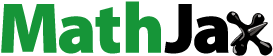
ABSTRACT
MUC1 is a glycoprotein mucin expressed in apical cells of luminal tissues, such as mammary gland luminal cells, which the main function is protect the cell surface from the environment microorganisms. The aim of this study were to identify the polymorphism of MUC1 gene in Murrah breed and to analyze the association between molecular marker and somatic cell counting, milk yield, fat yield, fat percentage, protein yield, protein percentage, age at first calving and calving interval. A total of 179 primiparous Murrah buffaloes were genotyped by PCR technique. Milk-recording were performed periodically to determine fat and protein content and somatic cell count. Three alleles of different lengths were amplified and named allele 1, 2 and 3, respectively. The allele 2 was more frequent followed by allele 3. The MUC1 gene was highly associated with traits related to mastitis resistance and milk percentage. Also was demonstrated a mild association between MUC1 gene and milk protein synthesis and a moderate effect on reproductive trait. The MUC1 gene is polymorphic in water buffaloes of Murrah breed and might be a potential marker to marker-assisted selection for intramammary infection and some milk production traits.
Introduction
Regarding to milk production and composition, mastitis is the most common disease in dairy cattle worldwide. Mastitis is an inflammatory response of the mammary gland to infection, almost always caused by infecting microorganisms with detrimental consequences to animal well-being and milk production. Therefore, increasing breeding programmes with the inclusion of genetic parameters to improve the resistance to mastitis in order to prevent infection might be a useful tool for the dairy industry (Meredith et al. Citation2013). Furthermore, fertility traits are also important to consider in dairy animals. Studies have shown that selection for standard alone higher milk yield producers is correlated with reduced fertility (Lucy Citation2001; Raden, Citation2004). Low fertility rate in buffaloes has been a major concern (Kumar et al. Citation2014) which could be partly explained due to the late maturity, prolonged intercalving period and low conception rate (Perera Citation2011). Hence, enhancing both productive and reproductive potential of buffaloes will lead to a better animal performance.
Among the candidates genes directly or indirectly associated with resistance to mastitis, milk production and fertility traits, there is the mucin 1 gene (MUC1). MUC1 is a glycoprotein mucin expressed in apical luminal epithelial tissues, such as mammary gland epithelial cells and reproductive tract cells, which the main function is protect the cell surface from the environment microorganisms, as well as it can play a role in epithelial differentiation and adhesion (Gendler Citation2001).
The MUC1 gene is well defined in several species (i.e. bovine), which has been associated with a highly polymorphic variable number of tandem repeats region in exon 2 (MUC1 VNTR), consisting in a 60 base-pairs (bp) sequence, that encodes 20 amino acids found in the extracellular domain. This region serve as sites for the O-glycosylation responsible for either adhesive or anti-adhesive role of the MUC1 in the cells (Rasero et al. Citation2002; Souza et al. Citation2007).
The aim of this study was to identify the VNTR polymorphism of MUC1 gene in water buffaloes of Murrah breed and to analyze the association between molecular marker and somatic cell counting, related with mastitis, milk production and composition traits, as well as with traits related to fertility.
Materials and methods
Animals and traits
A total of 179 primiparous Murrah buffaloes (age 2–4 year old) from a commercial herd located in Taipu-RN, Brazil was used. The farm integrates the buffalo milk-recording programme coordinated by the Department of Animal Science, São Paulo State University (Unesp), Jaboticabal-SP, Brazil. Animals were raised on pasture with feed supplementation during the dry period. The farm has 600 lactating buffaloes on average. The herd uses fixed-time artificial insemination and natural mating (∼1 bull to 30 cows). Machine milking was performed twice daily and milk yield and its components were measured monthly.
The production traits studied were: milk yield (MY), fat yield (FY), fat percentage (%F), protein yield (PY), protein percentage (%P) and somatic cell counting (SCC). The milk, protein and fat yield records were obtained starting on the fifth day after calving and were truncated at 305 days of lactation. The fat and protein percentage and SCC were obtained by averaging the monthly test-day records per lactation. Only buffaloes that had their first test-day record before 45 days after calving were considered in the analyses. The lactations smaller than 150 days were excluded. The SCC values were obtained by averaging monthly test-day records per lactation; SCC was transformed into SCS as described by Dabdoub and Shook (Citation1984). The fertility traits studied were: age at first calving (AFC), calculated by the date at first calving minus the date of dam birth, and calving interval (CI), calculated as the difference, in days, between subsequent calvings.
Sample collection and DNA extraction
Hair follicle samples from the Murrah buffaloes were collected and stored at 4°C until DNA extraction. Genomic DNA was extracted by the phenol–chloroform-isoamyl alcohol method (Sambrook et al. Citation1989). The purity and concentration were measured with an ultra-violet (UV) spectrophotometer (UV Mini1240, Shimadzu) with the absorbance reading at 260 and 280 nm. The A260/A280 ratio was used as an indication of DNA purity.
MUC1 polymorphism analysis
The forward and reverse primer sequences used to amplify the specifics regions of MUC1 (5’ – CGCAGAACTACGCCAGTTTC – 3’ and 5’ – AGAGCGGGTGGTCATGGA – 3’) were based on the sequence of the Bubalis bubalis MUC1 gene (Genbank under accession number JQ796281) and on the Bos Taurus mucin1 gene sequence. The bubaline primers sequences was adaptated from bovine primers previously described by Souza et al. (Citation2007) according with the specific sequence of MUC1 bubaline sequence. Primer-Blast (NCBI) was used to analyse the primers sequences for GC content, primer-dimer and hairpin formation, and primer melting temperature.
For the PCR assay, 100 ng of genomic DNA was mixed with 15pmol of each primer, Hot Start PCR Master Mix (Qiagen GmbH, Hilden, Alemanha) and with nuclease-free water in a total volume of 25 µl. PCR was carried out in a GeneTouch PCR Thermal cycler (Applied Biosystems, Foster City, USA) under the following conditions: an initial incubation at 95°C for 15 min, 37 cycles of denaturation at 94°C for 30 s, primer annealing at 58°C for 30 s and extension at 72°C for 1 min and 30 s. A final extension was carried out at 72°C for 10 min.
The amplified fragments were checked in a 2.0% agarose gel electrophoresis stained with GelRed™ (Biotium, USA) in 0.5 X Tris-borate-ethylenediaminetetraacetic acid (EDTA) buffer. The gel was photographed using a UV transluminator and the length of the alleles was estimated using the GeneRuler™ DNA Ladder Plus molecular weight marker (Fermentas International, Inc., Burlington, Ontario, Canada). The sequencing was performed by Macrogen. The Tandem Repeat Finder Program was used to identify and count the number of repetitions of the sequence (Benson Citation1999).
Buffaloes enrolment criteria
The following inclusion criteria were applied for the animals: (1) age at first calving between 24 and 48 months of age, (2) daughters of 29 bulls, with a minimum of five daughters per bull and a maximum of 20 daughters per breeder, (3) lactation length > 90 days, (4) assigned to contemporary group (GC) based on year of birth and calving season: October-march or April-September.
Statistical analysis
Analysis of variance was performed using the PROC MIXED procedure of SAS v 9.2 (SAS Institute, Inc., Cary, NC, USA). The SCC distribution was not normal and, therefore, was transformed to a logarithmic scale (CCSt), using the following function: CCSt = (log2(CCS/100.000))+3, previously described by Dabdoub and Shook (Citation1984). The mean of milk production and fertility traits for the different genotypes were analyzed using the Tukey test (P < 0.05). Association analysis between MUC1 genotypes and the traits studied was performed applying the following statistical model:where,
milk yield, fat and protein yields, fat and protein percentages, somatic cell count, age ate first calving and calving interval;
is the constant inherent to all observations;
fixed effect of contemporary group (formed by year and season);
random effect of breeder, with mean 0 and variance
;
fixed effect of marker genotype;
linear regression coefficient of trait
in relation of buffalo age at measurement;
quadratic regression coefficient of trait
in relation of buffalo age at measurement;
buffalo age at measurement;
buffaloes average age evaluated at measurement;
random residual effect associated with trait
with mean 0 and variance
. For AFC, the age at measurement regression was not included in the model.
The association analyses were based on the methods described by Byun et al. (Citation2010), Forrest et al. (Citation2009), Lin et al. (Citation2009), and Hickford et al. (Citation2010). After analyzing the associations between MUC1 genotypes and milk traits, the additive and co-dominance effects of the significant MUC1 genotypes were tested. First, it was evaluated the effect of the presence/absence of the MUC1 alleles on each trait. The allele presence or absence (coded as 1 or 0, respectively) was considered in place of the marker genotype.
A second set of analyses was performed in order to evaluate the effect of the number of copies of each allele on each trait. The number of copies (0, 1 and 2) of each allele was considered, whereas the number of copies = 2 corresponds to the homozygous form. Afterwards, Bonferroni correction was applied at 5% of significance for all traits.
Results
Three alleles of different lengths (600, 900 and 1000 bp) were amplified and named allele 1, 2 and 3, respectively. Allele 2 was the predominant with a frequency of 0.56, followed by allele 3 with a frequency of 0.32 in the population evaluated (). Genotype 2/3 was the most frequent in all buffaloes with a frequency of 0.41, followed by the genotype 2/2 with a frequency of 0.33. The genotype 1/3 was the least frequent.
Table 1. Allele and genotype frequencies of the MUC1 gene in total samples of 179 Murrah water buffaloes.
The statistical analysis considering the presence/absence of the MUC1 alleles revealed that the alleles 1 and 3 were associated with some economic traits studied (). A significant effect for the presence of allele 1 was observed for higher mean for SCC (mean > 7.52) and this effect remained significant after the Bonferroni correction. In addition, greater mean (4.39) for %P was observed in the presence of allele 1 and low PY (mean of 82.45) in the absence of allele 3. In contrast, these effects on PY and %P did not remain significant after the Bonferroni correction. Moreover, the absence of allele 3 was significantly associated with higher mean (862.12) for AFC. This effect, however, was not significant after the Bonferroni correction.
Table 2. Means for the associations between MUC1 alleles and milk production and fertility traits in Murrah water buffaloes.
The effect of the number of copies of each allele in each trait is reported in . Considering SCC, animals with 2 copies of allele 1 presented means significantly higher than animals with any or a single copy of this allele. For %F was observed that animals with no copy of allele 2 presented means significantly higher than animals with one or two copies of this allele. Additionally, animals with 2 copies of allele 2 showed the lower means for %F. Regardless of FY, the animals with 2 copies of allele 1 presented the highest mean for this trait. Overall, only the effect of 2 copies of allele 2 associated with the lower means for %F remained significant after Bonferroni correction.
Table 3. Means obtained for the effects of the number of copies of each allele of the MUC1 gene on milk production and fertility traits in Murrah water buffaloes.
Discussion
The repeat in the VNTR corresponds to a 60 nucleotides sequence not perfect conserved in bovine MUC1 gene. Due to the number of repeats, the VNTR varies in length in many species with exception in Mus musculus. The present study described the VNTR polymorphism of the MUC1 gene in buffaloes from 600 to 1000 bp. In humans, the number of repeats at the VNTR region ranges from 25 and 125 with size ranging from 1,000–5,000 bp (Ando et al. Citation1998). In ruminants, the VNTR region varied from 1,500–3,000 bp in goats (Sacchi et al. Citation2004), from 778 to 1894bp in bovines (Rasero et al. Citation2002; Souza et al. Citation2007; Sando et al. Citation2009), four alleles of approximately 1500 bp in sheep (Rasero et al. Citation2007) and from 1206 to 1566 bp in yaks (Zheng et al. Citation2009).
The gene MUC1 bovine is located in the BTA3q13 chromosome while in buffaloes it is located in the BBU6q13 chromosome (Perucatti et al. Citation2006). In ruminants, the VNTR polymorphism of the MUC1 gene has been extensively associated with weight at different ages in Nellore beef heifers (Souza et al. Citation2012). Also, Hens et al. (Citation1995) reported an association between the VNTR polymorphism of the MUC1 gene with yield, health and reproductive traits in Holstein cows. Furthermore, due to the expression pattern and properties of the mucin 1, the allele size is a putative factor for an epithelial barrier against pathogens. Additionally, it might play a role in mammary disease resistance and also, in conception rate due to the MUC1 expression on the surface of uterine epithelial cells (Patton et al. Citation1999; Rasero et al. Citation2007; Souza et al. Citation2007).
In the present study, allele 1 was associated with higher means for SCC, suggesting a potential relationship between alleles with low number of repeats to high susceptibility to infection in the mammary gland. Undergoing intramammary infection, macrophages, members of the innate immune system, serve as the first line of defense against invading pathogens in the mammary gland and together with the mammary epithelial cells, they release proinflammatory cytokines (such as interleukins and tumour necrosis factor (TNF)) which are signalling molecules for the recruitment and activation of neutrophils to the site of infection (Lewandowska-Sabat et al. Citation2013). Upon activation, neutrophils release enzymes such as myeloperoxidase, which displays cytotoxic oxidant, crucial for the infection resolution (Witko-Sarsat et al. Citation2000). In addition, during inflammation, neutrophils are the predominant cell type in milk, which result in approximately 90% of the total SCC in mastitic milk (Meredith et al. Citation2013). Thus, SCC is used to monitor mastitis incidence in a herd. An increase in milk SCC corresponds to an afflux of white blood cells (mainly neutrophils) that come from the bloodstream into the milk to eliminate infection in the udder. Therefore, SCC is closely linked to the magnitude of the inflammatory status. Moreover, the genetic correlation between SCC and clinical mastitis is high (approximately 0.70). Thus, it is expected that many of the genes affecting SCC would also affect the resistance to mastitis (Sahana et al. Citation2008). Taking the above considerations together, the identification of candidate genes which is associated with a major effect on SCC, form a powerful marker to mastitis resistance.
Taking the above considerations, molecules of MUC1 protein with a smaller length could provide less protection to the mammary gland against intramammary infections due the possibility of low adhesion of microorganisms in the mucus and further excretion before infection. Thus, an increase in SCC in the milk of these animals would be observed as an inflammatory response. An in vitro approach reported by Sando et al. (Citation2009) demonstrated Escherichia coli adhesion to MUC1 expressed in mammary epithelial cells, highlighting the protection function of the protein. However, these authors were not observed association between MUC1 and SCC. In addition, Hens et al. (Citation1995) not reported correlation between mucin 1 and SCC.
The effect of MUC1 gene polymorphism on %P, PY, %F and FY obtained in the present study could be explained by linkage disequilibrium of MUC1 with other genes that have observed effects for these traits. Because based on the biological function of the protein such responses could not be explained. In bovine, whereas the MUC1 gene lies in the BTA3 chromosome, there is evidence of quantitative trait loci (QTL) for %F (Viitala et al. Citation2003; Chun-hua et al. Citation2014), for FY (Heyen et al. Citation1999; Chun-hua et al. Citation2014), %P (Rodriguez-Zas et al. Citation2002; Viitala et al. Citation2003; Chun-hua et al. Citation2014), PY (Heyen et al. Citation1999) and for SCC (Chun-hua et al. Citation2014). Hens et al. (Citation1992) showed evidence for the association between MUC1 with milk protein, fat yields and milk fat percentage in Holstein dairy cows. Moreover, Sando et al. (Citation2009) reported significant associations between MUC1 and milk protein and fat percentages in Holstein Friesian cows.
Regardless of female fertility traits, mucin 1 has been investigated in many species. There is substantial evidence in the literature that MUC1 expression is associated with uterus overall health, maintenance of cell surface hydration as well as implanting blastocysts (Eriksen et al. Citation1998; Savaris et al. Citation2008; Thirkill et al. Citation2007). Regarding to fertility traits, Hens et al. (Citation1992) demonstrated the MUC1 protein association with age at first calving in Holstein cows.
Although, mucin 1 has been shown to be associated with female reproductive traits, it is possible that the polymorphism identified in the MUC1 gene could be in linkage disequilibrium with others regions of the chromosome 3, which might have significant fertility traits. For instance, a study in Nordic Red Cattle reported single nucleotide polymorphisms (SNPs) on the chromosome 3 associated with fertility traits (Höglund et al. Citation2015).
In summary, our results demonstrated that MUC1 gene is highly associated with traits related to mastitis resistance and milk fat synthesis (i.e. SCC and %F). However, it was demonstrated a mild association between MUC1 gene and milk protein synthesis (PY and %P) and also a moderate effect on reproductive traits (i.e. age at first calving). Despite these results, the real effect of MUC1 in these traits may be more investigated using a sample with more animals to improve the force of the statistical analysis.
Conclusions
The MUC1 gene in water buffaloes of Murrah breed is also polymorphic as in others ruminant species. In addition, MUC1 gene might be a potential marker to marker-assisted selection for intramammary infection and some milk production traits.
Acknowledgements
Thanks for CNPq for the financial support and for Department of Animal Science, São Paulo State University (Unesp), Jaboticabal-SP for provide the DNA samples.
Disclosure statement
No potential conflict of interest was reported by the author(s).
Additional information
Funding
References
- Ando I, Kukita A, Soma G, Hino H. 1998. A large number of tandem repeats in the polymorphic epithelial mucin gene is associated with severe acne. J Dermatol. 25:150–152.
- Benson G. 1999. Tandem repeats finder: a program to analyse DNA sequences. Nucleic Acids Res. 27:573–580.
- Byun SO, Zhou H, Frampton CM, Hickford JGH. 2010. No association between variation in the ovine calpastatin gene and either longevity or fertility in sheep. Anim Genet. 41:223–224.
- Chun-hua Q, Qin C, Gui-yan C, Yi Z, Qin Z, Sheng-li Z, Dong-xiao S. 2014. Mapping QTLs affecting economic traits on BTA3 in Chinese Holstein with microsatellite markers. J Int Agric. 13:1999–2004.
- Dabdoub SAM, Shook GE. 1984. Phenotypic relations among milk yeld, somatic cell count, and clinical mastitis. J Dairy Sci. 67:163–164.
- Eriksen GV, Carlstedt I, Uldbjerg N, Ernst E. 1998. Cervical mucins affect the motility of human spermatozoa in vitro. Fertil Steril. 70:350–354.
- Forrest RH, Itenge-Mweza TO, McKenzie GW, Zhou H, Frampton CM, Hickford JG. 2009. Polymorphism of the ovine beta3-adrenergic receptor gene (ADRB3) and its association with wool mean staple strength and yield. Anim Genet. 40:958–962.
- Gendler SJ. 2001. MUC1, the Renaissance Molecule. J Mammary Gland Biol Neoplasia. 6:339–353.
- Hens JR, Rogers GW, Huott ML, Patton S. 1995. Associations of epithelial mucin, PAS-1, with yield, health and reproductive traits in Holstein dairy cows. J Dairy Sci. 78:2473–2480.
- Heyen DW, Weller JI, Ron M, Band M, Beever JE, Feldmesser E, Da Y, Wiggans GR, VanRaden PM, Lewin HA. 1999. A genome scan for QTL influencing milk production and health traits in dairy cattle. Physiol Genomics. 11:165–175.
- Hickford JG, Forrest RH, Zhou H, Fang Q, Han J, Frampton CM, Horrell AL. 2010. Polymorphisms in the ovine myostatin gene (MSTN) and their association with growth and carcass traits in New Zealand Romney sheep. Anim Genet. 41:64–72.
- Höglund JK, Sahana G, Brondum RF, Guldbrandtsen B, Buitenhuis B, Lund MS. 2015. Fine mapping QTL for female fertility on BTA04 and BTA13 in dairy cattle using HD SNP and sequence data. BMC Genomics. 15:790.
- Kumar PR, Singh SK, Kharche SD, Govindaraju CS, Behera BK, Shukla SN, Kumar H, Agarwal SK. 2014. Anestrus in cattle and buffalo. Advanc Anim Vet Sci. 2:124–138.
- Lewandowska-Sabat A, Boman G, Downing A, Talbot R, Storset A, Olsaker I. 2013. The early phase transcriptome of bovine monocyte-derived macrophages infected with Staphylococcus aureus in vitro. BMC Genomics. 14:891.
- Lin L, Flisikowski K, Schwarzenbacher H, Scharfe M, Severitt S, Blocker H, Fries R. 2009. Characterization of the porcine AMPK alpha 2 catalytic subunitgene (PRKAA2): genomic structure, polymorphism detection and association study. Anim Genet. 41:203–207.
- Lucy MC. 2001. Reproductive loss in high-producing dairy cattle: where will it end? J Dairy Sci. 849:1277–1293.
- Meredith BK, Berry DP, Kearney F, Finlay EK, Fahey AG, Bradley DG, Lynn DJ. 2013. A genome-wide association study for somatic cell score using the Illumina high-density bovine beadchip identifies several novel QTL potentially related to mastites susceptibility. Front Genet Livest Genomics. 4:229.
- Patton S, Gendler SJ, Spicer AP. 1995. The epithelial mucin, MUC 1, of milk, mammary gland and other tissues. Biochim Biophys Acta. 1241:407–424.
- Perera BMAO. 2011. Reproductive cycles of buffalo. Anim Reprod Sci. 124:194–199.
- Perucatti A, Floriot S, Di Meo GP, Soglia D, Rullo R, Maione S, Incarnato D, Eggen A, Sacchi P, Rasero R, Iannuzzia L. 2006. Comparative FISH mapping of mucin, transmembrane (MUC1) among cattle, river buffalo, sheep and goat chromosomes: comparison between bovine chromosome 3 and human chromosome 1. Cytogenet Genome Res. 112:103–105.
- Raden V. 2004. Selection on net merit to improve life time profit. J Dairy Sci. 87:3125–3131.
- Rasero R, Bianchi L, Cauvin E, Maione S, Sartore S, Soglia D, Sacchi P. 2007. Analysis of the sheep MUC1 gene: Structure of the repetitive region and polymorphism. J Dairy Sci. 90:1024–1028.
- Rasero R, Sacchi P, Rosati S, Cauvin E, Maione S. 2002. Molecular analysis of the length polymorphic MUC1 gene in cattle. J Anim Breed Genet. 119:342–349.
- Rodriguez-Zas SL, Southey BR, Heyen DW, Lewin HA. 2002. Detection of quantitative trait loci influencing dairy traits using a model for longitudinal data. J Dairy Sci. 85:2681–2691.
- Sacchi P, Caroli A, Cauvin E, Maione S, Sartore S, Soglia D, Rasero R. 2004. Analysis of the MUC1 gene and its polymorphism in Capra hircus. J Dairy Sci. 87:3017–3021.
- Sahana G, Lund MS, Andersson-Eklund L, Hastings N, Fernandez A, Iso-Touru T, Thomsen B, Viitala S, Sørensen P, Williams JL, Vilkki J. 2008. Fine-mapping QTL for mastitis resistance on BTA9 in three Nordic red cattle breeds. Anim Genet. 39:354–362.
- Sambrook J, Fritschi EF, Maniatis T. 1989. Molecular cloning: a laboratorymanual. New York: Cold Spring Harbor Laboratory Press.
- Sando L, Pearson R, Gray C, Parker P, Hawken R, Thomson PC, Meadows JR, Kongsuwan K, Smith S, Tellam RL. 2009. Bovine Muc1 is a highly polymorphic gene encoding an extensively glycosylated mucin that binds bacteria. J Dairy Sci. 92:5276–5291.
- Savaris RF, Da Silva LC, Moraes GS, Edelweiss MI. 2008. Expression of MUC1 in tubal pregnancy. Fertil Steril. 89:1015–1017.
- Souza FRP, Dentillo DB, Meola J, Biase FH, Andrea MV, Vozzi PA, Lôbo RB, Martelli LR. 2007. The polymorphism in MUC1 gene in Nelore cattle. J Anim Breed Genet. 124:42–46.
- Souza FRP, Maione S, Sartore S, Soglia D, Spalenza V, Calvin E, Martelli LR, Mercadante MEZ, Sacchi P, Albuquerque LG, Rasero R. 2012. MUC1 gene polymorphism in three Nelore lines selected for growth and its association with growth and carcass traits. Mol Biol Reports. 39:1541–1549.
- Thirkill TL, Cao T, Stout M, Blankenship TN, Barakat A, Douglas GC. 2007. MUC1 is involved in trophoblast transendothelial migration. Biochim Biophys Acta. 1773:1007–1014.
- Viitala SM, Schulman NF, de Koning DJ, Elo K, Kinos R, Virta A, Virta J, Maki-Tanila A, Vilkki JH. 2003. Quantitative trait loci affecting milk production traits in Finnish Ayrshire dairy cattle. J Dairy Sci. 86:1828–1836.
- Witko-Sarsat V, Rieu P, Descamps-Latscha B, Lesavre P, Halbwachs-Mecarelli L. 2000. Neutrophils: molecules, functions and pathophysiological aspects. Laborat Invest. 80:617–653.
- Zheng Y, Fan Q, Liu Y, Zhao X, He X, Jin S. 2009. Analysis of Yak MUC1 protein polymorphisms and the corresponding VNTR structure. Anim Biotechnol. 20:231–237.