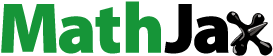
ABSTRACT
Seaweeds are potential feed additives for aquaculture but their effect on fish fatty acid content and profile is largely unknown. This study was, therefore, designed to assess the effect of incorporating green macroalgae seaweed (Ulva sp.) into diets of juvenile dusky kob (Argyrosomus japonicus) on fillet fatty acid profile and concentration. Five experimental diets were formulated to contain seaweed at the following inclusion levels: 0 (Ulva0), 50 (Ulva50), 100 (Ulva100), 150 (Ulva150) and 200 (Ulva200) g/kg commercial kob feed on a dry matter basis. Seventy-seven fingerlings (9.14 ± 0.30 g) were distributed into each of 20 experimental tanks and diets were randomly allocated to tanks such that each diet had 4 replicate tanks holding 77 fingerlings. There was no dietary effect on fillet fatty acids (FA) concentrations (P > .05). The concentration of C18:3n3 (α-linolenic acid) tended to increase with Ulva inclusion levels (P > .05). However, the opposite was observed with the fillet concentrations of both C20:4n6 (arachidonic acid methyl ester) and C20:5n3 (eicosapentaenoic acid (EPA)), but the decrease was also not significant. In conclusion, while Ulva-containing diets did not enhance the FA concentration in dusky kob fillet, the values were similar to those observed in fish fed on the relatively expensive commercial kob diet.
1. Introduction
Historically, fish has always been a major role player in human nutrition, with some studies suggesting that fish provides 20% of protein intake, especially in developing countries (Béné et al. Citation2007). Besides being an inexpensive, rich source of protein, fish are micronutrient- dense and could help eradicate micronutrient deficiency diseases mostly prevalent in impoverished communities around the globe (Roos et al. Citation2003). Some micronutrients such as vitamin D, iodine, selenium, zinc, magnesium and calcium, are more abundant in fish than in livestock animals and plants (Mohanty et al. Citation2017). Fish is also a source of fatty acids (FA), especially omega-3 (n-3) FA, which is widely recognized as an important nutrient for humans. The omega-3 FA, eicosapentaenoic (EPA; 20:5n-3) and docosahexaenoic (DHA; 22:6n-3) are thought to have a wide range of health benefits to humans (Calder Citation2014) and generally, fish is anaffordable source of EPA and DHA in humans diets (Tocher Citation2015). Daily recommendations for EPA and DHA intake differ from one country to another but there is a general consensus that two servings of fish, with one being an oily fish, would be adequate to meet human dietary requirements (Kris-Etherton et al. Citation2002; GOED Citation2014). However, the demand for fish is sky-rocketing due to rapid growth in human population necessitating the need for increased farmed fish. Aquaculture has been recognized as the fastest growing farming sector since the early 90’s, and currently supplies half of the fish and other seafood consumed around the world (FAO Citation2014). The expansion of aquaculture to meet rising demand for fish also means that innovative feeding strategies have to be adopted to reduce feed costs while enhancing growth performance and meat quality attributes. One strategy that can be employed to reduce feed costs is the identification and evaluation of non-conventional feedstuffs such as seaweed that can be incorporated into existing commercial diets. Ideally, the incorporation of such feedstuffs should not only reduce feed costs but should enhance growth performance, health and meat quality traits.
Seaweed is relatively inexpensive and readily available in South Africa. It contains a variety of nutritionally important FA (Evans and Critchley Citation2014) as well as between 18% and 25% crude protein (Makkar et al. Citation2015; Madibana et al. Citation2017), vitamins and minerals. Seaweeds also contain growth-boosting bioactive substances such as polyphenols, which possess antibacterial, antifungal and antiviral properties (Stengel et al. Citation2011). Indeed, inclusion of seaweed in diets of other fish species has been reported to enhance feed utilization and growth (Nakagawa et al. Citation1987). However, the use of seaweed in finfish aquaculture feeds has not been extensively investigated in South Africa.
Dusky kob (Argyrosomus japonicus) has good growth rate, survives in different water salinities (Whitfield Citation1998) and tolerates water with low oxygen levels (Fitzgibbon et al. Citation2007) making it suitable for aquaculture. Under farming conditions, dusky kob is easy to condition and spawn and has a good growth rate, achieving market size in seven to eight months from egg hatching. Because seaweed has been reported to contain significant quantities of FA (Evans and Critchley Citation2014), in the current study we hypothesized that inclusion of seaweed in dusky kob diets would positively modify dietary FA and consequently, fish fillet FA profile and concentration. Therefore, the current study was designed to determine the influence of dietary inclusion of green seaweed Ulva sp. on fillet FA concentration in juvenile dusky kob.
2. Materials and methods
2.1. Study site
This study was carried out at the Marine Research Aquarium of the Department of Agriculture, Forestry and Fisheries (DAFF) in Sea Point (33.9169°S, 18.3875°E), Cape Town, South Africa. The experimental system was a recirculating aquaculture system consisting of 20 black, high-density polyethylene grow-out tanks, (465 L capacity, 67 cm deep and 94 cm diameter) with flattened conical floors coated with white fibreglass resin to allow for better fish visibility. The sea water temperature was maintained at 25°C via a heat pump and dissolved oxygen at 5.5–6.0 mg/l via air lines. The filtration system included protein skimmer or foam fractionator, the sand filter and the biological filtration. Ultra violet lights (55 W) were fitted on the water route between the filtration system and the fish holding tanks.
2.2. Seaweed and experimental fish
Fresh seaweed was collected from an abalone farm in Gansbaai (34.5805° S, 19.3518° E), Western Cape, South Africa. The seaweed is cultured in raceways, which are an extension of the existing abalone grow-out tanks. The effluent from the abalone tanks (after sediment is removed) flows through four paddle raceways. After passing through the seaweed raceways, approximately 50% of the effluent water is re-circulated to the abalone tank. Dusky kob fingerlings were sourced from a commercial fish farm based in Mtunzini (28.9597°S, 31.7501°E), Kwa-Zulu Natal, off the South African east coast. A high-protein (48%) commercial fishmeal diet (Marifeed Pty Ltd) was offered to the fish during a two week acclimatization period. Seventy seven fish (9.14 ± 0.30 g) were randomly distributed into each of the 20 tanks in preparation for the commencement of the experiment. Ethical clearance was obtained from North-West University’s Animal Research Ethics Committee (NWU-00691-17-S9).
2.3. Feeding experiment
Five experimental diets were formulated as described by Madibana et al. (Citation2017) to contain seaweed meal at the following inclusion levels:0, 50, 100, 150, 200 g/kg commercial kob feed (Ulva0, Ulva50, Ulva100, 150, and Ulva200, respectively) (). Dietary treatments were randomly allocated to four replicate tanks at 2.8% of the fish body weight. Ten fish from each of the 20 tanks were randomly sampled for weekly weight measurements for a period of nine weeks. At trial termination, a pooled sample of 10 fish from each tank were sacrificed and filleted. The fillets were stored in a freezer (−80°C) pending fatty acid extraction and analysis.
Table 1. Ingredients and proximate composition of the experimental diets.
2.4. Fatty acid extraction and analyses
Seaweed meal, dietary treatments and fillet samples were processed for fatty acids determination. Fat was extracted using a 2:1 (v/v) chloroform: methanol solution (Folch et al. Citation1957), which contained 0.01% butylated hydroxytoluene (BHT) as an antioxidant. The samples were homogenized in the extraction solvent for 30 s using a polytron mixer (Wiggen Hauser D-500 Homogeniser, fitted with a standard shaft 1, speed setting D). Heptadecanoic acid (C17:0) was employed as an internal standard (catalogue number H3500, Sigma-Aldrich, Gauteng, South Africa) to quantify the individual fatty acids present in each muscle sample. A 250 μL sub-sample of the extracted lipids was subsequently transmethylated at 70°C for 2 h using 2 mL of a 19:1 (v/v) methanol: sulphuric acid solution as the transmethylating agent. After allowing the resultant mixtures to cool to room temperature, the fatty acid methyl esters (FAME) were extracted with water and hexane (Dodds et al. Citation2004). Following separation of the distilled water and FAME-containing hexane fluids, the top hexane layer was transferred to a spotting tube and dried under nitrogen. One hundred μL hexane was then added to each dried FAME sample, of which 1 μL was injected into the gas chromatograph. The FAMEs were analysed using a Thermo TRACE 1300 series gas-chromatograph (Thermo Electron Corporation, Milan, Italy) equipped with a flame-ionization detector, using a 30 m TR-FAME capillary column with an internal diameter of 0.25 mm and a 0.25 µm film (Cat. No. HY260M142P, Anatech, Cape Town, South Africa) and a run time of ca. 40 min. The following oven temperature settings were utilized: initial temperature of 50°C (maintained for 1 min) and final temperature of 240°C attained after three ramps (initial increase at a rate of 25°C/min) until a temperature of 175°C was reached; thereafter an immediate increase at a rate of 1.5°C/min to reach 200°C and maintenance of this temperature for 6 min; lastly an increase at a rate of 10°C/min to reach 240°C and maintenance of this temperature for a minimum of 2 min. The injector temperature was set at 240°C and the detector temperature at 250°C. The hydrogen gas flow rate was 40 mL/min. The FAME of each sample was identified by comparing the retention times with those of a standard FAME mixture (Supelco™ 37 Component FAME mix, Cat no. CRM47885, Supelco, USA), with results being expressed as mg fatty acid/g meat/diet. The ratios were calculated as follows:
2.5. Statistical analysis
The FA concentration data was subjected to a one-way ANOVA. Measurements from 10 fish per tank (four replicate tanks per treatment) were averaged before analysis, such that each tank had one value. Data analysis was performed using SAS statistical software (Citation2010) according to the following statistical linear model:where Yi j= dependant variable (fatty acid concentration), µ = population mean, Di = effect of diet, and Eij = random error associated with observation ij, assumed to be normally and independently distributed. For all statistical tests, significance was declared at P ≤ .05. Least squares means were compared using the probability of difference (pdiff) option in the lsmeans statement of SAS.
3. Results
3.1. Fatty acids: Ulva and formulated diets
A total of 14 saturated fatty acids (SFA) were detected in the five dietary treatments and the raw Ulva seaweed (). The C16:0 (palmitic acid) was the most abundant SFA. The control (Ulva0) diet recorded the highest numerical value for the palmitic acid (2.74 ± 0.43 mg/g diet) and Ulva150 recorded the lowest numerical value (2.51 ± 0.438 mg/g diet). The Ulva0 diet had the highest ΣSFA (4.17 ± 1.31 mg/g diet) with Ulva150 recording the lowest ΣSFA (3.95 ± 1.71 mg/g diet). Only seven monounsaturated fatty acids (MUFA) were detected in all the dietary treatments (). Concentration of C16:1 (palmitoleic acid), C24:1 (nervonic acid) and C18:1n9c (oleic acid methyl ester) tended to decrease with an increase in Ulva inclusion. Ulva0 had the highest ΣMUFA (3.31 ± 1.45 mg/g diet) while Ulva200 had the lowest ΣMUFA (2.38 mg/g diet). A total of nine polyunsaturated fatty acids were detected in all the dietary treatments (). There was a tendency of an increase in the concentration of C18:3n3 (α-linolenic acid) with an increase in Ulva inclusion in the diets (P > .05). However, the opposite was observed with the concentrations of both C20:4n6 (arachidonic acid methyl ester) and C20:5n3 (eicosapentaenoic acid (EPA)) (P > .05). As observed with SFA, the control diet recorded the highest ΣPUFA (2.64 ± 1.33 mg/g diet) while the Ulva150 recorded the lowest ΣPUFA (1.67 ± 0.83 mg/g diet). Overall, there was a decrease in fatty acid concentrations as Ulva inclusion increased in the diets, but not significant (P > .05).
Table 2. Saturated and mono-unsaturated fatty acid content (mg/g diet) of the experimental diets
Table 3. Polyunsaturated fatty acid content (mg/g diet) of the experimental diets.
3.2. Fillet fatty acids
A total of 11 SFA were detected in the fillet samples of all fish groups fed the five dietary treatments (). Dietary treatments did not influence the concentration of all the identified SFA (P > .05). A non-significant decrease in the concentration of C13:0 (tridecanoic acid) was evident as Ulva inclusion increased, with control fed group recording 0.43 ± 0.14 mg/g meat while Ulva200 group recorded much lower concentrations, only detectable in microgram units. The C24:0 (methyllignocerate) concentration in fish fillet tended to increase with an increase in dietary inclusion of Ulva up to 150 g seaweed/kg kob feed. Fillet from Ulva0-fed fish had a C24:0 concentration of 1.02 ± 0.34 mg/g meat while those fed Ulva50 (1.04 ± 0.35 mg/g meat) and Ulva150 fish (1.44 ± 0.10 mg/g meat) had higher levels. Fillet SFA concentration in Ulva200 fed group was numerically lower than in fillet from other dietary treatments.
Table 4. Saturated and mono-unsaturated fatty acid content (mg/g meat) of dusky kob fillets fed Ulva-based dietary treatments.
A total of seven MUFA were detected from all the fish fed the five different dietary treatments (). However, there was no dietary effect on the fillet concentration of all the MUFA. There was no clear trend in the effect of dietary treatments on any of the FA, but lower ΣMUFA concentration (5.45 ± 1.59 mg/g meat) was observed when fish were fed Ulva200 diet. The highest numerical ΣMUFA was recorded for Ulva150 fed group (15.07 ± 6.47 mg/g meat).
Nine PUFA were detected in fillet samples across all five dietary treatments (). There was also no significant dietary treatment effect on the concentration of all the PUFA (P > .05). No clear trend was observed with regards to the influence of graded levels of Ulva. The ΣPUFA was lower for the group fed Ulva200 diet when compared to the rest of the diets (P > .05). Fish fed Ulva150 recorded the highest numerical value for Σn-6 FA (3.56 ± 1.45 mg/g meat), with Ulva200 fed group recording the lowest (1.47 ± 0.50 mg/g meat). The same trend was observed for Σn-3 FA, with Ulva150 fed group recording the highest numerical value (4.64 ± 2.49 mg/g meat) and Ulva200 fed group recording the lowest numerical value (1.21 ± 0.49 mg/g meat). Ulva150 group recorded the highest total FA (43.72 ± 1.59 mg/g meat) while Ulva200 (19.83 ± 2.68 mg/g meat) fish recorded the lowest total FA.
Table 5. Polyunsaturated fatty acids content (mg/g meat) of dusky kob fillets fed Ulva-based dietary treatments.
4. Discussion
4.1. Fatty acids: Ulva and diets
The local Ulva sp. used in this study had 50% SFA; 19.7% MUFA; 30.3% PUFA; 8.03% total n-6 PUFA; 20.7% total n-3 PUFA and 0.39 n-6/n-3 ratio. Contrasting and fairly consistent results were observed by Kendel et al. (Citation2015) in Ulva armoricana that contained 46.5% SFA; 24.3% MUFA; 29.2% PUFA; 3.7% total n-6 PUFA; 23.9% total n-3 PUFA and 0.1 n-6/n-3 ratio. Varying FA results between the two seaweeds belonging to the same genus and probably the same species, may be attributed largely to different growth environments; the Mediterranean climate of Western Cape, South Africa (current study) and the maritime climate of Brittany France (Kendel et al. Citation2015 study) as well as water salinity and temperature (Mišurcová Citation2012). Processing methods prior to the extraction of the FA may also explain varying FA concentrations. Results of the current study also contrasted with a report by Floreto et al. (Citation1996) who reported 1.4% of total n-6 and 10.8% of total n-3 from Ulva seaweed.
There is extensive literature on inclusion of Ulva species in experimental diets for different aquaculture fish species as discussed in our earlier study (Madibana et al. Citation2017). However, there is limited information regarding the concentration of FA of the Ulva-based diets used in those studies. As expected, the most abundant SFA in dietary treatments from the current study was C16:0 (palmitic acid), constituting approximately 50% of the total SFA in all the treatments. Ragaza et al. (Citation2015) reported palmitic acid levels as high as 78% of total SFA in diets for juvenile Japanese flounder (Paralichthys olivaceus) supplemented with red seaweed (Eucheuma denticulatum) at 3%, 6% and 9% inclusion levels. The current dietary treatments showed a minimal decrease in SFA with an increase in Ulva inclusion, but Ragaza et al. (Citation2015) reported a minimal increase in SFA with an increase of E. denticulatum in the diets. Considering that the current study used Ulva seaweed at a higher inclusion rate than that for E. denticulatum in Ragaza et al. (Citation2015)’s study, the difference in the effect on SFA maybe be due to different bioactive compounds these two seaweeds contain. Theoretically, it was expected that addition of seaweed, which already contains SFA to a fishmeal base diet, would not result in a decline in the concentration of SFA. However, since the concentration of Ulva FA in this study was low, the lower SFA upon Ulva inclusion indicates that Ulva inclusion slightly diluted FA concentration of the basal diet. The same trend of lower FA concentration in the diets with higher Ulva inclusion was also evident for MUFA and PUFA, whereas, again, Ragaza et al. (Citation2015) reported the opposite. When verdemin (an algal product derived from Ulva ohnoi) was included in diets for juvenile Atlantic salmon, the following FA prolife was recorded: 33.78% SFA (lower than the current Ulva supplemented diets); 46.74% MUFA(higher than the current Ulva supplemented diets) and 19.49% PUFA (lower than the current Ulva supplemented diets) (Norambuena et al. Citation2015). The results suggest that the Ulva sp. used in the current study is rich in FA as compared to Ulva ohnoi.
4.2. Fillet fatty acids
Our previous report on the effect of Ulva seaweed on growth performance in dusky kob (Madibana et al. Citation2017) indicate that 50 g seaweed/kg diet is the upper limit for Ulva inclusion in dusky kob diets. This could be because carnivorous species such as dusky kob do not normally encounter plant material in their natural diets, which explains the slower growth observed when seaweed inclusion exceeded 50 g/kg diets. Indeed, Red tilapia (herbivorous specie) that utilize plant in their natural diets experienced weight gain much higher inclusion level (150 g/kg diet) of Ulva sp. (El-Tawil Citation2010). Even though natural resources such as seaweed could serve as affordable fish feed ingredients that do not compromise fish growth, it is imperative to investigate their impact on one of fish most valuable nutrient, fatty acids. A negative effect on the fatty acids concentration in fish (fillets) jeopardizes food and nutrition security and, consequently, human health.
The nutritional properties of fish render them indispensable foodstuffs that are beneficial to human health. In recent years, most of the focus has been directed on the significance of n-3 PUFA in human daily nutrition, particularly the essential n-3 PUFA eicosapentaenoic acid (EPA) and docosahexaenoic acid (DHA). These two PUFAs are thought to prevent human coronary artery disease (Gunasekera et al. Citation1999). The PUFA concentrations tend to vary among different fish species, but there are significant human health benefits from increased consumption of fish because of their rich composition of n-3 PUFA and poor in n-6 PUFA series (Sargent Citation1997).
This study was guided by the knowledge that fish (finfish) obtain their FA from algae (macro and microalgae) whether wild or farmed. Fish larvae consume zooplanktons (which feed mostly on algae) during their developmental stage before feeding on other marine vertebrates or formulated feed. Halver (Citation1980) highlighted factors such as water temperature, water salinity and diets that may influence fish FA concentration. This study focussed entirely on the effects of Ulva-based diets on the concentration of FA in fish fillets. The current dietary treatments and the seaweed Ulva possessed all the required fatty acids, but the effects of the diets on fillet FA concentrations were not statistically significant, even though Ulva inclusion at 200 g seaweed/kg kob feed (highest inclusion rate) seemed to lower FA concentrations. This is the first ever report on the effect of dietary inclusion of Ulva on dusky kob fillet FA concentration. In agreement with our findings, Norambuena et al. (Citation2015) reported that including algal products, verdemin (derived from Ulva ohnoi) and rosamin (derived from diatom Entomoneisspp.), in diets for juvenile Atlantic salmon did not affect FA composition of fish. Ragaza et al. (Citation2015) also reported the same observation when feeding diets with incremental levels of red seaweed (Eucheuma denticulatum) in juvenile Japanese flounder (Paralichthys olivaceus). In all these three studies fish were not reared until the adult stage (sexual maturity). Most fish in the markets are sold at adult stage, but most experimental systems cannot cope with raising fish to market size due to space limitation, time constraint and cost of feed. Future studies should, therefore, be carried out at a grow-out facility to determine, conclusively, whether seaweed inclusion in fish diets might boost fillet FA concentration.
The DHA content of most fish, including Catla catla, Tenualosa ilisha, Cirrhinusm rigala, Oncorhycus mykiss, Puntius sophore and some shellfish from Indian waters, varies from 0% to 28.55% of total fat, whereas the EPA content varies between 0% and 10.6% of the total fat (Mohanty et al. Citation2017). The average EPA concentration recorded from this study was 7.5% of the total fat and the DHA concentration was very low (<0.01 mg/g meat). Even though it contains lower amount of DHA, dusky kob could still be an important source of EPA for the Eastern coastal communities of South Africa.
5. Conclusions
In conclusion, dietary inclusion of Ulva did not affect FA content of the South African juvenile dusky kob as feeding Ulva-containing diets resulted in fish fillet with similar FA concentration as the fillet from fish fed the commercial diet (Ulva0). However there was a linear decrease in the concentration of a few FA with an increase in Ulva in the diets. For this reason, Ulva supplementation must be capped at 50 g/kg to avoid any significant reduction in fatty acid content of dusky kob fillet. Additional studies using older fish are necessary to investigate age-related variation in FA concentration in fish fed seaweed-containing diets.
Acknowledgements
The authors would like to thank the I&J Limited (Gansbaai) for donating fresh seaweed and the Department of Agriculture, Forestry and Fisheries for material support. Molatelo Junior Madibana designed the study, conducted the feeding trials and wrote the paper. Victor Mlambo designed the study, statistically analysed the data and wrote the paper. Brett Rhoderick Lewis designed the study, conducted the feeding trials and proof read the manuscript. Lisa Uys extracted, analysed the samples for fatty acid concentration and edited the materials and methods section for Fatty acids extraction and analyses.
Disclosure statement
No potential conflict of interest was reported by the authors.
ORCID
Molatelo Junior Madibana http://orcid.org/0000-0001-7385-7660
Victor Mlambo http://orcid.org/0000-0002-0799-3899
References
- Béné C, Macfayden G, Allison EH. 2007. Increasing the contribution of small-scale fisheries to poverty alleviation and food security. FAO Fisheries Technical paper no. 481, FAO, Rome.
- Calder PC. 2014. Very long chain omega-3 (n-3) fatty acids and human health. Eur J Lipid Sci Technol. 116:1280–1300. doi: 10.1002/ejlt.201400025
- Dodds ED, McCoy MR, Geldenhuys A, Rea L D, Kennish JM. 2004. Microscale recovery of total lipids from fish tissue by accelerated solvent extraction. J Am Oil Chem. Soc. 81:835–840. doi: 10.1007/s11746-004-0988-2
- El-Tawil NE. 2010. Effects of green seaweeds (Ulva sp.) as feed supplements in red tilapia (Oreochromis sp.) diet on growth performance, feed utilization and body composition. J Arabian Aquac Soc. 5:179–193.
- Evans FD, Critchley AT. 2014. Seaweeds for animal production use. J Appl Phycol. 26:891–899. doi: 10.1007/s10811-013-0162-9
- FAO. 2014. The state of the world fisheries and aquaculture 2012. Rome: FAO.
- Fitzgibbon QP, Strawbridge A, Seymour RS. 2007. Metabolic scope, swimming performance and the effects of hypoxia in the mulloway (Argyrosomus japonicus). Aquaculture. 270:358–368. doi: 10.1016/j.aquaculture.2007.04.038
- Floreto EAT, Teshima S, Koshio S. 1996. The effects of seaweed diets on the lipid and fatty acids of the Japanese disc abalone (Haliotis discus hannai). Fish. Sci. 62:582–588. doi: 10.2331/fishsci.62.582
- Folch J, Lees M, Stanley GHS. 1957. A simple method for the isolation and purification of total lipids from animal tissues. J Biol Chem. 226:497–509.
- Global Organisation for EPA and DHA (GOED). 2014. Global recommendations for EPA and DHA intake. Review 19.
- Gunasekera RM, de Silva SS, Ingram BA. 1999. Early ontogeny-related changes of the fatty acid composition in the Percichthyid fishes trout cod (Maccullochella macquariensis) and Murray cod (Maccullochella peelii). Aquat. Living Resour. 12:219–227. doi: 10.1016/S0990-7440(00)88472-7
- Halver JE. 1980. Fish feed technology. ADCP/REP/80/11. FAO, Rome, Italy, 395 pp.
- Kendel M, Wielgosz-Collin G, Bertrand S, Roussakis C, Bourgougnon N, Bedoux G. 2015. Lipid composition, fatty acids and Sterols in the seaweeds Ulva armoricana, and Solieriachordalis from Brittany (France): an analysis from nutritional, chemotaxonomic, and proliferative activity perspectives. Mar Drugs. 13:5606–5628. doi: 10.3390/md13095606
- Kris-Etherton PM, Harris WS, Appel LJ. 2002. Fish consumption, fish oil, omega-3 fatty acids, and cardiovascular disease. Circulation. 106:2747–2757. doi: 10.1161/01.CIR.0000038493.65177.94
- Madibana MJ, Mlambo V, Lewis BR, Fouche CH. 2017. Effect of graded levels of dietary Ulva sp on growth, hematological and serum biochemical parameters in dusky kob, Argyrosomus japonicus. Egypt J Aquat Res. 43:249–254. doi: 10.1016/j.ejar.2017.09.003
- Makkar HPS, Tran G, Heuzé V, Giger-Reverdin S, Lessire M, Lebas F, Ankers P. 2015. Seaweeds for livestock diets: a review. Anim Sci Technol. 212:1–17.
- Mišurcová L. 2012. Chemical composition of seaweeds. In: Se-Kwon Kim, editor. Handbook of marine macroalgae: biotechnology and applied phycology. Wiley; 567 pp.
- Mohanty BP, Mahanty A, Ganguly S, Mitra T, Karunakaran D, Anandan R. 2017. Nutritional composition of food fishes and their importance in providing food and nutritional security. Food Chem. DOI:10.1016/j.foodchem.
- Nakagawa H, Kasahara S, Sugiyama T. 1987. Effect of Ulva meal supplementation on lipid metabolism of Black Sea bream (Acanthopagrus schlegeli, Bleeker). Aquaculture. 62:109–121. doi: 10.1016/0044-8486(87)90315-2
- Norambuena F, Hermon K, Skrzypczyk V, Emery JA, Sharon Y, Beard A. 2015. Algae in fish feed: performances and fatty acid metabolism in juvenile Atlantic salmon. PLoS ONE. 10(4):e0124042. doi: 10.1371/journal.pone.0124042
- Ragaza JA, Koshio S, Mamauag RE, Ishikawa M, Yokoyama S, Villamor SS. 2015. Dietary supplemental effects of red seaweed (Eucheuma denticulatum) on growth performance, carcass composition and blood chemistry of juvenile Japanese flounder (Paralichthys olivaceus). Aquacult. Res. 46:647–657. doi: 10.1111/are.12211
- Roos N, Islam M, Thilsted SH. 2003. Small fish is an important dietary source of vitamin A and calcium in rural Bangladesh. Int. J. Food Sci. Nutr. 54:329–339. doi: 10.1080/09637480120092125
- Sargent JR. 1997. Fish oils and human diet. Brit. J. Nutr. 78:5–13. doi: 10.1079/BJN19970131
- SAS. 2010. Users guide. Carry, NC: Statistical Analysis System Institute Inc.
- Stengel DB, Connan S, Popper ZA. 2011. Algal chemodiversity and bioactivity: sources of natural variability and implications for commercial application. Biotechnol. Adv. 29:483–501. doi: 10.1016/j.biotechadv.2011.05.016
- Tocher DR. 2015. Omega-3 long-chain polyunsaturated fatty acids and aquaculture in perspective. Aquaculture. 449:94–907. doi: 10.1016/j.aquaculture.2015.01.010
- Whitefield AK. 1998. Biology and ecology of fishes of South African estuaries. Ichthological monographs of the JLB Smith Institute of Ichthyology. 2:1–223.