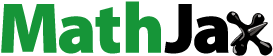
ABSTRACT
This research paper addresses the hypothesis that mineral supplementation (sodium chloride and magnesium oxide at 1:1 weight ratio) for dairy heifers grazing mature wheat in the spring-summer period will improve live weight gain compared with the non-supplemented group. In addition, the potential of mineral supplementation to reduce environmental pollution through diluting urinary nitrogen content was evaluated in this study. The grazing study was conducted at the Dookie Campus, The University of Melbourne, Australia between September and November 2017. The study comprised two temporal trial replications with two dietary treatments; graze wheat with (supplemented, SUP) or without mineral supplementation (control, CTR). The first and second trial replications used 22 and 24 heifers, respectively to conduct a 22-day and 21-day weight gain measurement. Heifers in the CTR group had 11% lower daily water intake than those in the SUP group. No differences were observed in heifers’ weight gain and urinary nitrogen content and excretion. The study indicates that protein deficiency may override the mineral deficiency when heifer grazed mature wheat, and this may have led to no mineral supplementation effect on heifer performance. The level of protein in grazing wheat needs to be considered in feeding minerals to heifers.
Introduction
Wheat is a common grazing crop for cattle and sheep in Australia due to its high nutritive value (Dove and Mcmullen Citation2009), which is comparable to mixed pastures like sub clover (Trifolium subterraneum), annual grass, phalaris (Phalaris aquatica) (Robertson et al. Citation2011). Early sown wheat can be used as a dual-purpose crop to support animal grazing and then harvest for grain or hay (Mcmullen and Virgona Citation2009). Dual-purpose wheat is generally grazed in the vegetative stage in late winter and early spring in Australia (Hersom et al. Citation2004), and then harvested for hay or grain in late spring/summer. Wheat grazing is also practiced in United States (Epplin et al. Citation2000), Argentina, Morocco, Pakistan, Syria, and Uruguay (Rodriguez et al. Citation1990).
Both dual-purpose winter and spring wheat crops may have a role as an important low-cost feed source that could provide green forage when annual pasture has not grown enough to be grazed (Doole et al. Citation2009; Moore Citation2009). However due to climate change, Northern Victoria, Australia is experiencing higher spring temperatures and more frequent heatwaves, less cool season rainfall, more time in drought, decreased water availability (Victoria’s Climate Science Report Citation2019). Those changes would lead to changes in wheat plant physiology and nutritional value. For example, high ambient temperature and water deficit slow photosynthesis and leaf area expansion, subsequently the flow of carbohydrates and other resources to the developing plant parts in wheat which may reduce dry matter production (Ferris et al. Citation1998).
It is well known that grazing wheat often can lead to sodium (Na) and magnesium (Mg) deficiency, as well as Na: Mg imbalance in the animal diet (CSIRO Citation2007). The Mg and Na deficiency is linked with a reduced animal Mg absorption, from wheat growing in soils with low pH and high potassium (K), which is the case in south-eastern Australia (Coventry et al. Citation1987; Gorhma et al. Citation1987). The consequence can be a high K: Na in the rumen, which further reduces Mg absorption and impairs animal performance (Berger Citation1992).
Monensin (Santos et al. Citation2019) and Zeolite (Khachlouf et al. Citation2019) have been extensively used to manipulate nitrogen (N) intake and mineral status in dairy cows. Supplementation of livestock grazing wheat with Na and Mg can increase sheep live weight gain (LWG) by up to 60% (Mulholland and Coombe Citation1979; Rowe et al. Citation1989; Dove and Mcmullen Citation2009). Little is known about how cattle will perform when grazing wheat and supplemented with Na and Mg. The optimal dietary requirement of Na for cattle is 0.8–1.2 g/kg dry matter (DM), which is higher than for sheep (0.7–1.0 g/kg DM; Blackwood and Duddy Citation2009). In addition, the animal response is also dependent on wheat mineral content at the time of grazing. The mineral content generally decreases in wheat as it matures (Cottle Citation1991), and this may raise the mineral demand for supplementation compared to feeding cattle with wheat at the vegetative stage. Therefore, the hypothesis of this study is that supplementation of Na and Mg for cattle grazing mature wheat in the spring-summer period will improve live weight gain (LWG) compared with the non-supplemented group.
In addition to the potential animal LWG benefit, the mineral supplementation could be a way to mitigate environmental pollution from grazing cattle. Mineral supplementation can increase cattle water intake and result in an increase in the urine output to dilute its N concentration (Spek et al. Citation2012). Under the grazing system, previous studies observed that supplementing cattle with salt increased urine volume by 28% and reduced urinary N concentration by 36%, respectively (Liu and Zhou Citation2014; Ledgard et al. Citation2015). Therefore, it is logical to hypothesize that supplementation of Na and Mg, while cattle graze mature wheat in the spring-summer period, will lead to a lower urinary N concentration compared with the non-supplemented group.
The objectives of this study were to (1) measure the effects of mineral supplementation on LWG and urinary N concentration of heifers grazing mature wheat in early summer, and (2) analyse blood and urine samples for metabolism biomarkers to understand the potential mechanisms that underpin the animal performance difference due to mineral supplementation.
Materials and methods
Ethical statement
This study utilized dairy heifers from the Dookie Campus, The University of Melbourne. The study was undertaken with the approval of The University of Melbourne Animal Ethics Committee (#1714219.1 and #1714290.1).
Paddock and forage preparation
A 10-hectare (ha) paddock in Dookie (36.37°S, 145.70°E) was sown with canola in May 2016 and then followed by sowing wheat in April 2017. A portion of the paddock was sub-divided into a 2.4 ha and a 4.8 ha block for grazing trial temporal replication 1 and 2, respectively. The grazing area difference in replication 1 and 2 reflects more feed was offered to heifers in temporal replication 2 than replication 1, due to increased heifer intake demand and reduced feed quality. The maximum and average rainfall were 22.2 and 3.17 mm/day; 39.4 and 1.97 mm/day for trial replication 1 and 2, respectively. The average of maximum temperature for trial replication 1 and 2 were 15.8°C and 20.6°C respectively.
The paddock was sprayed with glyphosate (1.2 L/ha), Logran-B-Power (40 g/ha), and Sakura 850WG (118 g/ha) prior to sowing on 19 April 2017 using a 3000 L Hardie 30 mt boom spray. Wheat (cv. Whister ASW; 85 kg seed/ha) was sown on 20 April 2017 with monoammonium phosphate (MAP) fertilizer applied at a rate of 100 kg/ha at a row spacing of 25 cm using a seeder (Gasen air cart seeder, with RFM seeder bar, Australia). Flutriafol 500 was also applied as a dressing on MAP with a rate of 300 mL/ha on 20 April 2017. To control weeds, Tigrex (0.75 L/ha), Clopyralid 600 (80 mL/ha), Clodinaftop 240EC (85 mL/ha) and BS1000 (0.10%) were applied through a water solution (70 L/ha) on 18 June 2017 with a 2006 3000 L Hardie sprayer. Urea was applied at a rate of 80 kg/ha on 2 August 2017 using a fertilizer spreader (2012 JCB precision spreader, Australia).
Grazing study design
The grazing study comprised two temporal trial replications with two grazing treatments. The two treatments were: grazing wheat only, which served as a control group (CTR) and grazing wheat with mineral supplementation (SUP). Each treatment group was allocated with half of the grazing block in temporal replication 1 (1.2 ha) and 2 (2.4 ha). Two minerals were (i.e. magnesium oxide and coarse salt) mixed at 1:1 ratio (weight: weight) and offered to grazing heifers ad libitum. Magnesium oxide was purchased from Amtrade International Pty Ltd, Melbourne, Australia while coarse salt was purchased from Copland & Sons, Lake Charm Salt Co., Australia. The minerals were offered to heifers using a feed trough and their intake was quantified by weighing the offered and refused mineral (kg) at the start and end of each trial replication.
The first and second trial replications used a total of 22 and 24 Holstein-Friesian heifers, respectively. The first replication was conducted from 7 September to 13 October 2017, and the second replication was conducted from 19 October to 22 November 2017. Each trial replication consisted of a 14-day adaptation period and a measurement period: the first and second trial replications had a 22-day and 21-day measurement period, respectively. Heifers were born in August-September 2016 and equally allocated into two treatment groups balanced for LW (mean ± SD: 319 ± 25.4 kg for trial replication 1; 365 ± 28.8 for trial replication 2) at the start of adaptation period. The heifers were managed under a set stocking system. Each group was provided with a portable water trough to allow ad libitum access to water.
Forage allocation
The forage allowance for each heifer was calculated according to the metabolizable energy (ME) requirements for maintenance plus a 0.8 kg daily LWG (Nicol and Brookes Citation2007), with an assumption that wheat ME content was 10 MJ ME/kg DM: heifer forage allowance (kg DM/heifer per day) = [0.65 × LW (kg)0.75 + 30 (MJ ME/heifer per day)] ÷ 10 (MJ ME/kg DM) (Nicol and Brookes Citation2007). Individual heifer forage allowance was then multiplied by the number of heifers in the group and the number of grazing days to calculate group forage allowance. To allocate feed, pre-grazing forage mass was determined by cutting 30 of 0.5 m rows of wheat randomly to ground-level per treatment group, with an assumption of a post-grazing forage mass was 1500 kg DM/ha.
Forage quality measurements
Forage quality samples were collected by cutting 15 samples (0.01 m2) per treatment group at random locations, to ground-level at the start and end of each replication. Forage samples were weighed fresh, oven-dried at 65° C for 48 h to determine DM content. The oven-dried wheat samples were then ground to 1 mm for analysis of nutritive value at the New South Wales Department of Primary Industries (Australia) Laboratory Services. Near-infrared reflectance spectrophotometry (Foss NIRSystems 5000, FOSS NIRSystems Inc, USA) was used to estimate N, crude protein (CP; N × 6.25), the digestible organic matter content in the dry matter (DOMD), and neutral detergent fibre (NDF). The ME was calculated from predicted DOMD [ME (MJ/kg DM) = DOMD (%) × 0.203–3.001] (Ministry of Agriculture, Fisheries and Food Citation1990). For mineral analysis [K, Na, calcium (Ca) and Mg], samples were digested with concentrated nitric acid and hydrogen peroxide at a maximum temperature of 125°C for 2 h. Digestate was then diluted and analyzed on a Perkin Elmer Optima 8300DV ICP-OES (PerkinElmer, Inc., Waltham, USA). This analysis was executed on a LECO Trumac CN (LECO Corporation, Michigan, USA) at a furnace temperature of 1350°C.
Dry Matter and Water Intake
Heifer dry matter intake (DMI) was estimated through back-calculation base on daily ME requirement (i.e. maintenance + LWG) ÷ forage energy content (MJ ME/kg DM) (Nicol and Brookes Citation2007):
If LWG > 0
If LWG < 0
Animal measurements
All heifers were weighed after a 12-hour overnight fasting at the start and the end of the measurement period. Heifers were sampled for urine and blood on measurement day 22 of the first replication and day 16 of the second replication. For each trial replication, 6 randomly selected heifers from each treatment group were herded into a cattle yard and sampled for urine and blood. Urine was collected mid-stream following vulva stimulation and acidified immediately to a pH <3.0 with concentrated sulfuric acid to prevent ammonia volatilization (Cheng et al. Citation2016). One 8-mL blood sample per heifer was collected from the coccygeal vein using sodium heparin vacuette tubes (BD Vacutainer, Belliver Industrial Estate, UK). The blood was then centrifuged at 3000 g at 4°C for 15 min to harvest plasma. The urine and plasma samples were then stored at −20°C for further analysis. Urinary N concentration was determined by N analyzer (Vario MAX CN, Elementar Analysen systeme, Hanau, Germany) while urinary salinity was determined using a hand-held refractometer (Opti Digital Range Life Science 38-28, Bellingham and Stanley, USA). Urinary creatinine and plasma urea were analyzed using a Cobas Integra 400 plus (Roche, Switzerland). Urinary N excretion (UN) during the measurement period was estimated from plasma urea N and LW using the equation published by Kohn et al. (Citation2005): UN (g N/day) = 1.3 x plasma urea N (g/L) x LW (kg). The LW value used in these calculations was the average LW of the start and end of the measurement period.
Data analysis
This study was analyzed as two temporal trial replications by ANOVA using GenStat (VSN International, Hemel Hempstead, UK, version 16; Cheng et al. Citation2016). Diet measurements were taken in each replication and these measurements were nested in the temporal replications. The model for diet measurements was
where
was ith measurement in the jth temporal replication for the kth diet treatment.
The model for animal-based measurements was
where
was the measurement for the kth animal in diet treatment i and temporal replication j. Urine and plasma data were averaged across sampling days before analysis.
Results
Forage allocation and nutritive value
The pre-grazing herbage mass was higher in the CTR group than in the SUP group (). Forage Na, Ca and Mg content did not differ between groups, but K was higher in forage wheat grazed by heifers in the CTR group than the SUP group (). No statistical differences were observed in NDF, ME, ADF, ASH, OM, DMD, DOMD and the water-soluble carbohydrate (WSC) content between two groups ().
Table 1. Pre-grazing forage mass, dry matter content and nutritive value of forage wheat grazed by heifers in supplemented with (SUP) and without (CTR) minerals.
Animal intake and growth performance
Estimated ME intake and CP intake were higher (P < 0.001; ) in the CTR group than those in the SUP group. Heifers in the CTR group had 10% higher feed water intake than those in the SUP group (P < 0.001). Heifers in the CTR group had 11% lower daily water intake than those in the SUP group. No significant differences were observed in LWG (P = 0.97; ).
Table 2. Live weight change, intake, plasma urea, urine salinity, nitrogen, creatinine and estimated urinary nitrogen excretion of heifers supplemented with (SUP) and without (CTR) minerals.
Blood and urine Parameters
Heifers in the SUP group had a higher (P < 0.05; ) urine salinity content than those in the CTR group. Urinary N concentration did not differ significantly (P = 0.87) between the two groups. On the other hand, urinary creatine concentration tended to be higher (P = 0.11) in the CTR group compared with that in the SUP group. No differences were observed in plasma urea N and UN (P = 0.97, 0.37 respectively; ).
Discussion
This study examined the effects of supplementing minerals on heifer LWG when grazing mature wheat in the spring-summer period. The ME and CP content were 9.0 MJ/kg DM and 7.1% on DM basis in CTR group while in SUP group those were 9.2 MJ/kg DM and 6.6% on DM basis, respectively. These nutritive characteristics are also low compared with a previous study (Mcgrath et al. Citation2015) that reported grazing wheat with ME 11.8 MJ/Kg DM and CP content of 22.9% on DM basis. In addition, Ca content was 0.09% on DM basis in SUP and CTR group while K content was 1.29, 1.41% on DM basis in CTR and SUP group, respectively in the current study which were also lower than those reported in a previous study of Mcgrath et al. (Citation2015) with a Ca content of 0.28% on DM basis, and K content of 4.8% on DM basis. Average annual rainfall (502 mm) and wheat cultivar (EGA Wedgetail) used in that study (Mcgrath et al. Citation2015) may explain the nutritional variability. Additionally, in Mcgrath et al. (Citation2015) study, nutritional analysis of wheat sample was conducted on June and July, while in the current study it was performed between September and November which may explain the variation in nutritional component. The DMI of heifers in the current study was similar to previous studies, which was in a range of 2.5–3% of heifer LW (Cheng et al. Citation2016, Citation2017a, Citation2017b). Mineral intake was 106 g/day/heifer for the SUP group, which is similar to a previous study of Bell and Dove (Citation2012, p. 140 g/day/heifer).
The previous review from Dove et al. (Citation2016) suggested that mineral supplementation should be provided to animals when the forage diet has less than 0.5% K, 0.07% Na, 0.3% Ca and 0.09% Mg on DM basis. Based on those suggested values, the mature wheat grazed in the current study was deficient in Na, Mg and Ca for cattle production. However, the mineral supplementation did not improve cattle LWG compared with their unsupplemented counterparts in this study. This is in contrast to many earlier studies where sheep and cattle offered vegetative wheat, showed an increase in LWG when minerals were supplemented (Dove and Mcmullen Citation2009; Bell and Dove Citation2012). Such discrepancy in LWG response to mineral supplementation was discussed in a recent review paper (Masters et al. Citation2019), which highlighted that the use of mineral to be considered in conjunction with energy and CP supply in animal production. Based on the ‘leaky bucket theory’, deficiency in major nutrient supply (energy or CP) is the first limiting factor to animal performance rather than mineral deficiency. The lack of LWG to mineral supplementation in this study may be due to inadequate CP supply to the animal. The CP content of the grazed wheat (6.6 and 7.1% on DM basis for the SUP and CTR groups, respectively) was substantially lower than growing heifer’s requirement (14% on DM basis; Pacheco and Waghorn Citation2008). Therefore, the CP deficiency most likely limited the cattle LWG in the current study (Nicol and Brookes Citation2007). This speculation is partly supported by the low LWG (0.5 kg/day/heifer for both groups) measured in this study, which is less than the target of 0.8 kg/day/heifer set at the start of the study. In addition, the current study also showed a lower LWG than observed in some earlier studies, 0.7 kg/day/heifer grazed vegetative wheat in Cheng et al. (Citation2016) and 0.7–0.9 kg/day/heifer grazed herbal pasture in Cheng et al. (Citation2017a, Citation2017b). Previous studies indicated that monensin (Santos et al. Citation2019) and Zeolite (Khachlouf et al. Citation2019) supplementation can improve the DM and CP intake in similar situations.
The current study also examined the potential of using mineral supplementation to mitigate environmental pollution in the grazing system. In the present grazing study, we used the spot urine sample technique to gain an understanding of potential urinary N concentration change due to mineral supplementation. It is important to note that caution is needed to interpret the urine measurements from spot samples, as diurnal variation in urinary N concentration is known to occur (Betteridge et al. Citation2013).
Urine salinity (%) was higher in the SUP group than in the CTR group, which is likely to reflect the higher mineral intake achieved in the SUP group (Spek et al. Citation2012). The current study did not detect a significant change in urinary N concentration. Urinary creatinine predicts urine volume and it is negatively related to urine volume (Chizzotti et al. Citation2008). From the result of having 40% lower urinary creatinine in SUP group it can be speculated that SUP group had higher urine volume which is partly proved by an 11% higher daily water intake in that group. Unfortunately, the large variation between individual cattle and two trial periods reduced the statistical power to detect a significant difference in urinary creatinine (P = 0.11) in this study.
Nitrogen intake is a major driver of UN (Totty et al. Citation2013; Beukes et al. Citation2014), and these two are generally positively correlated with each other (Johnson et al. Citation2016). However, there was no difference in the UN between two treatments in this study, despite that the CTR group was estimated to have 18% more N intake than the SUP group. This is likely due to dietary CP content in the present study which was very low relative to heifer’s requirement. This could have led to a retention or conservation of N and kept urinary N to a minimum (Pacheco and Waghorn Citation2008).
Overall, the study has not observed any effect of mineral supplementation to heifers grazing mature wheat on LWG and reduction of urinary N concentration. A future indoor study is needed to confirm the result by establishing the inter-relationships between feed intake, water intake, urine volume and nutrient output in the urine.
Conclusion
Mature wheat CP content was substantially below the growing heifers’ requirements, which may be the cause of having insignificant differences in LWG and UN between two groups tested in this study. Therefore, the level of dietary CP should be considered by farmers when feeding minerals to animals. A future indoor study is needed to confirm the result by establishing the inter-relationships between feed intake, water intake, urine volume and nutrient output in the urine.
Acknowledgments
We thank Ashley Gabler, Jess Reeks, Tim Reeks, and John Geddes from Dookie Campus, The University of Melbourne for technical support. We thank Jeffery Mccormick and Shawn McGrath from Charles Sturt University (Australia) for useful discussion. The study was supported by the Startup Fund from Faculty of Veterinary and Agricultural Sciences, The University of Melbourne.
Disclosure statement
No potential conflict of interest was reported by the author(s).
References
- Bell L, Dove H. 2012. Feed mineral supplements when grazing cereals. Melbourne: CSIRO Publishing, viewed 02 December 2018, https://grdc.com.au/resources-and-publications/groundcover/ground-cover-issue-98-may-june-2012/mineral-supplements-needed-when-grazing-cereals.
- Berger LL. 1992. Grass tetany: causes and prevention. Salt Trace Miner. 24:1–4.
- Betteridge K, Costall DA, Li FY, Luo D, Ganesh S. 2013. Why we need to know what and where cows are urinating – a urine sensor to improve nitrogen models. Proc NZ Grassland Assoc. 75:119–124.
- Beukes PC, Gregorini P, Romera AJ, Woodward SL, Khaembah EN, David FC. 2014. The potential of diverse pastures to reduce nitrogen leaching on New Zealand dairy farms. Animal Production Science. 54:1971–1979.
- Blackwood I, Duddy G. 2009. Assessing stock feed additives and mineral supplements. New South Wales, Australia: Primary Industries. Viewed on 02 January 2019, https://www.dpi.nsw.gov.au/__data/assets/pdf_file/0019/304912/Assessing-stock-feed-additives-and-mineral-supplements.pdf.
- Cheng L, Al-Marashdeh O, Mccormick J, Guo X, Chen A, Logan C. 2017a. Live weight gain, animal behaviour and urinary nitrogen excretion of dairy heifers grazing ryegrass–white clover pasture, chicory or plantain. N Z J Agric Res. 61:454–467.
- Cheng L, Mccormick J, Hussein AN, Logan C, Pacheco D, Edwards GR. 2017b. Live weight gain, urinary nitrogen excretion, and urination behaviour of dairy heifers grazing pasture, chicory and plantain. J Agric Sci. 155:669–678.
- Cheng L, Mccormick J, Logan C, Hague H, Hodge MC, Edwards GR. 2016. Live weight gain and urinary nitrogen excretion of dairy heifers grazing perennial ryegrass-white clover pasture, canola and wheat. Anim Prod Sci. 58:1073–1078.
- Chizzotti ML, Valadares SCF, Valadares RFD, Chizzotti FHM, Tedeschi LO. 2008. Determination of creatinine excretion and evaluation of spot urine sampling in Holstein cattle. Livest Sci. 113:218–225.
- Cottle DJ. 1991. Australian sheep and wool handbook, 472.
- Coventry DR, Morrison GR, Reeves TG, Fung KKH. 1987. Mineral composition and responses to fertiliser of wheat grown on a limed and deep ripped soil in north-eastern Victoria. Aust J Exp Agric. 27:687–694.
- CSIRO. 2007. Nutrient requirements of domesticated ruminants (Eds) M. Freer, H. Dove and J.V. Nolan. Melbourne: CSIRO Publishing.
- Doole GJ, Bathgate AD, Robertson MJ. 2009. Economic value of grazing vegetative wheat (Triticum aestivum L.) crops in mixed-farming systems of Western Australia. Anim Prod Sci. 49:807–815.
- Dove H, Masters G. D., Thompson N. A.. 2016. New perspectives on the mineral nutrition of livestock grazing cereal and canola crops. Anim. Prod. Sci. 56:1350–1360
- Dove H, Mcmullen KG. 2009. Diet selection, herbage intake and live weight gain in young sheep grazing dual-purpose wheats and sheep responses to mineral supplements. Anim Prod Sci. 49:749–758.
- Epplin FM, Hossain I, Krenzer EG, Jr. 2000. Winter wheat fall–winter forage yield and grain yield response to planting date in a dual-purpose system. Agric Sys. 63:161–173.
- Ferris R, Ellis RH, Wheeler TR, Hadley P. 1998. Effect of high temperature stress at anthesis on grain yield and biomass of field-grown crops of wheat. Ann Bot. 82:631–639.
- Gorhma J, Hardy C, Wyn Jones RG, Joppa LR, Law CN. 1987. Chromosomal location of a K/Na discrimination character in the D genome of wheat. Theor Appl Genet. 74:584–588.
- Hersom MJ, Horn GW, Krehbiel CR, Phillips WA. 2004. Effect of live weight gain of steers during winter grazing: I. Feedlot performance, carcass characteristics, and body composition of beef steers. J Anim Sci. 82:262–272.
- Johnson ACB, Reed KF, Kebreab E. 2016. Short communication: evaluation of nitrogen excretion equations from cattle. J Dairy Sci. 99:7669–7678.
- Khachlouf K, Hamed H, Gdoura R, Gargouri A. 2019. Effects of dietary Zeolite supplementation on milk yield and composition and blood minerals status in lactating dairy cows. J Appl Anim Res. 47:54–62.
- Kohn RA, Dinnees MM, Russek-Cohen E. 2005. Using blood urea nitrogen to predict nitrogen excretion and efficiency of nitrogen utilisation in cattle, sheep, goats, horse, pigs, and rats. J Anim Sci. 83:879–889.
- Ledgard FS, Welten B, Betteridge K. 2015. Salt as a mitigation option for decreasing nitrogen leaching losses from grazed pastures. J Sci Food Agric. 95:3033–3040.
- Liu H, Zhou D. 2014. Mitigation of ammonia and nitrous oxide emissions from pasture treated with urine of sheep fed diets supplemented with sodium chloride. Anim Feed Sci Technol. 192:39–47.
- Masters DG, Norman HC, Thomas DT. 2019. Minerals in pastures - are we meeting the needs of livestock? Crop Pasture Sci. 70:1184–1195.
- Mcgrath SR, Bhanugopan MS, Dove H, Clayton EH, Virgona JM, Friend MA. 2015. Mineral supplementation of lambing ewes grazing dual purpose wheat. Anim Prod Sci. 55:526–534.
- Mcmullen KG, Virgona JM. 2009. Dry matter production and grain yield from grazed wheat in southern New south Wales. Anim Prod Sci. 49:769–776.
- Ministry of Agriculture, Fisheries and Food. 1990. UK tables of nutritive value and chemical composition of feeding stuffs. Aberdeen: Rowett Research Services, 420 pp.
- Moore AD. 2009. Opportunities and trade-offs in dual-purpose cereals across the southern Australian mixed-farming zone: a modelling study. Anim Prod Sci. 49:759–768.
- Mulholland JG, Coombe JB. 1979. Supplementation of sheep grazing wheat stubble with urea, molasses and minerals: quality of diet, intake of supplements and animal response. Aust J Exp Agric Anim Husb. 19:23–31.
- Nicol AM, Brookes IM. 2007. The metabolisable energy requirements of grazing livestock. In: PV Rattray, IM Brookes, AM Nicol, editor. Pasture and supplements for grazing animals. New Zealand: New Zealand Society of Animal Production Occasional Publication No.14; p. 151–172.
- Pacheco D, Waghorn GC. 2008. Dietary nitrogen – definitions, digestion, excretion and consequences of excess for grazing ruminants. Proc NZ Grassland Assoc. 70:107–116.
- Robertson SM, Friend MA, Broster JC, King BJ. 2011. Survival of twin lambs is increased with shrub belts. Anim Prod Sci. 51:925–938.
- Rodriguez A, Trapp JN, Walker OL, Bernardo DJ. 1990. A wheat grazing systems model for the US southern plains: Part I model description and performance. Agric Sys. 33:41–59.
- Rowe JB, Brown G, Ralph IG, Ferguson J, Wallace JF. 1989. Supplementary feeding of young Merino sheep, grazing wheat stubble, with different amounts of lupin, oat or barley grain. Aust J Exp Agric. 29:29–35.
- Santos BCM, Araújo CPA, Venturelli CB, Freitas EJJR, Barletta VR, Gandra RJ, De Paiva GP, Acedo ST, Rennó PF. 2019. Effects of increasing monensin doses on performance of mid-lactating Holstein cows. J Appl Anim Res. 47:297–302.
- Spek JW, Banninkt A, Gort G, Hendriks WH, Dijkstra J. 2012. Effect of sodium chloride on urine volume, urinary urea excretion, and milk urea concentration in lactating dairy cattle. J Dairy Sci. 95:7288–7298.
- Totty VK, Greenwood SL, Bryant RH, Edwards GR. 2013. Nitrogen partitioning and milk production of dairy cows grazing simple and diverse pastures. J Dairy Sci. 96:141–149.
- Victoria’s Climate Science Report. 2019. Department of environment, land, water and planning. https://www.climatechange.vic.gov.au/__data/assets/pdf_file/0029/442964/Victorias-Climate-Science-Report-2019.pdf.