ABSTRACT
Intestinal fatty-acid binding protein (I-FABP, also called FABP2) is involved in the uptake of dietary fatty acids in the intestine, and its binding specificity is only with long-chain fatty acid. In this study, sequences of 4 exons in pigeon FABP2 were successfully amplified. The encoded protein had a molecular weight of 15.13 kDa and the theoretical pI was 6.75. Bioinformatics analysis indicated that the protein was predicted to contain 10 potential phosphorylation sites and did not contain signal peptide structure. The evolutionary analysis suggested the amino acid sequence of pigeon FABP2 was closest to the mallard (Nippoia nippon) homologs. Meanwhile the expression of FABP2 mRNA in different ages and tissues we conducted. It showed that the expression of the FABP2 gene was the highest in the liver, followed by the small intestine and adipose tissue, while lowest in the breast muscle. Also, the expression level in the gizzard was gradually increased with aging. Our results may serve as reference to better studies of the functional characterization and structure of the FABP2 in pigeon.
Introduction
The main function of fatty acid binding proteins (FABPs) is to transport fatty acids with a high affinity for hydrophobic ligands, such as long-chain fatty acids (LCFAs) (Veerkamp and Maatman Citation1995). To date, 12 FABP isoforms have been identified. FABP members were named according to the tissue in which they were discovered or prominently expressed (Glatz and van der Vusse Citation1990). They are mainly expressed in various tissues in mammals and have tissue expression specificity.
Intestinal fatty acid binding protein (FABP2) was first isolated from the cytosol of the intestinal mucosa (Ockner Citation1991) and synthesized in polar columnar epithelial cells (Scapin et al. Citation1992). FABP2 is involved in the uptake of dietary fatty acids in the intestine, and it only binds long-chain fatty acids with a ratio of one fatty acid for one protein (Besnard et al. Citation2002). Agellon et al. (Citation2007) reported that the loss of FABP2 rendered male mice susceptible to high-fat diet-induced fatty liver. In recent years, research on the FABP2 gene has mainly focused on polymorphism, especially in human diseases such as obesity, lipid metabolism, and diabetes (Albala et al. Citation2007; Tavridou et al. Citation2009) among others. Meanwhile, the systematic circulation content of FABP2 could be used as a marker for human intestinal diseases, such as intestinal injury (Liu et al. Citation2016; Voth et al. Citation2017), necrotizing enterocolitis (Schurink et al. Citation2015) and ileitis in patients (Wiercinska-Drapalo et al. Citation2008). FABP2 protein was thought to be restricted to the intestine and stomach of mammals (Veerkamp and Maatman Citation1995) but was found in a variety of tissues that participated in adipose metabolism in some animals. In our previous study, the full-length cDNA of FABP2 gene had been cloned from the intestine of domestic pigeons. The expression of FABP2 gene showed a distinct development pattern in the duodenum and ileum, which could be an indicator of intestinal development in pigeons (Xie et al. Citation2013). The FABP2 gene has been studied mainly in mammals, but its physiological function remains to be clearly defined. Thus far, information about avian FABP2 is very scarce. In order to reveal the function of FABP2, we detected FABP2 gene polymorphisms in pigeons. Subsequent systemic analysis of proteins encoded by the FABP2 gene and the expression profile in various tissues and the time distribution was presented to provide information for future functional study of this gene.
Materials and methods
Animals
Two hundred healthy domestic pigeons obtained from Xingliang commercial pigeon farm (Wenzhou, China) were used for SNP identification in the FABP2 gene. Blood samples were collected from pigeons at 4 weeks of age. The selected experimental pigeons were hatched on the same day and fed by their parents, which were housed one pair (male-female paired) per cage under the same managerial conditions. The samples of various tissues at different periods of age, including zero weeks, one week, two weeks, three weeks, and four weeks, were dissected, temporarily frozen in liquid nitrogen and then stored at −80°C. The experiment was conducted in accordance with the Chinese guidelines for animal welfare and was approved by the animal welfare committee of the Animal Science College, Zhejiang University.
DNA extraction, primer design, PCR amplification, and sequencing
Blood samples were collected and then stored at −20°C using a Genome DNA Extraction Kit (TIANGEN, Beijing, China) to isolate genomic DNA according to the manufacturer's instructions. The primer pairs used to amplify the exon regions of the FABP2 gene were designed using Primer Premier 5.0 software (Premier Biosoft International, Palo Alto, CA) from the reference sequences of the pigeon FABP2 gene in GenBank (accession number: NW-004973640.1). Primers were synthesized by TSINGKE Biotech Co., Ltd (Hang Zhou, China), and the sequences are shown in . PCR amplification was performed in a total reaction volume of 50 µL containing 25 μL of 2× Taq PCR Master Mix (including dNTP, Mg2+, and Taq DNA Polymerase), 2 µL of genomic DNA, 2 µL of each primer, and Milli-Q water. The cycling protocol was 3 min at 94°C, 30 cycles of 94°C for 30 s, 1 min at the annealing temperature (), extension at 72°C for 1 min, with a final extension at 72°C for 5 min. The PCR products were verified by 1.5% agarose gel electrophoresis and sequenced by Hangzhou Qingkezixi Biotech Co., Ltd (Hangzhou, China). To identify SNPs, the sequences were analyzed with DNAman software (DNAMAN Application 4.0.1.1, Lynnon BioSoft, USA).
Table 1. Primers sequences of PCR amplification of pigeon FABP2a gene.
Analysis of protein sequences
FABP2 protein sequences of pigeon and other species were obtained from the NCBI database ().
Table 2. Amino acid sequences encoded by different species of FABP2 genes.
Bioinformatics analysis of the FABP2 gene homologue
FABP2 amino acid sequence analysis and structure prediction were performed using the following bioinformatics software tools. The ORF Finder programme was used to analyse the ORF (open reading frame) of the sequence and predicted the amino acid sequence. The physicochemical properties of the protein were analyzed using the software ProtParam from ExPASy. The prediction of the protein phosphorylation sites of FABP2 was identified using the NetPhos 3.1 server. To analyse the subcellular localization of the protein, PSORT II Prediction was employed. The signal peptide cleavage sites in the amino sequence of FABP2 were analyzed by SignalP 3.0 Server, and the transmembrane helix was predicted with TMpred. The secondary structure of proteins used network software to make predictions. Motif Scan software was utilized to analyse the functional sites of the amino acid sequence. The tertiary structure of the protein was homologously modelled using the online software SWISS-MODEL. The amino acid sequence alignment was performed using ClustalW, and the phylogenetic tree was constructed with the programme MEGA 6.0.
RNA isolation and cDNA synthesis
RNA was extracted from the heart, liver, spleen, lung, kidney, breast muscle, gizzard, glandular stomach, intestine, and adipose tissue using TRIzol reagent (Invitrogen, Carlsbad, CA, USA) following the manufacturer's procedure. The total RNA concentration and integrity of samples was estimated by both RNA electrophoresis on 1.5% agarose gel and the OD absorbance ratio at 260 and 280 nm. The total RNA was reverse transcribed (TaKaRa) to produce cDNA at 42°C for 15 and 3 min at 95°C in reaction volume of 20 µL which including 4 µL 5x FastKing-RT SuperMix, 1 µL Total RNA, 15 µL RNase-Free ddH2O.
Analysis of the expression profile of FABP2
Semi-quantitative RT–PCR was used to quantify the expression of FABP2 in the various tissues.
According to the mRNA sequence of pigeon FABP2 (NM_001282808.1) gene, Primer Premier 5.0 software was used to design primers qPCR (). The relative mRNA abundance of the FABP2 gene in the various tissues of pigeons was normalized to the β-actin gene using the 2−ΔΔCt method. The RT–PCR was performed in a total reaction volume of 20 µL which containing 10 µL SYBR® Premix Ex TaqTMII(2×), 0.8 µL PCR Forward Primer (10 µM), 0.8 µL PCR Reverse Primer (10 µM), 0.4 µL ROX Reference Dye (50×), 2.0 µL genomic DNA, and 6.0 µL dH2O. The cycling protocol was 30 s at 95°C, 40 cycles of 95°C for 5 s, 30 s at 60°C.
Table 3. Primers sequences of Real-time PCR.
Results
Analysis of FABP2 mutation sites
Analysis of the sequencing results revealed that no base mutation was found in the exons of the FABP2 gene in pigeons.
Analysis of the amino acid sequence of FABP2
The gene and amino acid sequences of pigeon FABP2 were obtained from NCBI for further analysis. Analysis of the FABP2 amino acid sequence indicated that the ORF encodes a putative protein of 132 amino acids. The molecular formula of the protein is C671H1051N1850204S5. The analysis product encoded by the FABP2 gene showed that Gly and Lys had the highest frequency (9.8%), while Ser had the lowest frequency (0.8%). Analysis of the amino acid sequence indicated a predicted molecular weight of 15.13 kDa and a calculated isoelectric point of 6.75. The estimated half-life was 30 hours (mammalian reticulocytes, in vitro). The instability index (II) was computed to be 13.12, so the protein was classified as stable. The aliphatic index was 70.15. The grand average hydropathicity (GRAVY) was −0.645. Analysis of the hydropathicity of FABP2 revealed MIN −2.711 and MAX 1.300.
The NetPhos 3.1 server was used to predict the phosphorylation sites in pigeon FABP2 protein and identified 10 potential phosphorylation sites, including 2 serine, 7 threonine and 1 tyrosine. The subcellular localization of the protein was highest in the cytoplasm (69.6%), followed by the nucleus (26.1%) and peroxisome (4.3%). The SignalP-mm and SignalP-hmm results both showed that the protein did not contain a signal peptide structure and was a non-secretory protein. The TMpred results suggested that there was probably no transmembrane protein and no possible model found.
Evolutionary analysis of the amino acid sequences of FABP2 homologues
To analyse the evolutionary relationship between the FABP2 sequences from pigeon and other species, the ClustalW programme was used to align the FABP2 amino acid sequences from eighteen species (). The homologies between the pigeon and other species were as high as 62.88%–90.84% at the protein level. The phylogenetic tree was constructed using the MEGA 6.0 programme (). Analysis of evolutionary results showed that it was divided into three categories in the evolution process. Among them, pigeons have the closest relationship with birds, which is consistent with evolution, followed by fish, and the farthest relationship with mammals and ruminants. The results suggested that the amino acid sequence of pigeon FABP2 is the closest to the mallard homologues in terms of genetic data. For example, the pigeon FABP2 sequence exhibited 90.84% homology with mallard FABP2, whereas the homology to the crested ibis, turkey, zebrafish, pig, human, and sheep FABP2 sequences was 90.15%, 87.12%, 74.24, 77.27%, 71.21% and 75.00%, respectively ().
Figure 1. Multiple sequence alignment of FABP2 amino acid sequences in the eighteen species. ‘*’ represents identical aa, ‘:’, and ‘.’ are highly and relative highly conserved aa.
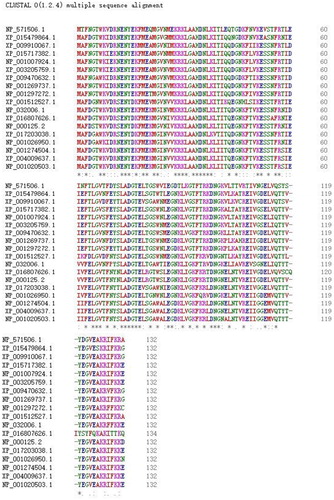
Structural modelling of pigeon FABP2
Motif Scan was used to predict the functional loci of the pigeon FABP2 protein. The Pfam programme results showed that a cytosolic fatty acid binding protein signature was identified from residues 5–22, and a lipocalin domain was found located at 4–132 amino acids.
Predict Protein software was used to predict the secondary structure of the protein encoded by the pigeon FABP2 gene. The results showed that H (alpha-helix), E (beta-sheet), and L (random coil) were 12.12%, 59.09%, and 28.79%, respectively.
The SWISS-MODEL online tool was used to predict the tertiary structure of proteins, and 50 better templates with sequence consistency above 30% were found. Using rat FABP2 as a template, the SWISS-MODEL programme built a tertiary structure of pigeon FABP2 protein (). Beta-sheet accounted for the main proportion of tertiary structure, followed by alpha-helix and random coil, which was consistent with the prediction of the secondary structure of the protein.
Analysis of differential expression in various tissues and different ages of pigeon
The real-time PCR results showed that the FABP2 gene was expressed in different ages and tissues (). During the period from 0 to 3 weeks, the expression of the FABP2 gene was highest in the liver, followed by the small intestine and adipose tissue, while the expression was lowest in the breast muscle. The expression was low in the spleen and gizzard at 0 weeks, but it increased significantly during the period of weeks 1–4. However, the expression in the heart was also low. The interesting thing about the lung expression was that it was only obvious during the first week and was still very low during the rest of the weeks, while there was a similar pattern of expression in the kidney, but it was highest in the second week. In adipose tissue, the expression of FABP2 increased with ageing. At 4 weeks of age, the expression of the FABP2 gene was highest in the lipid, followed by the spleen, liver and small intestine, while the expression was lowest in the breast muscle.
Discussion
Intestinal fatty acid binding protein (FABP2) is a member of the fatty acid binding protein family and is specifically expressed in intestinal epithelial cells (Weiss et al. Citation2002). The distribution of FABP2 corresponds to the anatomical location of dietary fat absorption and esterification. In intestinal epithelial cells, FABP2 binds to the long-chain fatty acids and transfers them to the endoplasmic reticulum, where they are esterified into triglycerides, which are secreted into the humoral circulation as chylomicrons.
Currently, studies on FABP2 polymorphisms mainly focus on obesity, diabetes and other human diseases. There have been relatively few reports about livestock and poultry. The pigeon FABP2 exon sequences were amplified, and it was confirmed that they were FABP2 homologous sequences by BLAST analysis. SNP sites were detected in four exons of the FABP2 gene by direct sequencing. Sequence alignment revealed that no polymorphic sites were found in the exons of the FABP2 gene. It could be inferred that the FABP2 gene sequence might be conserved in the pigeon. Therefore, we further analyzed the protein encoded by the pigeon FABP2 gene to determine this speculation.
Analysis of the predicted FABP2 amino acid sequence indicated that the open reading frame (ORF) encodes a putative protein of 132 amino acids. The calculated molecular weight of the protein is 15.13 kDa, and the theoretical pI is 6.75, which is consistent with the deduced size of FABP2 protein in other species (Jiang and Li Citation2006; Wang et al. Citation2015). The FABP2 protein does not contain a signal peptide structure and has 10 potential phosphorylation sites. The predictive analysis showed that FABP2 did not have a transmembrane helix, which was consistent with the prediction of FABP2 as a cytosolic protein. Moreover, this was in accordance with the subcellular localization of FABP2 protein. FABP was mainly expressed in the cytoplasm. This might be related to its involvement in intracellular lipid metabolism and fatty acid transport (Schaap et al. Citation2002). The tertiary structure of a protein is closely related to its biological function. The crystal structure of FABP2 in rats has been used as a standard reference to understand the tertiary structure of homologous proteins. FABPs have highly similar tertiary structures consisting of a 10-stranded antiparallel β-barrel and an N-terminal helix-turn-helix motif (Storch and Thumser Citation2010). The pigeon FABP2 protein tertiary structure consists of 10 antiparallel beta sheets, twisted and folded into a hydrophobic pocket. The structure of the model protein overlapped with the FABP2 protein from rats, suggesting that the gene family is basically conserved during evolution (Marcelino et al. Citation2006).
Furthermore, the alignment of the pigeon FABP2 sequence with 18 different species indicated that the encoded protein has very high homology with the amino acid sequence of FABP2 from the other 18 species (above 62.88%). The greatest homology of FABP2 amino acid sequences was observed between pigeons and mallards. The results further indicated that FABP2 in pigeons showed high conservation during evolution. Its encoded protein contained a conserved lipocalin domain, which was a transportation structure for typical hydrophobic small molecules (Dartt Citation2011). This was in agreement with the function of the FABP2 protein, which participates in the transport of intracellular fatty acids (Storch and Thumser Citation2000). In mammals, fatty acids are not only a nutrient in the body but are also regulators of animal metabolism. Fatty acids are highly hydrophobic, and they rarely exist in body fluids in free form. However, the fatty acids in cells can bind with FABP protein, making this hydrophobic molecule soluble in body fluids to ensure that the long-chain fatty acids are transported to the organelle and participate in intracellular adipose metabolism.
In this study, we analyzed the FABP2 expression profile in various tissues. We found that FABP2 was expressed in all selected tissues of pigeons. The analysis demonstrated that the highest levels of FABP2 mRNA were found in the liver, followed by the small intestine during 0–3 weeks post-hatching. At 4 weeks of age, the expression of FABP2 was highest in the adipose tissue, followed by the small intestine, spleen and liver. Liver, adipose tissue and small intestine are important sites for fatty acid metabolism. The high abundance of FABP2 in these tissues might be related to the high affinity of fatty acid binding protein to long-chain fatty acids. It is widely known that fats cannot dissolve in water and must be emulsified to be digested. Adipose was mainly converted into glycerol and fatty acids in the upper part of the small intestine by digestion with various enzymes and biliary saline solution (Jorgensen et al. Citation2001). Medium- and short-chain fatty acids could be absorbed directly by the lining of the small intestine, while long-chain fatty acids need to combine with apolipoprotein and cholesterol to form chylomicrons and enter the humoral circulation (Krogdahl Citation1985). Additionally, it was remarkable that the mRNA expression level of FABP2 in adipose tissue increased with age. This might be related to the gradual increase in fat deposition as pigeon age.
Jiang and Li (Citation2006) found that FABP2 was extensively expressed in various tissues but was more abundant in the intestine than other tissues. Similar findings were also obtained by Zhang et al. (Citation2019), who found that FABP2 was widely expressed in tissues and higher in the intestine, muscle, and brain than other tissues in loach. These results are consistent with the findings of the present study. However, Wang et al. (Citation2005) found that the FABP2 gene was expressed only in intestinal tissues in chickens. The same expression profile was observed in goat (Wang et al. Citation2015). Moreover, Chen et al. (Citation2012) found that FABP2 was mainly expressed in the intestine and the brain from the common carp Cyprinus carpio but was hardly expressed in the liver, heart, pancreas and muscle. This might reflect different expression locations in different species.
In conclusion, the FABP2 protein had a detectable lipocalin domain, and cytosolic fatty acid binding protein signatures were detected on the amino acid sequence. The pigeon FABP2 protein also had a very high homology with other species. The expression of the FABP2 gene showed a distinct pattern, with the majority in the liver, small intestine and lipid tissues in pigeons. The specific regulatory mechanism of FABP2 in vivo and its physiological and biochemical effects on adipose metabolism in animals remain to be further studied.
Disclosure statement
No potential conflict of interest was reported by the author(s).
Additional information
Funding
References
- Agellon LB, Drozdowski L, Li L, Iordache C, Luong L, Clandinin MT, Uwiera RRE, Toth MJ, Thomson ABR. 2007. Loss of intestinal fatty acid binding protein increases the susceptibility of male mice to high fat diet-induced fatty liver. Biochim Biophys Acta-Mol Cell Biol Lipids. 1771:1283–1288. doi: 10.1016/j.bbalip.2007.08.004
- Albala C, Villarroel A, Santos JL, Angel B, Lera L, Liberman C, Sanchez H, Pérez-Bravo F. 2007. FABP2 Ala54Thr polymorphism and diabetes in Chilean elders. Diabetes Res Clin Pract. 77:245–250. doi: 10.1016/j.diabres.2006.12.006
- Besnard P, Niot I, Poirier H, Clément L, Bernard A. 2002. New insights into the fatty acid-binding protein (FABP) family in the small intestine. Mol Cell Biochem. 239:139–147. doi: 10.1023/A:1020505512364
- Chen XW, Jiang S, Shi ZY. 2012. Identification and expression analysis of fabp2 gene from common carp Cyprinus carpio. J Fish Biol. 80:679–691. doi: 10.1111/j.1095-8649.2011.03203.x
- Dartt DA. 2011. Tear lipocalin: structure and function. Ocular Surface. 9:126–138. doi: 10.1016/S1542-0124(11)70022-2
- Glatz JFC, van der Vusse GJ. 1990. Nomenclature of fatty acid-binding proteins. Mol Cell Biochem. 98:231–235. doi: 10.1007/BF00231389
- Jiang YZ, Li XW. 2006. Molecular cloning and tissue-specific expression of intestinal-type fatty acid binding protein in porcine. Yi Chuan Xue Bao. 33:125–132.
- Jorgensen JR, Fitch MD, Mortensen PB, Fleming SE. 2001. In vivo absorption of medium-chain fatty acids by the rat colon exceeds that of short-chain fatty acids. Gastroenterology. 120:1152–1161. doi: 10.1053/gast.2001.23259
- Krogdahl A. 1985. Digestion and absorption of lipids in poultry. J Nutr. 115:675–685. doi: 10.1093/jn/115.5.675
- Liu Y, Jiang LF, Zhang RP, Zhang WT. 2016. Clinical significance of FABP2 expression in newborns with necrotizing enterocolitis. World J Pediatr. 12:159–165. doi: 10.1007/s12519-015-0035-1
- Marcelino AMC, Smock RG, Gierasch LM. 2006. Evolutionary coupling of structural and functional sequence information in the intracellular lipid-binding protein family. Proteins: Struct, Funct, Bioinf. 63:373–384. doi: 10.1002/prot.20860
- Ockner RK. 1991. Fatty-acid binding-proteins – considerable progress – more ground to cover – a citation-classic commentary on a binding-protein for fatty-acids in cytosol of intestinal-mucosa, liver, myocardium, and other tissues by Ockner, R.K., Manning, J.A., Poppenhausen, R.B., and Ho, W.K.L. Current Contents/Life Sci. Oct 7:9–9.
- Scapin G, Gordon JI, Sacchettini JC. 1992. Refinement of the structure of recombinant rat intestinal fatty acid-binding apoprotein at 1.2-a resolution. J Biol Chem. 267:4253–4269.
- Schaap FG, van der Vusse GJ, Glatz JFC. 2002. Evolution of the family of intracellular lipid binding proteins in vertebrates. Mol Cell Biochem. 239:69–77. doi: 10.1023/A:1020519011939
- Schurink M, Kooi EMW, Hulzebos CV, Kox RG, Groen H, Heineman E, Bos AF, Hulscher JBF, Chakravortty D. 2015. Intestinal fatty acid-binding protein as a diagnostic marker for complicated and uncomplicated necrotizing enterocolitis: a prospective Cohort study. Plos One. 10:e0121336. doi: 10.1371/journal.pone.0121336
- Storch J, Thumser AEA. 2000. The fatty acid transport function of fatty acid-binding proteins. Biochim Biophys Acta-Mol Cell Biol Lipids. 1486:28–44. doi: 10.1016/S1388-1981(00)00046-9
- Storch J, Thumser AE. 2010. Tissue-specific functions in the fatty acid-binding protein family. J Biol Chem. 285:32679–32683. doi: 10.1074/jbc.R110.135210
- Tavridou A, Arvanitidis KI, Tiptiri-Kourpeti A, Petridis I, Ragia G, Kyroglou S, Christakidis D, Manolopoulos VG. 2009. Thr54 allele of fatty-acid binding protein 2 gene is associated with obesity but not type 2 diabetes mellitus in a Caucasian population. Diabetes Res Clin Pract. 84:132–137. doi: 10.1016/j.diabres.2009.02.022
- Veerkamp JH, Maatman RGHJ. 1995. Cytoplasmic fatty acid-binding proteins: their structure and genes. Prog Lipid Res. 34:17–52. doi: 10.1016/0163-7827(94)00005-7
- Voth M, Duchene M, Auner B, Lustenberger T, Relja B, Marzi I. 2017. I-FABP is a novel marker for the detection of intestinal injury in severely injured trauma patients. World J Surg. 41:3120–3127. doi: 10.1007/s00268-017-4124-2
- Wang LJ, Li L, Jiang J, Wang Y, Zhong T, Chen Y, Wang Y, Zhang H. 2015. Molecular characterization and different expression patterns of the FABP gene family during goat skeletal muscle development. Mol Biol Rep. 42:201–207. doi: 10.1007/s11033-014-3759-4
- Wang QG, Li H, Liu SA, Wang GH, Wang YX. 2005. Cloning and tissue expression of chicken heart fatty acid-binding protein and intestine fatty acid-binding protein genes. Anim Biotechnol. 16:191–201. doi: 10.1080/10495390500276882
- Weiss EP, Brown MD, Shuldiner AR, Hagberg JM. 2002. Fatty acid binding protein-2 gene variants and insulin resistance: gene and gene-environment interaction effects. Physiol Genomics. 10:145–157. doi: 10.1152/physiolgenomics.00070.2001
- Wiercinska-Drapalo A, Jaroszewicz J, Siwak E, Pogorzelska J, Prokopowicz D. 2008. Intestinal fatty acid binding protein (I-FABP) as a possible biomarker of ileitis in patients with ulcerative colitis. Regul Pept. 147:25–28. doi: 10.1016/j.regpep.2007.12.002
- Xie P, Liu LL, Wang C, Zou XT. 2013. Molecular cloning, characterization, and mRNA expression of intestinal fatty acid binding protein (I-FABP) in Columba livia. Journal of Poultry Science. 50:9–19. doi: 10.2141/jpsa.0110125
- Zhang Y, Cao X, Gao J. 2019. Cloning of fatty acid-binding protein 2 (fabp2) in loach (Misgurnus anguillicaudatus) and its expression in response to dietary oxidized fish oil. Comp Biochem Physiol B Biochem Mol Biol. 229:26–33. doi: 10.1016/j.cbpb.2018.12.006