ABSTRACT
The objective of the research was to quantify three antibiotic resistance genes (tetQ, cfxA and mefA) in the faeces of dairy cows following therapeutic and prophylactic antibiotic treatments. Manure collected from dairy cows treated with either no antibiotic, pirlimycin hydrochloride (PIRL), ceftiofur crystalline free acid (CCFA) or cephapirin benzathine (CEPH) were submitted to quantitative PCR analysis. No treatment effects on the abundance of the tetQ and cfxA were observed. There was a trend for the abundance of the mefA to be increased in cows treated with PIRL (P = 0.07). Overall, the results showed no difference of measured three ARGs from cows receiving different antibiotics. Considering the limited scope of our investigation, further investigation is needed to provide more information on ARGs excretion from cows that received therapeutic and prophylactic antibiotic treatment.
Antibiotics, commonly used for therapeutic and prophylactic purposes in livestock, are playing an important role in disease treatment and disease prevention. However, its use has caused a significant increase in antibiotic resistance genes (ARGs) and resistant bacteria selection in the environment (Fahrenfeld et al. Citation2014). Runoff from farms and land application of manure have been reported to carry over ARGs into the environment and agricultural produce (Marti et al. Citation2013). Rapid and widespread emergence of both animal and human pathogens resistant to multiple antibiotics, also known as ‘superbugs’, has caused great concerns of public (Aslam et al. Citation2018). An example would be methicillin-resistant Staphylococcus aureus (MRSA) that is resistant to methicillin, amoxicillin, penicillin, oxacillin and other common antibiotics known as cephalosporins. In Germany, it was found that at least 10% of sporadic infections of healthcare-associated (HA)-MRSA and community-associated (CA)-MRSA is due to livestock-associated MRSA (LA)-MRSA, which is initially associated with livestock (Cuny et al. Citation2015).
In the dairy industry, common antibiotic uses involve the treatment or prevention of bacterial infections in dairy calves as well as the prevention (dry cow therapy) or treatment of mastitis in dairy cows. When cattle are therapeutically and prophylactically treated with antibiotics, impacts on their intestinal bacteria are likely, such as increased resistance to the antibiotics used (Mirzaagha et al. Citation2011).
At present, resistance to tetracycline has spread to almost all bacterial genera due to its previous overuse in human and veterinary medicine, such as growth promoters in the animal industry (Aminov et al. Citation2001). It was reported tetracycline accounted for 64% of the total amount of antibiotics sales and distribution of medically important antimicrobials approved for use in food-producing animals in 2017 (FDA Citation2018). One of the tetracycline resistance genes is tetQ, which encodes a protein that modifies the ribosome, thus abolishing the inhibitory effects of tetracycline on protein synthesis (Chopra and Roberts Citation2001). The β-lactam antibiotics are a broad class of antibiotics, consisting of all antibiotic agents that contain a β-lactam ring in their molecular structures. The β-lactam resistance genes, such as cfxA, encode β-lactamase, which provides antibiotic resistance by breaking the antibiotic’s ring structure (Munita and Arias Citation2016). The lincosamide, macrolide and streptogramin share the 50S subunit binding site, although they all possess a different structure. Since they function similarly, these antimicrobials are often linked together and called the Macrolide-Lincosamide-Streptogramin (MLS) group (Roberts Citation2008). The mefA, an example of MLS resistance genes, encodes the efflux pump, which can pump the antibiotics out of the bacterial cell, thus rendering it ineffective (Daly et al. Citation2004). Knowledge of the identity and distribution patterns of these resistance genes remains limited. The objective of this research was to use qPCR techniques to determine the effect of pirlimycin hydrochloride (mastitis treatment), ceftiofur crystalline free acid (metritis treatment) and cephapirin benzathine (dry cow therapy) on the temporal pattern of excretion of antibiotic resistance genes (tetQ, cfxA and mefA) to identify key time points for producers to focus their manure management strategies, helping to prevent the spread of antibiotic resistance.
Figure 1. Gene copies (log10) of tetQ, cfxA and mefA per gram of faeces from CCFA (ceftiofur crystalline free acid sterile suspension), PIRL (pirlimycin hydrochloride) and CEPH (cephapirin benzathine) treatment.
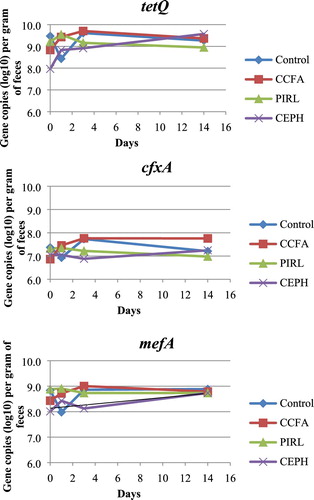
The experiment was conducted under the review and approval of the Animal Care and Use Committee (protocol 12-184-DASC) at Virginia Polytechnic Institute and State University.
Twelve Holstein cows in their first lactation (days in milk 110–200) from the Virginia Tech Dairy Center (Blacksburg, VA) were assigned to one of the four antibiotic treatments. Farm records showed that these cows had not received any antibiotic treatment for at least 9 months prior to parturition. During the study, cows were housed individually in tie-stalls with rubber mats and were fed a total mixed ration twice daily with ad libitum access to water. Except for the three dry cows that were assigned cephapirin benzathine for dry cow therapy, all of the other nine cows were randomly assigned to the rest of the three treatments. Cows for cephapirin benzathine treatment were selected based on the previously mentioned criteria as well as a scheduled date for dry-off that overlapped with the time of the experiment. Cows (n = 3) receiving the cephapirin benzathine (CEPH) treatment were dried-off on day 0. Before treated, the cows were milked, and each teat was cleaned with a gauze pad soaked in 70% isopropyl alcohol. Each teat was injected with 10 mL cephapirin benzathine (300 mg cephapirin, (ToMorrow®, Boehringer Ingelheim Vetmedica, Inc., St. Joseph, MO),) and then sealed with Orbeseal® (bismuth subnitrate, colloidal silicon dioxide and liquid paraffin; Zoetis, Madison, NJ). Cows (n = 3) receiving the pirlimycin hydrochloride (PIRL) treatment were infused with 10 mL of pirlimycin hydrochloride (50 mg pirlimycin, Pirsue®, Zoetis, Madison, NJ) in their front left-quarter on day 0 and day 1. Again, similar to for the cows receiving dry-off therapy, the teat was cleaned before treatment. Cows (n = 3) receiving the ceftiofur crystalline free acid (CCFA) treatment were injected subcutaneously with 1.5 mL ceftiofur crystalline free acid sterile suspension (150 mg ceftiofur, Excede®, Zoetis, Madison, NJ) per 45.4 kg live weight at the base of the right ear on day 0 and again at the base of the left ear on day 3. The control group cows (n = 3) received no antibiotic. Faecal samples were collected from all cows 30 min prior to antibiotic administration on day 0 and once daily on day 1, 3 and 14 following treatment. Faecal samples were collected rectally using a clean palpation sleeve and sterile lubricant for each collection and were immediately stored in a −20°C freezer until analysis. DNA from samples was extracted using the QIAamp® Fast DNA Stool Mini Kit (QIAGEN, Germantown, MD) and analysed with qPCR for 16S rRNA genes: tetQ, cfxA and mefA (). Statistical analysis was conducted using PROC GLIMMIX in SAS 9.2 (SAS Institute Inc., Cary, NC) with treatment, day and treatment by day interaction as fixed effects in the model. Data were logarithmically transformed to achieve normality before statistical analysis. Samples from d 0 were used as a covariate. Significance was declared at P < 0.05. Trends were declared at P < 0.10.
Table 1. Primers for the quantitative PCR.
The PIRL-treated groups had a numerically lower abundance of 16S rRNA gene but not statistically different. The tetQ and cfxA gene abundance was not affected by treatment, day and treatment by day interaction (). There was a trend that the PIRL-treated cows had a higher absolute abundance of the mefA gene (P = 0.07).
Table 2. Effect of antibiotic treatment on the absolute abundance of antibiotic resistance genes in dairy cow faeces.
Quantifying 16S rRNA gene, which exists in all bacteria cells, is one of the most popularly used techniques for quantifying bacteria community population in environmental samples (Suzuki et al. Citation2000). Hornish et al. (Citation1992) reported that approximately 24% of the intra-mammary administered prilimycin was excreted through faeces. In the current study, the numerically lower bacteria counts (16S rRNA gene) of PIRL-treated cows could be due to a large percentage of pirlimycin entering the gut thus reducing bacteria population. In contrast, cephapirin does not easily cross from the udder into the blood (Gehring and Smith Citation2006); thus, the bacterial population in CEPH-treated cows was close to the control cows.
In most dairies, treatment for mastitis, metritis and dry cow therapy are mostly performed by antibiotic use. Pirlimycin hydrochloride for mastitis treatment belongs to the lincosamide class of antimicrobials. There was a trend that mefA, which encodes for resistance to the Macrolide-Lincosamide-Streptogramin group, was higher than other groups. Ceftiofur crystalline free acid for metritis treatment and cephapirin benzathine for dry cow therapy all target bacteria-producing β-lactamase. The resistance gene cfxA coding for β-lactamase was not different in the CCFA and CEPH groups when compared with the control (, ). This could be partly due to the short term of the antibiotic administration. Compared with the CCFA groups, the CEPH group had a numerically lower abundance of cfxA. CCFA was administered on day 0 and day 3, while CEPH was administered only on day 0 could be the possible reason.
It has been reported that resistance genes coding resistance to fluoroquinolones, tetracyclines, β-lactams and other classes of antibiotics have been observed in cattle that were never exposed to antibiotics (Durso et al. Citation2011). Consistent with the previous results, all the three ARGs were detected in the control cows in this study. Antibiotic residue in the environment can promote the development and spread of ARGs in the environmental bacterial populations which might be the reason ARGs were detected on the animals never exposed to antibiotics. Thames et al. (Citation2012) detected resistance genes for tetracycline, sulphonamide and macrolide-lincosamide-streptogramin in newborn calves. In a study comparing the occurrence of antibiotic resistance, it was found that the frequency of antibiotic resistance in organic farms was not different from conventional farms (Roesch et al. Citation2006). However, antibiotic use did increase abundance and diversity of ARGs in swine microbiomes whereas the animals from the control group already had a high background of resistance genes (Looft et al. Citation2012). In the current study, there is no difference between the control group and other treatment group animals for all of the ARGs measured. Except the short term of the antibiotic administration, the dose could be another reason. It should be noted that for the pirlimycin hydrochloride group (mimic mastitis treatment), ceftiofur crystalline free acid group (mimic metritis treatment), the actual results from reality might differ from what was observed in the current study as the cows used here were healthy cows. The antibiotics activity can be affected by the disease status as serum proteins binding action could surrender the antimicrobial ineffective (Li et al. Citation2017). However, information of disease status on ARGs development was not found.
Overall, the results showed no difference of measured three ARGs from cows receiving different antibiotics. Considering the limited scope of our investigation, the next-generation sequencing techniques should be further used to provide more information on ARGs excretion from cows that received therapeutic and prophylactic antibiotic treatment.
Statement
This experiment was conducted under the review and approval of the Animal Care and Use Committee (protocol 12-184-DASC) at Virginia Polytechnic Institute and State University.
Disclosure statement
No potential conflict of interest was reported by the authors.
References
- Aminov RI, Garrigues-Jeanjean N, Mackie RI. 2001. Molecular ecology of tetracycline resistance: development and validation of primers for detection of tetracycline resistance genes encoding ribosomal protection proteins. Appl Environ Microbiol. 67:22–32. doi: 10.1128/AEM.67.1.22-32.2001
- Aslam B, Wang W, Arshad MI, Khurshid M, Muzammil S, Rasool MH, Nisar MA, Alvi RF, Aslam MA, Qamar MU, et al. 2018. Antibiotic resistance: a rundown of a global crisis. Infect Drug Resist. 11:1645–1658. doi: 10.2147/IDR.S173867
- Chopra I, Roberts M. 2001. Tetracycline antibiotics: mode of action, applications, molecular biology and epidemiology of bacterial resistance. Microbiol Mol Biol Rev. 65:232–260. doi: 10.1128/MMBR.65.2.232-260.2001
- Cuny C, Wieler LH, Witte W. 2015. Livestock-associated MRSA: the impact on humans. Anitibiotics (Basel). 4:521–543.
- Daly MM, Doktor S, Flamm R, Shortridge D. 2004. Characterization and prevalence of mefA, mefE, and the associated msr(D)gene in Streptococcus pneumoniae clinical isolates. J Clin Microbiol. 42:3570–3574. doi: 10.1128/JCM.42.8.3570-3574.2004
- Durso LM, Harhay GP, Bono JL, Smith TPL. 2011. Virulence-associated and antibiotic resistance genes of microbial populations in cattle feces analyzed using a metagenomic approach. J Microbiol Meth. 84:278–282. doi: 10.1016/j.mimet.2010.12.008
- Fahrenfeld N, Knowlton K, Krometis LA, Hession WC, Xia K, Lipscomb E, Libuit K, Green BL, Pruden A. 2014. Effect of manure application on abundance of antibiotic resistance genes and their attenuation rates in soil: field-scale mass balance approach. Environ Sci Technol. 48:2643–2650. doi: 10.1021/es404988k
- FDA. 2018. Summary report on antimicrobials sold or distributed for use in food-producing animals in 2017. Center for veterinary Medicine. December, 2018.
- Gehring R, Smith GW. 2006. An overview of factors affecting the disposition of intramammary preparations used to treat bovine mastitis. J Vet Pharmacol Ther. 29:237–241. doi: 10.1111/j.1365-2885.2006.00750.x
- Hornish RE, Arnold TS, Baczynskyj L, Chester ST, Cox TD, Flook TF, Janose RL, Kloosterman DA, Nappier JM, Reeves DR, et al. 1992. Pirlimycin in the dairy-cow - metabolism and residue studies. Xenobiotics and Food-Producing Animals. 503:132–147. doi: 10.1021/bk-1992-0503.ch009
- Li J, Xie S, Ahmed S, Wang F, Gu Y, Zhang C, Chai X, Cai J, Cheng G. 2017. Antimicrobial activity and resistance: influencing factors. Front Phamaol. doi:10.3389/fphar.2017.00364.
- Looft T, Johnson TA, Allen HK, Bayles DO, Alt DP, Stedtfeld RD, Sul WJ, Stedtfeld TM, Chai B, Cole JR, et al. 2012. In-feed antibiotic effects on the swine intestinal microbiome. Proc Natl Acad Sci. 109:1691–1696. doi: 10.1073/pnas.1120238109
- Marti R, Scott A, Tien YC, Murray R, Sabourin L, Zhang Y, Topp E. 2013. Impact of manure fertilization on the abundance of antibiotic-resistant bacteria and frequency of detection of antibiotic resistance genes in soil and on vegetables at harvest. Appl Environ Microbiol. 79:5701–5709. doi: 10.1128/AEM.01682-13
- Mirzaagha P, Louie M, Sharma R, Yanke LJ, Topp E, McAllister TA. 2011. Distribution and characterization of ampicillin-and tetracycline-resistant Escherichia coli from feedlot cattle fed subtherapeutic antimicrobials. BMC Microbiol. 11:78. doi: 10.1186/1471-2180-11-78
- Munita J, Arias C. 2016. Mechanisms of antibiotics resistance. Microbiol Spectr. April. 4:2. doi:10.1128/microbiolspec.VMBF-0016-2015.
- Roberts MC. 2008. Update on macrolide-lincosamide-streptogramin, ketolide, and oxazolidinone resistance genes. FEMS Microbiol Lett. 282:147–159. doi: 10.1111/j.1574-6968.2008.01145.x
- Roesch M, Perreten V, Doherr MG, Schaeren W, Schallibaum M, Blum JW. 2006. Comparison of antibiotic resistance of udder pathogens in dairy cows kept on organic and on conventional farms. J Dairy Sci. 89:989–997. doi: 10.3168/jds.S0022-0302(06)72164-6
- Sóki J, Gonzalez SM, Urbán E, Nagy E, Ayala JA. 2011. Molecular analysis of the effector mechanisms of cefoxitin resistance among bacteroides strains. J Antimicrob Chemother. 66:2492–2500. doi: 10.1093/jac/dkr339
- Suzuki MT, Taylor LT, DeLong EF. 2000. Quantitative analysis of small-subunit rrna genes in mixed microbial populations via 5'-nuclease assays. Appl Environ Microbiol. 66:4605–4614. doi: 10.1128/AEM.66.11.4605-4614.2000
- Thames CH, Pruden A, James RE, Ray PP, Knowlton KF. 2012. Excretion of antibiotic resistance genes by dairy calves fed milk replacers with varying doses of antibiotics. Front Microbiol. 3:139. doi: 10.3389/fmicb.2012.00139