsky AS , Trifonov VA , Stanyon R. 2011. The genome diversity and karyotype evolution of mammals. Mol Cytogenet. 4:22. doi: 10.1186/1755-8166-4-22
- Harvell D , Altizer S , Cattadori IM , Harrington L , Weil E. 2009. Climate change and wildlife diseases: when does the host matter the most? Ecology. 90:912–920. doi: 10.1890/08-0616.1
- Hill RL , Delaney R , Fellows RE , Lebovitz HE. 1966. The evolutionary origins of the immunoglobulins. Proc Nat Acad Sci. 56:1762–1769. doi: 10.1073/pnas.56.6.1762
- Hsieh HM , Chiang HL , Tsai LC , Lai SY , Huang NE , Linacre A , Lee JCI. 2001. Cytochrome b gene for species identification of the conservation animals. Forensic Sci Int. 122:7–18. doi: 10.1016/S0379-0738(01)00403-0
- Hu T , Zhao H , Zhang Y , Zhang W , Kong Q , Zhang Z , Cui Q , Qiu W , Deng B , Fan Q , Zhang F. 2016. Fatal influenza A (H5N1) virus infection in zoo-housed tigers in Yunnan Province, China. Sci Rep. 6:25845. doi: 10.1038/srep25845
- Humphreys AF , Tan J , Peng R , Benton SM , Qin X , Worley KC , Mikulski RL , Chow DC , Palzkill TG , Ling PD. 2015. Generation and characterization of antibodies against Asian elephant (Elephas maximus) IgG, IgM, and IgA. PloS One. 10:e0116318. doi: 10.1371/journal.pone.0116318
- Irwin DM , Kocher TD , Wilson AC. 1991. Evolution of the cytochrome b gene of mammals. J Mol Evol. 32:128–144. doi: 10.1007/BF02515385
- Isa MB , Martínez L , Giordano M , Passeggi C , de Wolff MC , Nates S. 2002. Comparison of immunoglobulin G subclass profiles induced by measles virus in vaccinated and naturally infected individuals. Clin Diagn Lab Immunol. 9:693–697.
- Jakob W , Wesemeier HH. 1996. A fatal infection in a Bengal tiger resembling cytauxzoonosis in domestic cats. J Comp Pathol. 114:439–444. doi: 10.1016/S0021-9975(96)80018-1
- Janczewski DN , Modi WS , Stephens JC , O'Brien SJ. 1995. Molecular evolution of mitochondrial 12S RNA and cytochrome b sequences in the pantherine lineage of Felidae. Mol Biol Evol. 12:690–707.
- Janeway C Jr , Travers P , Walport M , Shlomchik MJ. 2001. Immunobiology: the immune system in health and disease. 5th ed. New York : Garland Publishing; 732 p.
- Jefferis R , Lowe J , Ling NR , Porter P , Senior S. 1982. Immunogenic and antigenic epitopes of immunoglobulins I. Cross-reactivity of murine monoclonal antibodies to human IgG with the immunoglobulins of certain animal species. Immunology. 45:71.
- Johnson WE , Dratch PA , Martenson JS , O'Brien SJ. 1996. Resolution of recent radiations within three evolutionary lineages of Felidae using mitochondrial restriction fragment length polymorphism variation. J Mamm Evol. 3:97–120. doi: 10.1007/BF01454358
- Johnson WE , Eizirik E , Pecon-Slattery J , Murphy WJ , Antunes A , Teeling E , O'brien SJ. 2006. The late Miocene radiation of modern Felidae: a genetic assessment. Science. 311:73–77. doi: 10.1126/science.1122277
- Kania SA , Richman L , Kennedy MA , Montali RJ , Potgieter LND. 1997. The isolation, detection, and cross-reactivity in Asian elephant IgG for the development of serological diagnostic tests. J Vet Allergy Clin Immunol. 5:125–128.
- Keawcharoen J , Oraveerakul K , Kuiken T , Fouchier RA , Amonsin A , Payungporn S , Noppornpanth S , Wattanodorn S , Theamboonlers A , Tantilertcharoen R , et al. 2004. Avian influenza H5N1 in tigers and leopards. Emerg Infect Dis. 10:2189–2191. doi: 10.3201/eid1012.040759
- Kim S , Cho YS , Kim HM , Chung O , Kim H , Jho S , Seomun H , Kim J , Bang WY , Kim C , et al. 2016. Comparison of carnivore, omnivore, and herbivore mammalian genomes with a new leopard assembly. Genome Biol. 17:211. doi: 10.1186/s13059-016-1071-4
- Kinyanjui SM , Mwangi T , Bull PC , Newbold CI , Marsh K. 2004. Protection against clinical malaria by heterologous immunoglobulin G antibodies against malaria-infected erythrocyte variant surface antigens requires interaction with asymptomatic infections. J Infect Dis. 190:1527–1533. doi: 10.1086/424675
- Kumagai T , Yan J , Graham DY , Tozuka M , Okimura Y , Ikeno T , Sugiyama A , Katsuyama T , Ota H. 2001. Serum immunoglobulin G immune response to Helicobacter pylori antigens in Mongolian gerbils. J Clin Microbial. 39:1283–1288. doi: 10.1128/JCM.39.4.1283-1288.2001
- Laemmli UK. 1970. Cleavage of structural proteins during the assembly of the head of bacteriophage T4. Nature. 227:680–685. doi: 10.1038/227680a0
- Lamberski N. 2015. Felidae. In: Miller RE , Fowler ME , editors. Fowler’s zoo and wild animal medicine, volume 8. St.Louis (MO ): Elsevier Saunders; p. 467–476.
- Lastras ME , Pastor J , Viñ L , Marco I , Lavin S. 2000. Immunoglobulin G class identification from wild ungulates by cross-reactivity with antisera to domestic animals. J Vet Med B Infect Dis Vet Public Health. 47:429–432. doi: 10.1046/j.1439-0450.2000.00365.x
- Leusen JH , Nimmerjahn F. 2013. The role of IgG in immune responses. In: N. Falk , editor. Molecular and cellular mechanisms of antibody activity. New York (NY ): Springer; p. 85–112.
- Lewis KM , Cohn LA , Downey ME , Whitney MS , Birkenheuer AJ. 2012. Evaluation of Cytauxzoon felis infection status in captive-born wild felids housed in an area endemic for the pathogen. J Am Vet Med Assoc. 241:1088–1092. doi: 10.2460/javma.241.8.1088
- Lindahl JF , Grace D. 2015. The consequences of human actions on risks for infectious diseases: a review. Infect. Ecol Epidemiol. 5:30048. doi: 10.3402/iee.v5.30048
- Luo SJ , Kim JH , Johnson WE , Van Der Walt J , Martenson J , Yuhki N , Miquelle DG , Uphyrkina O , Goodrich JM , Quigley HB , Tilson R. 2004. Phylogeography and genetic ancestry of tigers (Panthera tigris). PLoS Biol. 2:e442. doi: 10.1371/journal.pbio.0020442
- Mandal E , Batabyal S , Chattopadhyay S , Kesh SS , Barui A. 2013. Partial purification and immune-biochemical characterization of Royal Bengal tiger (Panthera tigris tigris) serum immunoglobulin G. Explor Anim Med Res. 3:131–135.
- Masuda R , Lopez JV , Slattery JP , Yuhki N , O'Brien SJ. 1996. Molecular phylogeny of mitochondrial cytochrome b and 12S rRNA sequences in the Felidae: ocelot and domestic cat lineages. Mol Phylogenet Evol. 6:351–365. doi: 10.1006/mpev.1996.0085
- Mbengue B , Fall MM , Sylla Niang M , Niang B , Varela ML , Diatta AM , Mbow M , Ndiaye K , Ndiaye Diallo R , Dieye A , Perraut R. 2016. Relationship between antibody levels, IgG binding to Plasmodium falciparum-infected erythrocytes, and disease outcome in hospitalized urban malaria patients from Dakar, Sénégal. BioMed Res Int. 2016:1–11. doi: 10.1155/2016/5381956
- McCallum H , Dobson A. 1995. Detecting disease and parasite threats to endangered species and ecosystems. Trends Ecol Evol. 10:190–194. doi: 10.1016/S0169-5347(00)89050-3
- Nagao Y , Nishio Y , Shiomoda H , Tamaru S , Shimojima M , Goto M , Une Y , Sato A , Ikebe Y , Maeda K. 2012. An outbreak of canine distemper virus in tigers (Panthera tigris): possible transmission from wild animals to zoo animals. J Vet Med Sci. 74:699–705. doi: 10.1292/jvms.11-0509
- Neoh SH , Jahoda DM , Rowe DS , Voller A. 1973. Immunoglobulin classes in mammalian species identified by cross-reactivity with antisera to human immunoglobulin. Immunochemistry. 10:805–813. doi: 10.1016/0019-2791(73)90184-5
- Nollens HH , Ruiz C , Walsh MT , Gulland FM , Bossart G , Jensen ED , McBain JF , Wellehan JF. 2008. Cross-reactivity between immunoglobulin G antibodies of whales and dolphins correlates with evolutionary distance. Clin Vaccine Immunol. 15:1547–1554. doi: 10.1128/CVI.00219-08
- Oliveira TM , Mineo TW , Bason M , Day MJ , Machado RZ. 2009. Igg subclass profile of serum antibodies to Leishmania chagasi in naturally infected and vaccinated dogs. Vet Parasitol. 162:16–22. doi: 10.1016/j.vetpar.2009.02.018
- Plumb G , Babiuk L , Mazet J , Olsen S , Pastoret P , Rupprecht C , Slate D. 2007. Vaccination in conservation medicine. Rev Sci Tech. 26:229–241. doi: 10.20506/rst.26.1.1740
- Risi E , Agoulon A , Allaire F , Le Dréan-Quénec'hdu S , Martin V , Mahl P. 2012. Antibody response to vaccines for rhinotracheitis, caliciviral disease, panleukopenia, feline leukemia, and rabies in tigers (Panthera tigris) and lions (Panthera leo). J Zoo Wildl Med. 43:248–255. doi: 10.1638/2010-0166.1
- Sadler RA , Ramsay E , McAloose D , Rush R , Wilkes RP. 2016. Evaluation of two canine distemper virus vaccines in captive tigers (Panthera tigris). J Zoo Wildl Med. 47:558–563. doi: 10.1638/2015-0223.1
- Sanderson EW , Forrest J , Loucks C , Ginsberg J , Dinerstein E , Seidensticker J , Leimgruber P , Songer M , Heydlauff A , O’Brien T , et al. 2010. Setting priorities for tiger conservation: 2005–2015. In: Tilson R , Nyhus PJ , editors. Tigers of the world: the science, politics and conservation of Panthera tigris (Noyes series in animal behavior, ecology, conservation, and management). 2nd ed. London : Academic Press; p. 143–161.
- Stanisic DI , Richards JS , McCallum FJ , Michon P , King CL , Schoepflin S , Gilson PR , Murphy VJ , Anders RF , Mueller I , Beeson JG. 2009. Immunoglobulin G subclass- specific responses against Plasmodium falciparum merozoite antigens are associated with control of parasitemia and protection from symptomatic illness. Infect Immun. 77:1165–1174. doi: 10.1128/IAI.01129-08
- Tian J , Liu D , Liu Y , Wu H , Jiang Y , Zu S , Liu C , Sun X , Liu J , Qu L. 2016. Molecular characterization of a feline calicivirus isolated from tiger and its pathogenesis in cats. Vet Microbial. 192:110–117. doi: 10.1016/j.vetmic.2016.07.005
- Towbin H , Staehelin T , Gordon J. 1979. Electrophoretic transfer of proteins from polyacrylamide gels to nitrocellulose sheets: procedure and some applications. Proc Natl Acad Sci. 76:4350–4354. doi: 10.1073/pnas.76.9.4350
- Vongchan P. 2013. Production of a rabbit polyclonal anti-elephant IgG antibody for the elephant laboratory investigation. Chiang Mai J Sci. 40:376–385.
- Wang X , Li T , Liu H , Du J , Zhou F , Dong Y , He X , Li Y , Wang C. 2017. Recombinant feline parvovirus infection of immunized tigers in central China. Emerg Microbes Infect. 6:e42.
- Yang YR , Feng YJ , Lu YY , Dong H , Li TY , Jiang YB , Zhu XQ , Zhang LX. 2017. Antibody detection, isolation, genotyping, and virulence of Toxoplasma gondii in captive felids from China. Front Microbial. 8:1414. doi: 10.3389/fmicb.2017.01414
- Yu L , Zhang YP. 2005. Phylogenetic studies of pantherine cats (Felidae) based on multiple genes, with novel application of nuclear β-fibrinogen intron 7 to carnivores. Mol Phylogenet Evol. 35:483–495. doi: 10.1016/j.ympev.2005.01.017