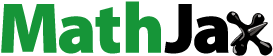
ABSTRACT
Research on the use of heart of palm by-product (HPB) as a feed for dairy cattle is needed since it is a low-cost alternative in addition avoiding environmental contamination. The objective of this study was to evaluate intake, digestibility, milk yield and composition from cows fed HPB. Sixteen Holstein cows were used. Treatments consisted of fresh sugarcane replacement (dry matter basis – DM) of HPB at levels 0%, 7.5%, 15% and 22.5%. Experimental design was randomized blocks with measures repeated in time, and data were compared by linear, quadratic and cubic contrasts. A decreasing linear effect of HPB was observed on DM, organic matter (OM), crude protein and non-fibrous carbohydrates intakes and on digestibility of DM and neutral detergent fibre. A quadratic effect was observed for metabolizable energy intake and OM digestibility. Milk yield and composition were not affected by levels of HPB added to the diet. A cubic effect was detected for fat-corrected milk (FCM) and energy-corrected milk (ECM). HPB is detrimental to intake and digestibility, but the 7.5% replacement level of HPB is recommended depending on the milk production level, since rumen environment was improved, leading to greater FCM and ECM yields at lower production costs.
Introduction
In the global production of heart of palm, Brazil, Costa Rica and Ecuador are the largest producers and exporters (Bergo et al. Citation2013). Countries like the USA (Hawaii), France (Réunion Island), Indonesia and Malaysia have also been developing heart of palm production (Steinmacher Citation2011) because of the ban on the extraction of wild palm heart and the demand in the consumer market for sustainable products.
Of the cultivated species, production of heart of palm from Alexander palm (Archontophoenix alexandrae) has increased due to the high value and production characteristics of this species (Bayão et al. Citation2017). The processing of one plant yields approximately 400 g of commercial heart of palm and generates approximately 13 kg of waste, including leaves, stipe and sheaths (Fermino et al. Citation2014), which correspond to 96.9% of waste per plant.
During the production process, leaves are left on the field, and stems are transported to the factory, where they are processed and the leaf sheaths are discarded. Leafs, with a low carbon/nitrogen ratio, tend to decompose rapidly (Silva et al. Citation2014), while the sheaths, on the other hand, because of their high carbon/nitrogen ratio, take some time to degrade in the soil, thus becoming an environmental problem.
The use of wastes derived from agrosystem areas has risen broadly (Romano et al. Citation2010; Gervasi et al. Citation2017) and it is appreciable for the consumers, who are increasingly involved in environmental causes and zero-waste movements. In the case of the waste from heart-of-palm production; however, little information can be found on its use in animal nutrition. Research results in the literature are mainly related to the peach palm species (Bactris gasipaes) in sheep feeding (Rombola et al. Citation2010) and in silage production (Rodrigues Neto et al. Citation2001; Oliveira et al. Citation2010; Schmidt et al. Citation2010). Whereas for the waste from the Alexander palm, there is only one study involving sheep feeding (Bayão et al. Citation2014) and silage production (Bayão et al. Citation2017). Thus, research related to the use of this waste as feed for dairy cattle should be encouraged, as this is a low-cost alternative that can help in reducing costs in the production system in addition to avoiding environmental contamination.
Our hypothesis was that heart-of-palm by-product (HPB) from the Alexander Palm can be used as a replacement of fresh sugarcane in diets for lactating cows without affecting intake and performance. The objective of this study was to evaluate intake, digestibility, and milk production and composition of milk-producing cows fed increasing levels of HPB.
Material and methods
This research was approved by the institutional Committee on Animal Use (record number 21/2013). All procedures have been conducted in accordance with the guidelines set out by the Brazilian College of Animal Experimentation in the Code of Practice for the Care and Use of Animal for Experimental Purposes.
Sixteen multiparous Holstein cows in the final third of their lactation with an average yield of 16.7 ± 4.5 kg of milk, average live weight 628.5 ± 10.6 kg and 286.75 ± 23.5 days in lactation were confined in a loose-housing system adapted with steel wire ropes to allow for individualization of the animals. Cows were adapted for 21 days before the beginning of the experiment. The experimental period lasted 60 days, which were subdivided into four 15-day periods, in which feed samples and orts were collected daily. Samples of blood, urine, feces and milk were collected on the last five days of each period. The HPB was processed daily in a stationary chopper adjusted for cuts between 2 and 3 cm, as was done with the sugarcane, which was cut daily in the morning ().
Table 1. Chemical composition of HPB production and fresh sugarcane.
Levels of fresh sugarcane replacement of HPB in the diet (DM basis) were 0%, 7.5%, 15%, and 22.5%. Different concentrates were used for each level to meet the requirements for 600-kg cow producing 25 kg of milk per day (), which was the average production of the animals at the beginning of the study (previous to adaptation period). Diets were formulated to be isoenergetic and isonitrogenous to meet the requirements of crude protein (CP), macrominerals and trace minerals, with a roughage:concentrate ratio of 50:50, according to NRC (Citation2001).
Table 2. Ingredients and chemical composition of experimental diets.
Feed was supplied twice daily (06h30 and 15h30) and adjusted to keep orts at a maximum 10% of the total supplied. Orts were weighed to estimate daily intake and collected along with feed supplied samples. Animals were weighed at the beginning and the end of the experiment, to determine live weight gain.
Animals were milked twice daily (06h00 and 15h00), and their respective yields were recorded. On days 1 and 15 of each experimental period, according to recommendations by Broderick and Clayton (Citation1997), milk was collected using a device coupled to the milking machine, twice daily (morning and afternoon), forming a composite sample that was conditioned in a plastic bottle with Bronopol® at 4°C. Contents of milk fat, protein, lactose and total solids were determined using a MilkoScan® FT 120 analyzer (Foss Electric, Hillerod, Denmark) via infrared spectroscopy. Fat-corrected milk (FCM) was calculated as FCM (kg/d) = 0.4 × milk (kg/d) + 15 × fat (kg/d) (NRC Citation2001), and energy-corrected milk (ECM) was calculated as ECM = milk yield, kg/d × [(0.0929 × percentage of fat) + (0.0563 × percentage of true protein) + (0.0395 × percentage of lactose)], divided by the assumed energy content of 4% FCM of 0.749 NEL, Mcal/kg (Tyrrell and Reid Citation1965), where the true protein was calculated as 95% of the milk CP (NRC Citation2001). Milk urea nitrogen was determined using Bioclin® kits (Bioclin/Quibasa, Belo Horizonte, Brazil).
Blood samples were collected from all cows on day 14 of each experimental period, four hours after morning feeding via jugular venipuncture and into tubes containing EDTA. After collection, blood samples were immediately centrifuged at 2700 × g for 20 min for the separation of the plasma and were stored at 18°C for subsequent analysis of plasma urea-N (Talke and Schubert Citation1965).
Spot urine samples (60 mL) were collected from all cows on day 13 of each experimental period by urination stimulated by vulva massage. Urine samples were filtered, and 10 mL were collected and diluted directly in 40 mL 0.036 N sulphuric acid solution to prevent bacterial destruction of the purine derivatives. Samples were stored at 4°C for further analyses of urea using commercial kits (Laborlab® and CELM®) with the enzymatic colorimetric endpoint method. The concentration of plasma urea nitrogen was obtained by multiplying the urea content by 0.466, which corresponded to the nitrogen content (N) in urea. Total daily urinary excretion was estimated using the daily creatinine excretion as proposed by Chizzotti et al. (Citation2008). Microbial protein synthesis (MPS) was estimated as a function of absorbed purines, which was calculated from the excretion of the purine derivatives, uric acid and allantoin in urine and milk, according to equations proposed by Chen and Gomes (Citation1992).
From days 8–15 of each experimental period, spot samples of feces were taken. Composite samples were formed per period for the feeds supplied, and one orts sample was formed per animal per period. Samples were partially dried at 55°C for 72 h in a forced ventilation oven and ground in a Willey mill (Thomas Scientific, Swedesboro, NJ) with a 1 mm screen and stored in plastic containers.
Feedstuffs, orts and feces were analysed for dry matter (DM) (Method 934.01; AOAC Citation1990), organic matter (OM) (method 942.05; AOAC Citation1990), CP (method 920.87; AOAC Citation1990) and ether extract (EE) (Ankom® method; AOCS Citation2004). The neutral detergent fibre (NDF) was analysed according to the technique described by Mertens (Citation2002) without the addition of sodium sulphite, but with the addition of detergent thermostable alpha amylase (Ankon Tech Corporation Fairport, NY). Ash correction was performed in the NDF residues (INCT-CA M-002/1). NDF was also corrected for protein residues according to Licitra et al. (Citation1996). Non-fibre carbohydrates (NFC) were calculated as proposed by Detmann and Valadares Filho (Citation2010). Metabolizable energy (ME) was estimated according to NRC (Citation2001) recommendations.
Apparent nutrient digestibilities were estimated using an indigestible neutral detergent insoluble fibre (iNDF) as the marker (Detmann et al. Citation2012). Briefly, samples of feedstuffs, orts and feces were incubated in the rumen of cannulated animals in F-57 bags Ankon® for 288 h, after which they were analysed for NDF (Valente et al. Citation2011a).
The experiment was designed in a randomized block design with repeated measures, using milk yield as the criterion for the formation of blocks. Data were compared by linear, quadratic and cubic contrasts of the levels of HPB.
The variables were analysed according to the following statistical model:where μ is the overall mean; Ti is the fixed effect of treatment i; δik is the random error with mean 0 and variance σ2, the variance between animals within treatments and it is equal to the covariance between repeated measures within animals; βj is the random effect of block j; Pe is the fixed effect of period e; (TxP)ie is the effect of interaction between treatment i and period e; and εijke is the random error with mean 0 and variance σ2, the variance between measures within animals.
As cows were weighed only at the beginning and end of the experiment, average daily gain was evaluated as a completely randomized block design. Data were analysed using the PROC MIXED procedure of SAS University Edition and significance was declared when P < 0.05.
Results
Interaction treatment vs. period was not detected for any of the evaluated variables evaluated in the study (P > 0.05, data not shown). Data on intake, milk yield, FCM and ECM were affected by period (P < 0.05, data not shown). This effect was expected due to the physiological effect of advancing lactation and because data were analysed as repeated measurements.
DM, OM, CP and NFC intake were linearly reduced (P < 0.05), while a quadratic effect was observed for ME intake (P < 0.05) with the addition of the HPB in the roughage (). A linear reduction was observed for digestibility coefficients of DM and NDFap. (P < 0.05) and a quadratic effect was noticed for OM digestibility (P < 0.05; ).
Table 3. Intake and digestibility of nutrients in dairy cows fed a diet with HPB in replacement of sugarcane.
A decreasing linear effect was observed for MPS and urinary nitrogen excretion (UN) (P < 0.05), while efficiency of microbial protein synthesis, urinary urea nitrogen, plasma urea nitrogen and milk urea nitrogen were not influenced by the levels of HPB on diet (P > 0.05; ).
Table 4. Microbial protein synthesis (MPS), efficiency of microbial protein synthesis (EMS), urinary nitrogen (UN), urinary urea nitrogen (UUN), plasma urea nitrogen (PUN), and milk urea nitrogen (MUN) of dairy cows fed a diet with HPB for replacement of sugarcane.
Milk yield, milk efficiency and milk composition were not affected by levels of HPB added to the diet (P > 0.05; ), but a cubic effect was detected for FCM and ECM (P < 0.05). FCM and ECM increased approximately 22% and 19%, respectively, when 7.5% of HPB was included in diet compared to 0%. A quadratic effect was observed for ECM efficiency (P < 0.05) and a cubic effect for FCM efficiency and cows’ ADG (P < 0.05; ).
Table 5. Milk yield, FCM, ECM, milk composition, feed efficiency, and average daily gain of dairy cows fed a diet with HPB in replacement of sugarcane.
Discussion
A huge amount of waste is produced as a consequence of the development of heart of palm production from the Alexander Palm. This waste is harmful for the environment and its usage to feed ruminants could preserve the ambience, in addition to having low acquisition cost for ruminant producers. Therefore, in vivo evaluations on animals’ performance when fed the waste, in this case the HPB, are crucial for its use in ruminant nutrition.
In this research, dry matter intake decreased as the amount of HPB included in the diet was increased. The NDF content of the HPB differed from the NDF content of fresh sugarcane by 4.04 percentage points (). This corresponded to 5.86%, indicating that they were very similar with regard to the proportion of fibrous fraction. However, comparing the lignin content between the two forages it was observed that the HPB lignin content was 90% greater than that of the sugarcane. This reflected the lower digestibilities (DM, OM and NDFap) of diets containing HPB, thus limiting intake.
As the plant maturation stage advances, lignification of their vascular system increases (Valente et al. Citation2011b). Because the Alexander palm takes around three years to be able to produce the heart of palm, an excessive deposition of lignin occurs in the plant tissue, reducing the nutritive value of the by-product. Lignin is negatively correlated with the availability of digestible energy in feeds because it makes energy unreachable (Frei Citation2013), justifying the lower metabolizable energy (ME) intake observed with HPB. In addition, lignin is related to a rumen-fill effect (Mertens Citation1987) which causes a reduction in DM intake. The slower passage rate and greater retention time observed in diets with high lignin contents do not improve ruminal fermentation, so a decrease in DM, OM and NDFap digestibilities is detected. The decrease in DM and nutrient intakes associated with HPB was the main cause of the reduction of ME intake.
Another possible reason for the DMI decrease could be the presence of glucosinolates in heart of palm (Hasler Citation1998; Moraes and Colla Citation2006). These compounds are related to intake depression due to its low palatability (Tripathi and Mishra Citation2007; Tripathi and Mishra Citation2017). It is important to note that, despite the negative effects on animal feeding, the glucosinolates are able to increase unsaturated fatty acids (C22:2 and trans C18:1) in carcass and milk when supplemented at adequate quantities (Tripathi and Mishra Citation2007). Unsaturated fatty acids are known as nutraceutical compounds (Santini et al. Citation2018), which have been studied due to its cancer therapeutic properties for humans (Santini et al. Citation2013) and ways to increase it in animal products should be encouraged. Therefore, we believe that it is a topic for further studies on HPB as animal feed.
The lower CP protein intake reduces rumen ammonia production (Russell et al. Citation1992), affecting not only the availability of substrate for bacteria that ferment structural carbohydrates, and thus microbial synthesis, but also urinary nitrogen excretion. A trend observed in EMS, 31.53 and 24.47 g N/ kg digestible OM intake, respectively, for 0% and 22.5% HPB, indicates that lower energy within the rumen of cows fed HPB probably was a factor impairing microbial synthesis.
It was expected that greater levels of HPB than the ones used in this research would compromise animals’ performance. In the pre-trial to define levels of replacement, it was noted that levels greater than 66% of HPB for replacement of sugarcane led to a lower roughage intake by animals (data not shown) due to rumen fill, impairing animal performance. In the pre-trial, we did not observe a reduced DMI with 33% of HPB for replacement of sugarcane, and that was the reason we hypothesized that HPB would not affect intake and production up to 22.5%. However, the pre-trial was performed only for 15 days, and we suspect HPB may have enhanced effects in long term, such as the 60 d of the present trial.
In spite of the reduction on nutrient intake and digestibility observed with the addition of HPB, FCM and ECM were around 22% and 19% greater, respectively, when 7.5% HPB was included in the diet. In addition, when comparing 0% and 7.5% HPB, milk fat was numerically greater in the treatment with HPB. Cows fed fresh sugarcane in high concentrate diets are susceptible to subclinical acidosis because of the high amount of NFC ingested (Souza et al. Citation2017). In addition, Souza et al. (Citation2017) highlighted that Streptococcus bovis, a prevalent bacteria found in the rumen during lactic acidosis, prefer NFC sources other than starch, such as sucrose, the sugarcane soluble sugar. Rodrigues et al. (Citation2017) observed high volatile fatty acids (VFA) concentration in the rumen content of cows fed 40% sugarcane and 60% concentrate with increasing levels of soybean oil. The authors justified VFA values based on the great amounts of sucrose present in sugarcane. Therefore, the inclusion of 7.5% of HPB likely improved ruminal environment, probably stabilizing ruminal pH and leading to greater fermentation parameters, which was observed in greater milk fat percentage, FCM and ECM and greater FCM and ECM efficiencies as well.
The level of 7.5% replacement provided an average gain of 0.36 g per day and was able to improve ruminal fermentation compared to 0%. Considering that the price of HPB is very low or negligible, and that cows could gain weight while increasing FCM and ECM, HPB could be an alternative to decrease production costs, especially for smallholders. In addition, despite HPB’s low quality, it can be used in combination with other feedstuff as long as the diet is adequately balanced to meet the animals’ requirements. On the other hand, at levels of 15% and 22.5%, gains were closer to zero, which indicate that loses in body weight may occur. Moreover, FCM and ECM were smaller than at HPB 7.5%, evidencing that the decrease in ME intake was affecting these cows’ performance.
In conclusion, sugarcane replacement by HPB is detrimental to intake and nutrient digestibility. However, 7.5% replacement level is recommended depending on the milk production level of cows, since this level improves the rumen environment leading to greater FCM and ECM yields at lower production costs. Thus, the use of heart of palm can improve quality of life for milk producers as well as benefit the environment by providing adequate use of the by-products.
Acknowledgements
We acknowledge Prof. Carlos Machado for supplying the residue from palm production.
Disclosure statement
No potential conflict of interest was reported by the authors.
ORCID
Geraldo Fábio Viana Bayão http://orcid.org/0000-0002-4610-8709
Camila Soares Cunha http://orcid.org/0000-0001-9341-9975
Lucas Ladeira Cardoso http://orcid.org/0000-0001-7948-4455
Katiene Régia Silva Sousa http://orcid.org/0000-0002-9945-2706
Marcos Inácio Marcondes http://orcid.org/0000-0003-4843-2809
Additional information
Funding
References
- Bayão GFV, Marcondes MI, Queiroz AC, Pimentel RM, Cardoso LL, Cardoso AJS, Sousa KRS, Batalha CDA. 2017. Chemical composition and fermentative parameters of heart of palm waste produced from Alexander Palm ensiled with chemical additives. Revista Brasileira de Zootecnia. 46(6):489–493. doi:10.1590/s1806-92902017000600003.
- Bayão GFV, Queiroz AC, Freitas SG, Batalha CDA, Sousa KRS, Pimentel RM, Cardoso LL, Cardoso AJS. 2014. Substitution the waste from heart of palm from Alexander Palm (Archontophoenix alexandrae) on sugarcane silage in sheep diets. Archivos Latinos Nutricion. 64:271–276.
- Bergo CL, Negreiros JRS, Miqueloni DP, Lunz AMP. 2013. Repeatability estimates of yield traits in peach palm to palm heart of Putumayo landrace. Revista Brasileira de Fruticultura. 3:829–836. doi: 10.1590/S0100-29452013000300020
- Broderick GA, Clayton MK. 1997. A statistical evaluation of animal and nutritional factors influencing concentrations of milk urea nitrogen. J Dairy Sci. 80:964–2971. doi: 10.3168/jds.S0022-0302(97)76262-3
- Chen XB, Gomes MJ. 1992. Estimation of microbial protein supply to sheep and cattle based on urinary excretion of purine derivatives – an overview of the technical details. Occasional Publication. Rowett Research Institute, Bucksburn, Aberdeen, UK.
- Chizzotti ML, Valadares Filho SC, Valadares RFD, Chizzotti FHM, Tedeschi LO. 2008. Determination of creatinine excretion and evaluation of spot urine sampling in Holstein cattle. Livest Sci. 113:218–225. doi: 10.1016/j.livsci.2007.03.013
- Detmann E, Souza MA, Valadares Filho SC, Queiroz AC, Berchielli TT, Saliba EOS, Cabral LS, Pina DS, Ladeira MM, Azevedo JAG. 2012. Métodos para Análise de Alimentos: INCT – Ciência Animal. 1a Ed. Editora Suprema, Visconde do Rio Branco, Minas Gerais, BRA.
- Detmann E, Valadares Filho SC. 2010. On the estimation of non-fibrous carbohydrates in feeds and diets. Arquivo Brasileiro de Medicina Veterinária e Zootecnia. 62:980–984. doi: 10.1590/S0102-09352010000400030
- Fermino MH, Gonçalves RS, Silveira JRP, Battistin A, Trevisan M, Busnello AC. 2014. Palm fiber as substrate for vegetables. Horticultura Brasileira. 32:404–408. doi: 10.1590/S0102-053620140000400006
- Frei M. 2013. Lignin: characterization of a multifaceted crop component. A review. Scientific World J. 2013:1–25. doi: 10.1155/2013/436517
- Gervasi T, Vito Pellizzeri V, Benameur Q, Gervasi C, Santini A, Cicero N, Dugo G. 2017. Valorization of raw materials from agricultural industry for astaxanthin and □-carotene production by Xanthophyllomyces dendrorhou. Nat Prod Res. 32(13):1554–1561. doi: 10.1080/14786419.2017.1385024
- Hasler CM. 1998. Functional foods: their role in disease in: developing new food products for a changing prevention and health promotion. Food Technol. 52(2):57–62.
- Licitra G, Hernandez TM, Van Soest PJ. 1996. Standardization of procedures for nitrogen fractionation of ruminant feeds. Anim Feed Sci Technol. 57:347–358. doi: 10.1016/0377-8401(95)00837-3
- Mertens DR. 1987. Predicting intake and digestibility using mathematical models of ruminal function. J Anim Sci. 64:1548–1558. doi: 10.2527/jas1987.6451548x
- Mertens DR. 2002. Gravimetric determination of amylase-treated neutral detergente fiber in feeds with refluxing in beaker or crucibles: collaborative study. J AOAC Int. 85:1217–1240.
- Moraes FP, Colla LM. 2006. Functional foods and nutraceuticals: definition, legislation and health benefits. Revista Eletrônica de Farmácia. 3(2):99–112.
- National Research Council. 2001. Nutrient requirements of dairy cattle. 7th ed. Washington: National Academy Press, 408 pp.
- Official Method Am 5–04 Oil – AOCS. 2004. Rapid determination of oil/Fat utilizing high temperature solvent extraction. Association of official analytical chemists, Arlington, USA 3 pp.
- Official Method of Analysis- AOAC. 1990. Association of official analytical chemists. 15th ed., Arlington, USA, 746 pp.
- Oliveira LS, Pereira LGR, Azevedo JAG, Pedreira MS, Loures DRS, Bomfim MAD, Barreiros DC, Brito RLL. 2010. Nutritional characterization of co-product silages of pejibaye. Revista Brasileira de Saúde e Produção Animal. 11:426–439.
- Rodrigues JPP, de Paula RM, Rennó LN, Fontes MMS, Machado AF, Valadares Filho SC, Huhtanen P, Marcondes MI. 2017. Short-term effects of soybean oil supplementation on performance, digestion, and metabolism in dairy cows fed sugarcane-based diets. J Dairy Sci. 100(6):4435–4447. doi:10.3168/jds.2016-11725.
- Rodrigues Neto AJ, Bergamaschine AF, Isepon OJ, Alves JB, Hernandez FBT, Macedo MP. 2001. Effect of additives on nutritive value of silages based on palm cabbage production by-products from Pupunha (Bactris gasipaes). Revista Brasileira de Zootecnia. 30:1367–1375. doi: 10.1590/S1516-35982001000500033
- Romano R, Masucci F, Giordano A, Spagna Musso S, Naviglio D, Santini A. 2010. Effect of tomato by-products in the diet of Comisana sheep on composition and conjugated linoleic acid content of milk fat. Int Dairy J. 20:858–862. doi: 10.1016/j.idairyj.2010.05.006
- Rombola GL, Silva Sobrinho AG, Gonzaga Neto SG, Moro JR, Zeola NMBL, Marques CAT. 2010. Pupunha (bactris gasipaes h.b.k.) by-products as feed for hair sheep. Agropecuária Científica no Semi-árido, 6, 19-26.
- Russell JB, O’Connor JD, Fox DG, Van Soest PJ, Sniffen CJ. 1992. A net carbohydrate and protein system for evaluating cattle diets: I. ruminal fermentation. J Anim Sci. 70:3551–3561. doi: 10.2527/1992.70113551x
- Santini A, Cammarata SM, Capone G, Ianaro A, Tenore GC, Pani L, Novellino E. 2018. Nutraceuticals: opening the debate for a regulatory framework. Br J Clin Pharmacol. 84(4):659–672. doi: 10.1111/bcp.13496
- Santini A, Novellino E, Armini V, Ritieni A. 2013. State of the art of ready-to use therapeutic food: a tool for nutraceuticals addition to foodstuff. Food Chem. 140:843–849. doi: 10.1016/j.foodchem.2012.10.098
- Schmidt P, Rossi Junior P, Toledo LM, Nussio LG, Albuquerque DS, Meduri B. 2010. Fermentative losses and chemical composition of pupunha palm by-products ensiled with chemical additives. Revista Brasileira de Zootecnia. 39:262–267. doi: 10.1590/S1516-35982010000200005
- Silva MP, Arf O, Sá ME, Abrantes FL, Berti CLF, Souza CD, Arruda N. 2014. Straw and coverage levels of nutrients soil for plants coverage seeded in summer for direct seeding bean. Revista Agrarian. 7:233–243.
- Souza JM, Sousa DO, Mesquita BS, Mesquita LG, Silva LFP. 2017. Effect of sugarcane fiber digestibility, conservation method and concentrate level on the ruminal ecosystem of beef cattle. AMB Express. 7:55. Epub 2017 Mar 6. doi: 10.1186/s13568-017-0356-7
- Steinmacher D. 2011. Somatic embryogenesis in pupunha as a strategy for mass multiplication of selected genotypes. I Brazilian Symposium of peach palm. Ilheus, Bahia, BRA.
- Talke H, Schubert GE. 1965. Enzymatic urea determination in the blood and serum in the warburg optical test. Wiener Klinische Wochenschrift. 43:174. doi: 10.1007/BF01484513
- Tripathi MK, Mishra AS. 2007. Glucosinolates in animal nutrition: a review. Anim Feed Sci Technol. 132:1–27. doi: 10.1016/j.anifeedsci.2006.03.003
- Tripathi MK, Mishra AS. 2017. Prospects and problems of dietary glucosinolates in animal feeding. Journal of Advanced Dairy Research. 5(180):1–4. doi:10.4172/2329-888X.1000180.
- Tyrrell HF, Reid JT. 1965. Prediction of the energy value of cow’s milk. J Dairy Sci. 48(9):1215–1223. doi:10.3168/jds.S0022-0302(65)88430-2.
- Valente TNP, Detmann E, Queiroz AC, Valadares Filho SC, Gomes DI, Figueiras JF. 2011a. Evaluation of ruminal degradation profiles of forages using bags made from different textiles. Revista Brasileira de Zootecnia. 40:2565–2573. doi: 10.1590/S1516-35982011001100039
- Valente TNP, Lima ES, Henriques LT, Machado Neto ORM, Gomes DI, Sampaio CB, Costa VAC. 2011b. Anatomy of forage plants and the availability of nutrients for animal ruminants. Veterinária e Zootecnia. 18:347–358.