ABSTRACT
Two experiments were conducted to evaluate the inclusion of feed enzymes into beef cattle creep feeds. In the first one, the following treatments were tested in vitro: (1) 100% bermudagrass (GRASS); (2) a mixture of 75% bermudagrass and 25% creep feed (CREEP); (3) CREEP enhanced with endo-1,4-β-xylanase (XYLAN); (4) CREEP enhanced with endo-1,3(4)-β-glucanase (BGLUC). Degradation of fibre was greatest (P ≤ 0.03) for BGLUC, whereas in vitro dry matter digestibility was greatest (P = 0.01) for XYLAN. In the second experiment, cow-calf pairs from 2 farms were split into 3 groups: (1) Group with no supplementation of calves (NO FEED); (2) Group where calves were supplemented in a creep feeding system (PLAIN FEED); and (3) Same feed regimen as PLAIN FEED, but with the addition of endo-1,4-β-xylanase due to the positive results observed in experiment 1 (ENZYME FEED). There was a numerical increase in calf average daily gain in the 2 groups that were supplemented, however, only ENZYME FEED was significantly different (P ≤ 0.03) than NO FEED. At one farm, supplement gain:feed was improved (P = 0.01) in ENZYME FEED, compared to PLAIN FEED. Collectively, these results indicate that xylanase can be used to enhance beef cattle creep feeds.
1. Introduction
Exogenous feed enzymes are frequently used as feed additives to enhance the rations of poultry and swine; however, the use of these additives in beef cattle diets is not a common practice. Recent improvements in their manufacturing process have allowed feed enzymes to become more economically viable (Balci et al. Citation2007; Meale et al. Citation2014), so products that are now commercially available may help producers increase the profitability of their operations. The feed enzymes more suitable for ruminants normally contain xylanase and/or cellulase activities resulting from bacterial or fungal fermentations. These compounds have the potential to enhance fibre digestion in the rumen and improve feed efficiency (Beauchemin et al. Citation2003; He et al. Citation2014). Still, factors such as the moisture level of the feed, diet pH, the time required for enzymes to interact with the substrates, and many others, can all influence the observed animal performance responses to feed enzymes (Beauchemin et al. Citation1995).
Previous studies on applied nutrition have demonstrated that pre-weaning supplementation of beef cattle (or creep feeding) can be a great tool for improving calf growth rates and weaning weights (Prichard et al. Citation1989; Tarr et al. Citation1994; Viñoles et al. Citation2013). However, the efficiency by which calves convert creep feeds into body weight (BW) gain may limit the success of this kind of supplementation. Moreover, the poorer feed conversion normally observed when unlimited creep feed is offered to calves can be attributed to a substitution effect and lower fibre digestibility, leading to a decrease in forage use (Moriel and Arthington Citation2013). Since beef calves are usually introduced to forages at early ages, their forestomach is still not fully developed when they start grazing (Church Citation1979). Consequently, digestibility of forages by these animals is expected to be lower than what is normally seen in adult beef cattle, so one viable option to overcome this problem may be the inclusion of feed enzymes in the diets of young cattle.
To our knowledge, there is no published research on the utilization of feed enzymes in beef cattle creep feeds. Therefore, the objectives of the present study were: (1) using an in vitro batch culture setting, to identify the best option between 2 enzymes [endo-1,4-β-xylanase, and endo-1,3(4)-β-glucanase] for inclusion in creep feeds; and (2) to perform an on-farm evaluation of the best creep feeding (the best option according to the in vitro results). To that end, two separate experiments were carried out: Experiments 1 and 2, as described below. Our hypothesis was that the addition of feed enzymes to the creep feeds would improve desirable traits such as degradability of fibre and total dry matter (in the in vitro trial), and calf growth rates and weaning weights (in the on-farm trial).
2. Materials and methods
As previously mentioned, this research was divided into 2 experiments. Experiment 1 consisted of an in vitro trial in which 2 enzymes were evaluated as feed additives in beef cattle creep feeds. Experiment 2 consisted of an on-farm trial in which beef calves were offered creep feeds with and without the addition of a feed enzyme, and results were compared to a control (non-creep fed group). All procedures involving animals were verified and approved by the University of Georgia’s Office of Animal Care and Use (Animal Use Protocol #A2015 07–018-Y1-A0).
2.1. Experiment 1
In order to properly emulate the digestive processes that takes place in the rumen of beef calves during their suckling phase, an in vitro batch culture trial was carried out using rumen fluid obtained from 6-month-old suckling beef calves. In this trial, a total of 4 different treatments were incubated for a period of 24 h. These treatments were formulated using a combination of bermudagrass (Cynodon dactylon), a creep feed, and 2 selected carbohydrases: endo-1,4-β-xylanase (EC/IUB No. 3.2.1.8) and endo-1,3(4)-β-glucanase (EC/IUB No. 3.2.1.6). These enzymes were added to a forage:concentrate mix composed of 75% bermudagrass and 25% creep feed. This ratio was set on a dry matter basis and intended to mimic the daily dry matter intake (DMI) of a beef calf supplemented with a moderate amount of creep feed. The forage used in this study was collected from a plot that was harvested at 4-week intervals, and the creep feed was composed mainly of corn and soybean meal (). Thus, the four treatments used in the study were: (1) 100% bermudagrass (GRASS); (2) a mixture of 75% bermudagrass and 25% creep feed (CREEP); (3) CREEP enhanced with endo-1,4-β-xylanase at 3000 units/kg of dry matter (XYLAN); (4) CREEP enhanced with endo-1,3(4)-β-glucanase at 200 units/kg of dry matter (BGLUC).
Table 1. Composition of the creep feed used in Experiments 1 and 2*.
2.1.1. Substrate preparation
Prior to being used, each feed ingredient (except the enzymes) was ground to pass a 2-mm screen using a Model 4 Wiley Mill (Thomas Scientific, Swedesboro, NJ). The ground ingredients were then combined accordingly to prepare the four treatments previously described. Once all treatments were prepared, 1.4 g of each was placed into 250-mL glass bottles, which were used to determine the production of gas and volatile fatty acids (VFA) during the 24 h of fermentation. A total of 5 bottles per treatment were used (5 replications). In addition to the glass bottles, a separate set of 125-mL plastic scintillation vials was also prepared for the incubations. The plastic bottles were used exclusively to determine in vitro dry matter digestibility (IVDMD) and fibre degradability. They contained half the amount of treatment used in the glass bottles: 0.7 g each. A total of 5 plastic scintillation vials were used for each treatment. In order to determine the blank contributions and make the proper adjustments, an additional set of five 250-mL glass bottles and five 125-mL plastic vials were used in incubations.
2.1.2. Preparation of the inoculum
The inoculum used in all incubations was prepared by mixing McDougall’s buffer (McDougall Citation1948) and rumen fluid in a 2:1 ratio. The rumen fluid was collected by oesophageal tubing from 12 six-month-old beef calves (6 males and 6 females; 186 ± 7 days-old) which were still in the suckling phase and raised on a forage-based regimen. Approximately 600 mL of fluid were collected from each animal and immediately placed in a sealed thermos to be transported to the laboratory, where it was further processed. Processing began by straining the fluid through a 500-micron nylon mesh to remove any feed particles and then combining all the strained fluid into one mixture. The McDougall’s buffer was then added to this mixture and the container was placed in a water bath at 39°C, where CO2 was bubbled into it for 5 min. Following, each one of the previously prepared 250-mL glass bottles received 100 mL of this inoculum. The bottles were then gassed with CO2, sealed with rubber stoppers, and placed in a water bath incubator at 39°C for 24 h. The 125-mL plastic vials were inoculated following the same protocol, but with 2 modifications: the amount of inoculum used was 50 mL, and their tops were sealed with rubber stoppers fitted with a 16-gauge needle to allow gas release.
2.1.3. Collection and analysis of gases
Collection of fermentation gases were conducted at 3-hour intervals on all of the 250-mL glass bottles using a water displacement method (Lourenço et al. Citation2017). Briefly, this method consisted of inserting one 22-gauge needle into the incubation bottle, and another one into a water-filled bottle that was connected to a 60-mL syringe by a 3-way valve. Gas production was measured by allowing the gas to flow from the incubation bottle to the 60-mL syringe. In this process, gas pressure moved the syringe plunger until pressure was equilibrated. The incubation bottle was swirled to allow all the produced gas to escape. Once the plunger stopped moving, the syringe reading was recorded. The 3-way valve was then turned to direct the collected gas into the water-filled bottle. During this procedure, an extra 22-gauge needle was inserted into the water-filled bottle to allow displacement of water and capture of gas inside the bottle, which was upside down. The apparatus was then disconnected and the water bottle containing the gas was kept upside down until analysis of its gas content was carried out. Compositional analysis of the collected gas was performed for CH4 by gas chromatography. Ten mL of gas sampled from each bottle were injected in an Agilent 7820A gas chromatograph (Agilent Technologies, Santa Clara, CA) equipped with a 0.25 mL sample loop and a flame ionization detector. A capillary column (Molsieve, 5A, 25 m × 0.32 mm, Varian CP7536) was used. The injector, oven, and detector temperatures were set at 80°C, 160°C, and 200°C, respectively. Total gas produced in 24 h, bottle headspace, and bottle volume were also considered in the calculations of CH4 concentrations.
2.1.4. Analysis of volatile fatty acids
Upon conclusion of the 24-h fermentation, the 250-mL glass bottles were opened and their contents were transferred to polypropylene tubes, which were immediately frozen at −20°C. One week later, these samples were thawed and centrifuged for 10 min at 10,000×g at 4°C. Then, 2 mL of the supernatant was pipetted into centrifuge tubes along with 0.4 mL of a metaphosphoric acid:crotonic acid (internal standard) solution, and samples were frozen overnight. On the next day, samples were once again thawed and centrifuged for 10 min at 10,000×g at 4°C. The supernatant was transferred into vials and mixed with ethyl acetate in a 2:1 ratio of ethyl acetate to supernatant. After 5 min, a subsample of the supernatant was transferred to a screw-thread vial for analysis of VFA in an Agilent 7820A gas chromatograph (Agilent Technologies, Santa Clara, CA) equipped with a flame ionization detector and a capillary column (CP-WAX 58 FFAP 25 m × 0.53 mm, Varian CP7767). Sample injection volume was 0.50 μL. The column temperature was maintained at 110°C, and the injector and detector temperatures were 200°C and 220°C, respectively. Nitrogen was used as the carrier gas, set at a flow rate of 40 mL/min.
2.1.5. Analysis of in vitro digestibility
Digestibility analyses were performed both for total dry matter and for the fibre portion of the treatments. Briefly, once the 24 h fermentation was concluded, all the 125-mL plastic scintillation vials were capped and placed in a freezer at −20°C. One week later, they were freeze-dried at −50°C for 36 h (FreeZone 6, Labconco Corporation, Kansas City, MO), and then placed in a drying oven for 16 h at 100°C (Blue M Electric Company, Blue Island, IL). The IVDMD was obtained by difference between initial and final incubated dry matter (DM) mass, corrected by blank contributions. Next, the dried samples were mashed and 0.5 g were placed into filter bags (F57 filter bags, Ankom Technology, Macedon, NY). These samples and the original treatments (prior to the 24 h fermentation) were both subjected to analysis of neutral detergent fibre (NDF) and acid detergent fibre (ADF), and results were compared to calculate the in vitro disappearance (which was considered the digestibility) of these fibre portions. Similarly, the contents of the plastic scintillation vials incubated as blanks were analysed for their NDF and ADF contents, and results were utilized in the adjustments of treatment NDF and ADF digestibilities.
2.1.6. Statistical analysis
Statistical analyses were carried out using the software Minitab® (version 18.1). Analysis of variance was performed using treatment and replicate bottle (or vial) as factors. The 250-mL glass bottles or the 125-mL plastic scintillation vials were considered the experimental unit. Contrasts were assessed using Tukey’s honest significant difference test. Differences were considered significant at P ≤ 0.05, and were treated as trends when 0.05 < P < 0.10.
2.2. Experiment 2
2.2.1. Animals, diets and management
The second experiment was an on-farm study carried out using spring-born calves over 3 consecutive years (2016, 2017 and 2018) beginning in the end of May and ending in the first half of September of each year. It was conducted at 2 different research stations: the first one located in Eatonton, GA (University of Georgia Eatonton Beef Research Unit; 33°24 N, 83°29 W), and the second in Alapaha, GA (University of Georgia Alapaha Range Grazing Unit; 31°21 N, 83°13 W). At the first station (Eatonton, GA), a total of 75 steers (199.9 ± 34 kg) and 22 heifers (186.6 ± 28 kg) were used; while at the second station (Alapaha, GA) only steers were used: a total of 84 (168.5 ± 26 kg). On both farms, the cow-calf herd was divided into three groups, namely: (1) Group with no supplementation of calves (NO FEED); (2) Group in which the calves were supplemented in a creep feeding system (PLAIN FEED); and (3) Group in which the calves were fed the same supplement offered in PLAIN FEED but with the addition of the fibrolytic enzyme endo-1,4-β-xylanase (ENZYME FEED). The enzyme added to this treatment was included at 13,800 fungal xylanase units/kg DM of the supplement. The commercial product (RONOZYME® WX, DSM Nutritional Products) was granulated and had an average particle size of 600 μm. Its main activity was a heat-stable endo-1,4-β-xylanase from Thermomyces lanuginosus (EC/IUB No. 3.2.1.8). The commercial enzyme used in ENZYME FEED was selected based on the cost per kg, and on the results from our previous in vitro screening trial (experiment 1), in which increased IVDMD was observed when this enzyme was used. Thus, in this second experiment, the concentration of xylanase was adjusted in the ENZYME FEED treatment to account for the total DMI expected from suckling calves, while providing the same amount of xylanase that calves would consume if they were fed the XYLAN treatment from experiment 1. Therefore, XYLAN (from experiment 1) and ENZYME FEED (from experiment 2) were treatments designed to be equivalent.
In order to have uniformity, the three aforementioned cow-calf groups were formed taking into consideration calf sex, age, and live body weight prior to starting the study. Once the three groups were formed, they remained separated for the entire trial, which lasted an average of 98 days each year. Each farm had a total of 6 paddocks which were randomly assigned to the experimental treatments, so each treatment was applied to 2 paddocks in each farm every year the study was replicated. Within each farm, the forage composition of the experimental paddocks was approximately the same. On the farm located in Eatonton, GA, the research paddocks were composed of a combination of bermudagrass (Cynodon dactylon) and dallisgrass (Paspalum dilatatum), whereas in Alapaha, GA, animals were grazing a combination of bermudagrass and bahiagrass (Paspalum notatum). In order to assess forage quality, one initial and one final forage sample were obtained from each individual paddock every year.
Both the calves and their respective dams had their performance evaluated three times during the study: at the beginning, middle, and end of the trial. On these evaluation days, body condition scores (BCS) were assessed for the cows, and live BW were assessed for both cows and calves. The creep feeds were offered to the supplemented groups three times per week, and the portion of the feed that was not consumed by calves was removed and recorded on a weekly basis.
2.2.2. Statistical analysis
Statistical analyses were carried out using the software Minitab® (version 18.1). Since there were significant treatment by farm interactions for some important traits, results from the analyses are presented for each farm individually. Analysis of variance was carried out using treatment, year, and sex (for the Eatonton, GA station) as fixed effects. The model also included the interaction terms for these effects. Additionally, since the accuracy of the model was significantly improved by its inclusion, calf initial age was included as a covariate. Orthogonal contrasts were tested using Tukey’s honest significant difference test, and means were considered different at P ≤ 0.05.
3. Results
3.1. Experiment 1
3.1.1. Total production of gas and methane
Results from show that the total volume of gas produced per g of incubated DM after 24 h of fermentation was lowest (P < 0.001) for GRASS, and there were no differences (P > 0.05) in gas production among all the other treatments. In addition, across all treatments, total production of CH4 in 24 h was lowest (P = 0.002) when GRASS was used as substrate. Similarly, production of CH4 per g of incubated DM was the least among all treatments for GRASS (P < 0.001).
Table 2. Production of gas, methane, disappearance of fibre (NDF and ADF), and IVDMD after 24 h of incubation.
3.1.2. Digestibility of dry matter and fibre
As can be noticed, IVDMD was lowest (P = 0.01) for GRASS, and the only treatment that significantly improved this trait compared to GRASS was XYLAN, which increased it by 50.7% (27.4 versus 41.3%). Moreover, it can be noticed that the average percentage of IVDMD across all treatments was 33.9%, which is considerably lower than what is usually reported in the literature for batch culture incubations lasting 24 h. Regarding the fibre portion, differences were detected in the digestibility of both NDF and ADF across treatments (P ≤ 0.03; ). Moreover, both of these digestibilities were lowest for GRASS and highest for BGLUC.
3.1.3. Volatile fatty acids
shows a summary with regard to the VFA produced after 24 h of incubation. It can be noticed that total VFA concentration was lowest (P < 0.001) for GRASS, followed by CREEP, it was maximized in BGLUC, with XYLAN being intermediate. With respect to each individual VFA, GRASS yielded the greatest (P < 0.001) proportion of acetate, and the lowest (P < 0.001) proportions of butyrate and isovalerate. Furthermore, GRASS tended (P = 0.09) to yield lower proportions of propionate.
Table 3. Total VFA concentration, acetate:propionate ratio, and proportions of main VFA (mol/100 mol) after 24 h of incubation.
3.2. Experiment 2
3.2.1. Animal performance
Performance of calves for the 2 experimental stations over the 3 years is summarized in and . At both stations, calf initial age and initial BW were similar (P ≥ 0.54) across all treatment groups; however, final BW was significantly different (P = 0.04) at the station located in Alapaha, GA, but not at the one located in Eatonton, GA (P = 0.74). In Alapaha, GA, final BW was greatest for calves in ENZYME FEED, lowest for NO FEED, with PLAIN FEED being intermediate. Regarding calf growth rates, it can be noticed that average daily gain (ADG) during the first half of the trial was different (P ≤ 0.03) at both stations, with greater numerical values observed in the supplemented groups (i.e. PLAIN FEED and ENZYME FEED). Average daily gain during the second half of the trial was affected by treatment (P < 0.001) only in Alapaha, GA, but when comparing ADG for the entire trial, differences across treatments (P ≤ 0.03) were observed at both stations. Moreover, although ADG of calves in ENZYME FEED was greater than what was observed for NO FEED at both stations, no differences were detected (P > 0.05) between PLAIN FEED and ENZYME FEED. Regarding the cows, results from and show that except for ADG at the station located in Eatonton, GA, none of the treatments offered to calves significantly affected performance of their dams during the trial.
Table 4. Performance of calves at the first experimental station (Eatonton, GA) during the 3-year feeding trial.
Table 5. Performance of calves at the second experimental station (Alapaha, GA) during the 3-year feeding trial.
Table 6. Performance of cows at the first experimental station (Eatonton, GA) during the 3-year feeding trial.
Table 7. Performance of cows at the second experimental station (Alapaha, GA) during the 3-year feeding trial.
3.2.2. Intake of supplements and feed conversion
The daily DMI for the supplements used in our feeding trial and their conversion into BW gain are summarized in . At the station located in Alapaha, GA, supplement DMI was significantly lower (P = 0.01) in ENZYME FEED, compared to PLAIN FEED. At the station located in Eatonton, GA, only a trend (P = 0.08) for reduced DMI due to addition of xylanase into the creep feed was observed. Regarding the supplement conversion into BW gain, the presence of xylanase in the creep feed numerically improved feed conversion (supplement gain:feed ratio), however, this improvement was only statistically significant at one experimental station (Eatonton, GA; P = 0.01).
Figure 1. Supplement dry matter intake (DMI) and gain:feed ratio observed during the 3 years of the study for calves in PLAIN FEED and ENZYME FEED*. Bars with different letters on the top (a or b) within each plot are different (P ≤ 0.05). (A) Supplement DMI observed in Eatonton, GA (1.39 vs. 1.33 kg/day); (B) Supplement DMI observed in Alapaha, GA (1.26 vs. 1.01 kg/day); (C) Supplement Gain:Feed ratio observed in Eatonton, GA (0.031 vs. 0.062); (D) Supplement Gain:Feed ratio observed in Alapaha, GA (0.133 vs. 0.144). * PLAIN FEED = calves were creep fed. ENZYME FEED = calves were creep fed with an enhanced feed containing xylanase.
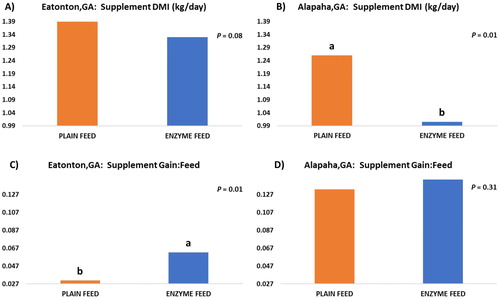
4. Discussion
4.1. Experiment 1
4.1.1. Total production of gas and methane
As previously mentioned, shows the total volume of gas produced in the 24-h fermentation. Gas measurement provides useful data on digestion kinetics of both soluble and insoluble fractions of feedstuffs, and for that reason, the gas measuring technique has been widely used in the evaluation of nutritive value of feeds (Getachew et al. Citation1998). In a recent study, Kholif et al. (Citation2017) evaluated the effects of different forage:concentrate ratios on in vitro total gas production using rumen fluid from a cannulated cow. Their results showed that increasing the concentrate portion of the diet linearly increased the volume of gas produced per g of incubated DM, which is in line with our findings: Except for GRASS, all of our treatments had concentrate in their composition, which resulted in greater production of gas after 24 h of incubation.
Giraldo et al. (Citation2008) studied the effects of fibrolytic enzymes on in vitro fermentation of substrates with different forage:concentrate ratios. Their results showed that, regardless of the inclusion of fibrolytic enzymes, the forage:concentrate ratio had a significant effect on the quantity of CH4 produced per g of incubated dry matter. Similarly, Kholif et al. (Citation2017) reported that the ratio of forage:concentrate in the diet affects the proportional production of CH4 per g of incubated DM in a quadratic manner for incubations lasting 24 h. Although the present study did not evaluate more than 2 levels of inclusion of concentrate in the treatments (i.e. the percentage of concentrate was actually 0% in GRASS, and 25% in all the other treatments), our results agree with the findings of these authors since they clearly show that the presence or absence of concentrate markedly affected production of CH4. With respect to the use of feed enzymes, our results did not show any differences in CH4 production, which is in harmony with the findings from Giraldo et al. (Citation2008). These authors tested the inclusion of xylanase from Trichoderma viride, and cellulase from Aspergillus niger, both at 2 doses: 40 and 80 enzymatic units per g of diet DM. Regardless of which enzyme or the dose of inclusion, they found no significant variations in production of CH4 after 24 h of incubation.
4.1.2. Digestibility of dry matter and fibre
As mentioned before, the percentage of IVDMD in the present study was relatively low compared to what was reported in other studies. For instance, a previous study from our group (Lourenco et al. Citation2016) used a similar in vitro technique and found IVDMD values ranging from 69.9 to –74.5%. Such substantial differences may be partially explained by the composition of the treatments used in both studies: while the present study used forage-based diets, that study used diets that resemble the ones typically used in feedlots (which have higher inclusion of concentrate feeds). However, the main factor behind the lower IVDMDs observed in the present study is probably the age of the rumen fluid donors. Whereas Lourenco et al. (Citation2016) used rumen fluid obtained from mature steers, the present study used fluid collected from a group of 186-day-old calves which were still in their suckling phase. Because calves in that stage of their lives are still experiencing transformations in their forestomach, and since their associated microbial population is not fully developed, it seems reasonable that the IVDMD values assessed using rumen fluid obtained from such young calves would be inferior than what is normally seen when the fluid is obtained from mature animals. Moreover, Segers et al. (Citation2013) performed in vitro evaluations of diets that had 75% roughage in their composition, which is the same percentage used in most of our treatments. Still, their IVDMD values were markedly higher than the ones observed in our study: they ranged from 70.5 to 74.9% for all their tested diets. Not surprisingly, the rumen fluid used in their study was obtained from adult ruminants: lactating dairy cows. In another in vitro study performed with treatments containing the same roughage:concentrate ratios that were used in our research, Ramírez et al. (Citation2015) found IVDMD values ranging from 52.5to 61.6% for treatments with a 75:25 roughage:concentrate ratio, and 42.2 to 52.4% for treatments composed of 100% roughage, incubated for 24 h. In accordance with the other authors previously cited, Ramírez et al. (Citation2015) also utilized rumen fluid from adult animals in their incubations, which in their case was obtained from 3 Holstein Friesian cows. Therefore, the relatively low IVDMD values observed in the present study are most likely due to the young age of our rumen fluid donors, which were only 186 ± 7 days-old when their fluid was collected.
Concerning the fibre portion of the treatments, our results diverge from the ones reported by Giraldo et al. (Citation2008), who evaluated the use of 2 cellulases and 1 xylanase on in vitro fermentations. When compared to their control treatment (i.e. no addition of feed enzyme), all the enzymes used in their research significantly increased ADF digestibility after 24 h of incubation. However, NDF digestibility at 24 h was only improved by the addition of xylanase obtained from Trichoderma viride, and by the cellulase from Trichoderma longibrachiatum. In another study, He et al. (Citation2014) used 4 adult cannulated heifers to evaluate the effects of xylanase and cellulase on in situ and in vivo digestion. Although they did not measure in situ ruminal disappearance of ADF, their data show that ruminal disappearance of the NDF fraction at 24 h was not affected by the addition of the fibrolytic enzymes. Likewise, they found no response of adding those enzymes on total tract digestibility of NDF or ADF, even when the dose of inclusion was doubled (i.e. from 16,150 to 32,300 units/kg DM for xylanase; and from 500 to 1000 units/kg DM for cellulase).
4.1.3. Volatile fatty acids
Because the in vitro technique used in the present study allows VFA to be produced but not absorbed as it happens in vivo (Carpenter et al. Citation2017), our results indicate that more fermentation – and consequently greater VFA production – occurred when creep feed was added to the pure bermudagrass. Moreover, they show that the rumen microorganisms were able to ferment even more of these compounds when the treatment BGLUC was used. An adequate development of the ruminal epithelial tissue of calves is critical since this tissue is responsible for absorption of VFA. Consumption of dry feeds and the resulting end products of microbial fermentation (such as VFA) stimulate the development of the rumen epithelium. Furthermore, no end product generated in the rumen stimulates this development more than butyrate (NRC, Citation2001; Yáñez-Ruiz et al. Citation2015). Since the in vitro production of butyrate was increased in all treatments in comparison to GRASS, it can be inferred that, if tested in live animals, these treatments would have caused greater ruminal epithelial tissue development in the animals consuming them, compared to animals fed exclusively forage.
4.1.4. Conclusions from Experiment 1
In summary, for most of the traits analysed in Experiment 1, there was a clear distinction between the results yielded by GRASS and all the other treatments. The lower IVDMD, digestibility of fibre, smaller production of total VFA, and lower proportion of butyrate observed for GRASS indicate that suckling beef calves receiving forage as their only source of dry feed may not have the same development as calves that are supplemented with creep feed. In addition, compared to the treatment containing a plain creep feed (CREEP), the addition of the 2 selected enzymes to creep feeds did not significantly improve most of the traits measured in this in vitro study. However, we may have failed to capture significant differences between CREEP and the enzyme-containing treatments (BGLUC and XYLAN) due to the limited number of replications in this study. But still, some important traits such as IVDMD and ADF digestibility were numerically improved by the inclusion of the enzymes, to a point that these traits became significantly different than GRASS.
4.2. Experiment 2
4.2.1. Animal performance
Our results regarding calf ADG during the whole trial are in harmony with the in vitro digestibilities assessed in Experiment 1. The IVDMD of pure bermudagrass in that first experiment (which would resemble NO FEED in Experiment 2) was the lowest among all treatments: 27.4%. In contrast, the treatment from Experiment 1 with greatest IVDMD (41.3%) was the one containing the enzyme xylanase, which is comparable to the ENZYME FEED treatment in Experiment 2. Therefore, just as XYLAN was the only treatment yielding IVDMD values significantly different from GRASS in Experiment 1, ENZYME FEED was the only treatment with significantly distinct overall ADG compared to NO FEED in Experiment 2. Thus, the values of IVDMD observed in Experiment 1 using analogous treatments were in fact closely related with the calf ADG observed during the whole trial in Experiment 2.
As shown in , supplement intake was reduced by the addition of xylanase to the creep feed, and this reduction in feed consumption likely helped the improved feed conversion observed for calves in ENZYME FEED, compared to PLAIN FEED. Although this improvement in supplement conversion into BW gain was only statistically significant in one of our stations (Eatonton, GA; P = 0.01), this improvement in efficiency was numerically consistent at both of our experimental stations. This indicates that the inclusion of xylanase into creep feeds can reduce feed intake and enhance creep feed conversion.
To our knowledge, there are no studies dealing with the inclusion of feed enzymes in beef cattle creep feeds (i.e. during their suckling phase). However, two studies (Beauchemin et al. Citation1995 and McAllister et al. Citation1999) that used fibrolytic enzymes in recently-weaned beef calves generated results that are somewhat comparable to the ones from the present study. McAllister et al. (Citation1999) conducted a feeding trial using recently-weaned steers and fed them a 60:40% roughage:concentrate diet with 3 different levels of inclusion of cellulase and xylanase for 120 days. Their results showed no differences in overall ADG due to enzyme, regardless of the dose of inclusion. In addition, no differences were detected on their overall feed efficiency. In another study using a combination of xylanase and cellulase, Beauchemin et al. (Citation1995) fed steers with incremental levels of those enzymes in different diets. One of their treatments was composed of grass hay plus concentrate, and steers fed this diet had greater ADG when the tested enzymes were included at their greatest dosage. In addition to increased gains, authors also observed increased digestible DMI and greater ADF digestibility at this dose. In the present study, the lack of differences between PLAIN FEED and ENZYME FEED for ADG and final BW indicates that our findings are more in line with the ones reported by McAllister et al. (Citation1999).
Concerning the performance of cows, given that the feeding trial began when calves were on average 133 days-old, the lack of effects on cows’ BW and BCS are not surprising. It is known that beef cows reach peak milk production well before they reach 100 days postpartum. In addition, the first 3 months postpartum are the typically the most demanding ones in cows’ productive cycle, since they are still recovering from calving while producing large quantities of milk (NRC, Citation2000). Consequently, regardless if cows gained or lost BW and BCS during that high-demand phase of their productive cycle, most of the effects related to those high biological demands had already past when this experiment started. Thus, since the cows already had their body conditions well established when this trial started (i.e. 133 days postpartum on average), the influence of supplementing or not their calves was minimal on them.
4.2.2. Conclusions from Experiment 2
In conclusion, although no changes were observed in their dams, calves in the PLAIN FEED group had greater ADG than calves in NO FEED in one of the two experimental stations. On the other hand, calves in ENZYME FEED experienced greater ADG compared to NO FEED at both experimental stations. In addition, although no differences were observed between PLAIN FEED and ENZYME FEED concerning final BW and ADG, calves in ENZYME FEED experienced a reduction in supplement intake, and an improved conversion of the supplement into BW gain. These findings indicate that the inclusion of xylanase into beef cattle creep feeds is a promising strategy and should be further investigated using different scenarios (e.g. different season of the year, or different forages). In addition, levels other than 13,800 fungal xylanase units/kg of dry matter of feed should be studied, to evaluate if calf response is further improved, and to verify if such potential improvement occurs in a predictable way (i.e. linear or quadratic).
5. Conclusion
Overall, results from the 2 experiments demonstrated positive results concerning the use of feed enzymes in beef cattle creep feeds. Important traits like in vitro dry matter digestibility and total production of ruminal volatile fatty acids were maximized by the inclusion of fibrolytic enzymes in the creep feed. When tested on-farm, the enrichment of the creep feed with xylanase (ENZYME FEED) yielded greater calf ADG in both of our experimental stations, compared to the control group (NO FEED). However, the creep feed that did not have the inclusion of xylanase (PLAIN FEED) only promoted greater ADG in one of our experimental stations, compared to NO FEED. Furthermore, adding xylanase into the creep feed decreased the daily intake of supplement and improved the conversion of creep feed into body weight gain. Therefore, taken together, these results provide evidence that encourage the addition of fibrolytic enzymes (particularly xylanase) into beef cattle creep feeds.
Acknowledgments
The authors would like to thank the Georgia Agricultural Commodity Commission for Beef for providing financial support for this research. In addition, we would like to thank DSM Nutritional Products for providing financial support and the enzymes used in this research.
Disclosure statement
No potential conflict of interest was reported by the author(s).
ORCID
J. M. Lourenco http://orcid.org/0000-0003-1479-0528
References
- Balci F, Dikmen S, Gencoglu H, Orman A, Turkmen II, Birick H. 2007. The effect of fibrolytic exogenous enzyme on fattening performance of steers. Bulg. J Vet Med. 10:113–118.
- Beauchemin KA, Colombatto D, Morgavi DP, Yang WZ. 2003. Use of exogenous fibrolytic enzymes to improve feed utilization by ruminants. J Anim Sci. 81:E37–E47. doi:10.2527/2003.8114_suppl_2E37x doi: 10.2527/2003.8161628x
- Beauchemin KA, Rode LM, Sewalt VJH. 1995. Fibrolytic enzymes increase fiber digestibility and growth rate of steers fed dry forages. Can J Anim Sci. 75:641–644. doi:10.4141/cjas95-096.
- Carpenter AJ, Binversie E, Ruiz-Moreno M, Stern MD. 2017. Effect of dried fermentation biomass on microbial fermentation in continuous culture and in vitro intestinal digestibility. Anim Feed Sci Technol. 230:47–58. doi:10.1016/j.anifeedsci.2017.05.020.
- Church DC. 1979. Growth and development of the ruminant stomach. digestive physiology and nutrition of ruminants. Volume 1: digestive Physiology, 2nd ed. Portland, OR: Oxford Press Inc.
- Getachew G, Blümmel M, Makkar HPS, Becker K. 1998. In vitro gas measuring techniques for assessment of nutritional quality of feeds: a review. Anim Feed Sci Technol. 72:261–281. doi:10.1016/S0377-8401(97)00189-2.
- Giraldo LA, Tejido ML, Ranilla MJ, Carro MD. 2008. Effects of exogenous fibrolytic enzymes on in vitro ruminal fermentation of substrates with different forage: concentrate ratios. Anim Feed Sci Technol. 141:306–325. doi:10.1016/j.anifeedsci.2007.06.013.
- He ZX, He ML, Walker ND, McAllister TA, Yang WZ. 2014. Using a fibrolytic enzyme in barley-based diets containing wheat dried distillers grains with solubles: ruminal fermentation, digestibility, and growth performance of feedlot steers. J Anim Sci. 92:3978–3987. doi:10.2527/jas.2014-7707.
- Kholif AE, Elghandour MMY, Salem AZM, Barbabosa A, Márquez O, Odongo NE. 2017. The effects of three total mixed rations with different concentrate to maize silage ratios and different levels of microalgae Chlorella vulgaris on in vitro total gas, methane and carbon dioxide production. J Agric Sci. 155:494–507. doi:10.1017/S0021859616000812.
- Lourenco JM, DiLorenzo N, Stelzleni AM, Segers JR, Stewart Jr RL. 2016. Use of by-product feeds to decrease feed cost while maintaining performance of developing beef bulls. Prof. Anim Sci. 32:287–294. doi:10.15232/pas.2015-01436.
- Lourenço JM, Froetschel MA, Segers JR, Tucker JJ, Stewart Jr RL. 2017. Utilization of canola and sunflower meals as replacements for soybean meal in a corn silage-based stocker system. Transl Anim Sci. 1:592–598. doi:10.2527/tas2017.0068.
- McAllister TA, Oosting SJ, Popp JD, Mir Z, Yanke LJ, Hristov AN, Treacher RJ, Cheng KJ. 1999. Effect of exogenous enzymes on digestibility of barley silage and growth performance of feedlot cattle. Can J Anim Sci. 79:353–360. doi:10.4141/A98-099.
- McDougall EI. 1948. Studies on ruminant saliva. 1. The composition and output of sheep's saliva. Biochem J. 43:99–109. doi: 10.1042/bj0430099
- Meale SJ, Beauchemin KA, Hristov AN, Chaves AV, McAllister TA. 2014. Board-Invited Review: opportunities and challenges in using exogenous enzymes to improve ruminant production. J Anim Sci. 92:427–442. doi:10.2527/jas.2013-6869.
- Moriel P, Arthington JD. 2013. Effects of trace mineral-fortified, limit-fed preweaning supplements on performance of pre- and postweaned beef calves. J Anim Sci. 91:1371–1380. doi:10.2527/jas.2012-5469.
- NRC. 2000. Nutrient requirements of beef cattle. 7th rev. ed. Natl. Washington, DC: Acad. Press.
- NRC. 2001. Nutrient requirements of dairy cattle. 7th rev. ed. Natl. Washington, DC: Acad. Press.
- Prichard DL, Hargrove DD, Olson TA, Marshall TT. 1989. Effects of creep feeding, zeranol implants and breed type on beef production: I. calf and cow performance. J Anim Sci. 67:609–616. doi:10.2527/jas1989.673609x.
- Ramírez J, Posada O, Noguera R. 2015. Effects of Kikuyu grass (Pennisetum clandestinum) age and different forage: concentrate ratios on methanogenesis. Rev MVZ Cordoba. 20:4726–4738. doi: 10.21897/rmvz.43
- Segers JR, Stelzleni AM, Pringle TD, Froetschel MA, Ross CL, Stewart Jr RL. 2013. Use of corn gluten feed and dried distillers grains plus solubles as a replacement for soybean meal and corn for supplementation in a corn silage-based stocker system. J Anim Sci. 91:950–956. doi:10.2527/jas.2011-4392.
- Tarr SL, Faulkner DB, Buskirk DD, Ireland FA, Parrett DF, Berger LL. 1994. The value of creep feeding during the last 84, 56, or 28 days prior to weaning on growth performance of nursing calves grazing endophyte-infected tall fescue. J Anim Sci. 72:1084–1094. doi:10.2527/1994.7251084x.
- Viñoles C, Jaurena M, De Barbieri I, Do Carmo M, Montossi F. 2013. Effect of creep feeding and stocking rate on the productivity of beef cattle grazing grasslands. N Z J Agr Res. 56:279–287. doi:10.1080/00288233.2013.840320.
- Yáñez-Ruiz DR, Abecia L, Newbold CJ. 2015. Manipulating rumen microbiome and fermentation through interventions during early life: a review. Front Microbiol. 6:1133. doi:10.3389/fmicb.2015.01133.