ABSTRACT
To explore the feasibility of land-based farming on mackerel tuna (Euthynnus affinis), the changes of antioxidant, immune and digestive enzyme activities of wild caught and indoor cultured juvenile mackerel tuna were evaluated. The activities of total superoxide dismutase, catalase, peroxidase and alkaline phosphatase in the liver of the wild group were significantly higher than those cultured under captivity. The total superoxide dismutase and peroxidase activities in the indoor cultured group were significantly increased in the head kidney, and the MDA content was significantly increased. The activities of lysozyme in the liver and head kidney, the activities of amylase, lipase and trypsin activities in the intestine were significantly increased in the indoor cultured group. The pepsin activity in the stomach was significantly reduced. The land-based farming increased the digestive enzyme activity compared to the wild caught group. The mode of land-based farming is suitable for growth and fattening of young fish, but it leads to inhibition of antioxidant capacity and increase in immune enzyme activity. This study adds knowledge of our understanding on the physiological response of mackerel tuna and improves future farming practice of this species in an indoor condition.
1. Introduction
The high volume of market demand of tuna and the decrease of tuna population in the wild have promoted the development of tuna farming industry (Benetti et al. Citation2016). At present, the major countries practising tuna farming are Japan, Australia, Mexico, the United States, Canada and some other countries around the Mediterranean Sea including Spain, Turkey, Malta, Croatia, Italy, Tunisia and Greece (Mylonas et al. Citation2010; Buentello et al. Citation2016). The main tuna species for aquaculture include Pacific bluefin tuna (Thunnus orientalis), Atlantic bluefin tuna (Thunnus thynnus), southern bluefin tuna (Thunnus maccoyii), yellowfin tuna (Thunnus albacares) and other smaller tunas (Peng et al. Citation2019). However, the development of tuna aquaculture production is restricted by the technique of artificial breeding of tuna fish, while the vast majority of tuna production still comes from farming juvenile tuna fish captured from the wild (Metian et al. Citation2014). The land-based domestication of juvenile tuna is an important undertaking for development of tuna culture (Chen et al. Citation2016). Current research on tuna mainly focuses on the biology, ecology, fishery management, species identification, genetics and nutritional value (Castrillon et al. Citation1996; Block et al. Citation2001; Graham et al. Citation2007; Rooker et al. Citation2007; Die Citation2016; Bojolly et al. Citation2017). However, little is known on the difference in digestive physiology and immunity between the tuna in the wild and under captivity.
Mackerel tuna (Euthynnus affinis), also named Kawakawa or Bonito, is a species of tuna that lives in the tropical and subtropical waters of the Indo-West Pacific. As a highly migratory species, mackerel tuna are in schoolings in the surface water where the temperature is over 20oC. As the native species in South China Sea, the artificial breeding of mackerel tuna has not been achieved in China, but artificial domestication of this species was established by South China Sea Fisheries Research Institute, Chinese Academy of Fishery Sciences in Hainan Province in 2019. As the first step of artificial breeding of mackerel tuna, the understanding of the physiological responses of this species to artificial rearing environment is essential. Therefore, the present study was conducted to compare the digestive, immune and antioxidant enzyme activities between wild caught and artificially cultured mackerel tunas during the juvenile stage.
2. Materials and methods
2.1. Experiment design and sample collection
Thirty juvenile Euthynnus affinis used in the experiment were initially caught by a fishing boat at the sea (18o22′55.35″ N, 109o58′20.23″E) near Xincun Harbour, Xincun Town, Lingshui County, Hainan Province. Then the juveniles were acclimated in two indoor cement tanks (5 m in diameter and 2.5 m deep) with a recirculating water system in the Tropical Aquaculture Research and Development Center, South China Sea Fisheries Research Institute, Chinese Academy of Fishery Sciences. After 7-day acclimation, wild caught fish start actively feeding. The water temperature was controlled at 30.6 ± 0.5°C, and the photoperiod was maintained at 14 h light and 10 h dark through an incandescent lamp at 2000 lux, dissolved oxygen >5.27 mg/L, ammonia nitrogen <0.1 mg/L, pH 7.57 ± 0.12, salinity 33‰. Fish were fed once a day from 08:30 to –09:00. The feeding daily ration was 5%∼10% of the fish body weight. The feed was mainly provided with small and wild fishes.
Upon experiment started, the mean body length and wet weight were 239.1 ± 22.1 mm and 310.3 ± 13.8 g, respectively. At the end of this experiment, fish were deprived of feed for 12 h before the sampling was conducted. After the 45-day rearing experiment, six fish from each rearing tank were randomly collected and anesthetized with 0.03% MS-222, and body length and weight were measured. But, three fish from each tank were randomly collected and dissected to obtain the liver, spleen, stomach, intestine and head kidney tissues. Each tissue was stored in 5 mL sterile centrifuge tubes at −80 °C until enzyme activity measurement. At the end of rearing experiment, wild caught fish with similar wet weight (947.2 ± 65.4 g) and length (412.6 ± 27.8 mm) were sampled directly on boat after capture and preserved in liquid nitrogen.
2.2. Enzyme activity measurement
All the tissue samples were partially thawed and homogenized mechanically using a tissue homogenizer on ice. The suspensions were centrifuged according to the requirements of kits and the protein content in the supernatant was determined by BCA Protein Assay kit. The activities of total superoxide dismutase (T-SOD, E.C. 1.15.1.1), catalase (CAT, E.C.1.11.1.6), peroxidase (POD, E.C. 1.11.1.7), lysozyme (LZM, E.C. 3.2.1.17), alkaline phosphatase (AKP, EC 3.1.3.1) and malondialdehyde (MDA) contents in the head kidney and liver were measured. The lysozyme and acid phosphatase (ACP, EC 3.1.3.2) activities in the spleen were determined. The activities of amylase, lipase, trypsin activities in the intestine, and the pepsin activity in the stomach were evaluated. All the assays were determined using commercial kits (Nanjing Jiancheng Bioengineering Institute, Nanjing, China) in triplicates.
2.3. Calculations and statistical analysis
Specific growth rate (SGR) was calculated as follows: SGR = 100 × (Ln(Lf)–ln(Li))/ΔT, where Lf was the final body length; Li was the initial body length; and ΔT was the experimental duration. The data were expressed as the mean ± standard deviation (SD). Statistical analyses were carried out by PASW Statistics (Version 18). Comparisons between different groups were conducted by independent T-test, and significant difference was set at P < 0.05.
2.4. Ethics statement
The experiment was in compliance with the regulations and guidelines established by the Animal Care and Use Committee of South China Sea fisheries Research Institute, Chinese Academy of Fishery Sciences.
3. Results and discussion
After the 45-day experiment, the body length and wet weight were 409.6 ± 0.6 mm and 934.1 ± 124.8 g, respectively. The specific growth rate in total body length was 0.62 ± 0.10 mm day−1, while the specific growth rate in weight was 3.03 ± 0.48 g day−1.
The activities of T-SOD, CAT and POD in the liver for the wild caught tuna were significantly higher than those in the indoor cultured group (P < 0.05, (A–C)). In the cultured group, the AKP activity in the liver was significantly lower than that in the wild caught group (P < 0.05, (D)). On the contrary, the activity of LZM had an opposite tendency with other enzymes (P < 0.05, (E)). In addition, compared to the wild caught group, the MDA content decreased significantly in the cultured group (P < 0.05, (F)). In the spleen, there was no significant difference in the activities of AKP and ACP (P > 0.05, (A,B)).
Figure 1. Activities of total superoxide dismutase (T-SOD), catalase (CAT), peroxidase (POD), lysozyme (LZM), alkaline phosphatase (AKP) and malondialdehyde (MDA) contents in the liver between the wild group and cultured group (W – wild caught group, F – cultured group). Different superscript letters indicate significant differences (P < 0.05) and no superscript letters indicate no significant difference (P > 0.05). Error bars represent standard error.
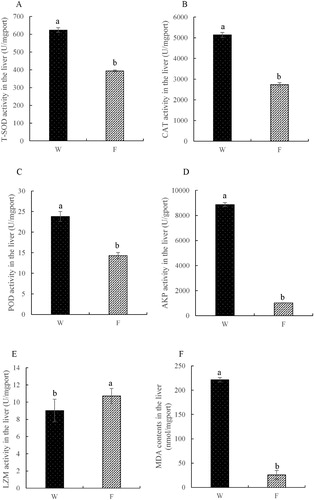
Figure 2. Alkaline phosphatase (AKP) and acid phosphatase (ACP) activities in the spleen (W – wild caught group, F – cultured group).
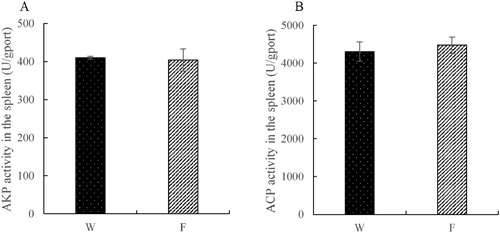
The activities of CAT and AKP in the head kidney in the cultured group were significantly lower than in those in the wild caught group (P < 0.05, (B,D)). However, in the head kidney, the T-SOD and POD activities in the cultured group were significantly increased (P < 0.05, (A,C)). The change of LZM activity in the head kidney was consistent with that in the liver (P < 0.05, (E)). Compared with the wild group, the MDA level was significantly increased in the cultured group (P < 0.05, (F)).
Figure 3. Activities of total superoxide dismutase (T-SOD), catalase (CAT), peroxidase (POD), lysozyme (LZM), alkaline phosphatase (AKP) and malondialdehyde (MDA) contents in the head kidney (W – wild caught group, F – cultured group).
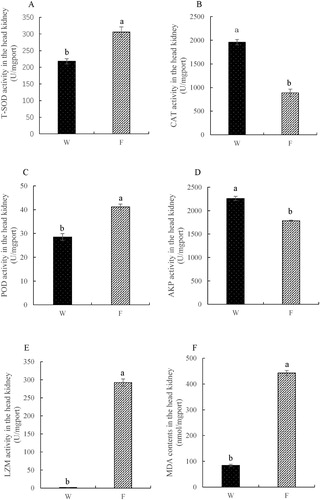
The antioxidant capacity and non-specific immunity play an important role in maintaining the dynamic balance and enhancing the immunity of fish. The activity of antioxidant enzymes and immune-related enzymes can be affected by external factors (Kolayli and Keha Citation1999; Mila-Kierzenkowska et al. Citation2005; Saurabh and Sahoo Citation2008). The SOD, POD and CAT are key enzymes reflecting the antioxidant capacity as they protect cells from damage by excessive reactive oxygen species (ROS), including −OH, O2− and H2O2 (Olsvik et al. Citation2005; Hong et al. Citation2019).
MDA is commonly used as a marker of lipid peroxidation, and its expression level is a valid reflection of the ROS scavenging ability (Draper and Hadley Citation1990; Ozbayer et al. Citation2014; Fu et al. Citation2019). In this experiment, the activities of SOD, POD and CAT in the liver of juvenile mackerel tuna were significantly reduced in fish under captivity, while the content of MDA was also significantly decreased after cultivation. The results of this study were similar to the previous study which showed that a significant depletion was observed in the liver SOD activity in the reared tunas in comparison with wild ones (Ferrante et al. Citation2008). Such a reduced pattern may relate to the cultured environment and feed supplement in the indoor culturing environment. Contrary to the liver, T-SOD and POD activities in the cultured group were significantly increased in the head kidney, but the MDA contents were still increased. This suggests that the antioxidant capacity of fish may be inhibited by the condition of land-based faming.
The innate immune system is an essential part of the defence system and more important than the specific immune system in fish (Kordon et al. Citation2018; Zaibel et al. Citation2019). The LZM is an enzyme with antibiotic properties released by leucocytes and can serve as an indicator for innate immune function (Saurabh and Sahoo Citation2008). After culture for 45 days, the LZM activity in both the liver and head kidney showed a significant increased trend. As for the AKP, its activity was inhibited in the liver and head kidney. The immunity of juvenile mackerel tuna was also significantly affected under the indoor rearing conditions.
In the intestine, the activities of amylase, lipase and trypsin showed the same pattern. In the cultured group, the activities of amylase, lipase and trypsin were significantly higher than those in the wild caught group (P < 0.05, (A–C)). However, the pepsin activity was not significantly different between the wild caught group and cultured group (P > 0.05, (D)).
Figure 4. Activities of amylase, lipase, trypsin in the intestines and the pepsin in the stomach (W – wild caught group, F – cultured group).
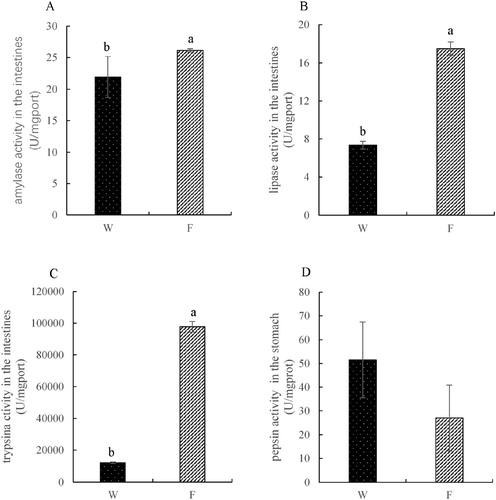
The activities of digestive enzymes, such as protease, lipase and amylase, play a crucial role in the digestive process (Zhao et al. Citation2016). In the present study, digestive enzyme activities between the wild and cultured groups were also significantly different. This may be due to the limited space available in the land-based farming system and inappropriate nutrition. As the indoor cultured fish may use less energy to swim than those in the wild, the activities of amylase, lipase and trypsin in the intestines were increased. Interestingly, the pepsin activity in both wild caught and indoor cultured groups was not significantly different. Owing to the limitation of experimental conditions, there was little evidence for these conjectures and further studies are needed to test this hypothesis.
In general, mackerel tuna in the land-based farming system have increased digestive enzyme activity compared to the wild ones, suggesting the feasibility to maintain growth and fattening of young fish under captivity. However, the tuna in the indoor farming condition show inhibition of antioxidant capacity and an increase in immune enzyme activity. Therefore, there is a need to further improve in the farming environment of mackerel tuna such as optimization of nutritional requirement. The present study provides physiological evidence towards understanding key obstacles for domestication of wild mackerel tuna and its artificial breeding under captivity.
Disclosure statement
No potential conflict of interest was reported by the author(s).
Additional information
Funding
References
- Benetti DD, Partridge GJ, Stieglitz J. 2016. Overview on status and technological advances in tuna aquaculture around the world. In: Benetti DD, Partridge GJ, Buentello A, editors. Advances in tuna aquaculture. Amsterdam: Elsevier; p. 1–19
- Block BA, Dewar H, Blackwell SB, Williams TD, Prince ED, Farwell CJ, Boustany A, Teo SL, Seitz A, Walli A, Fudge D. 2001. Migratory movements, depth preferences, and thermal biology of Atlantic bluefin tuna. Science. 293(5533):1310–1314. doi:10.1126/science.1061197.
- Bojolly D, Doyen P, Le Fur B, Christaki U, Verrez-Bagnis V, Grard T. 2017. Development of a qPCR Method for the identification and Quantification of Two Closely related tuna species, Bigeye tuna (Thunnus obesus) and yellowfin tuna (Thunnus albacares), in Canned tuna. J Agric Food Chem. 65(4):913–920. doi:10.1021/acs.jafc.6b04713.
- Buentello A, Seoka M, Kato K, Partridge GJ. 2016. Tuna farming in Japan and Mexico. In: Benetti DD, Partridge GJ, Buentello A, editors. Advances in tuna Aquaculture. Amsterdam: Elsevier; p. 189–215.
- Castrillon AM, Navarro MP, GarciaArias MT. 1996. Tuna protein nutritional quality changes after canning. J Food Sci. 61(6):1250–1253. doi:10.1111/j.1365-2621.1996.tb10972.x.
- Chen BN, Hutchinson W, Foster C. 2016. Southern bluefin tuna captive breeding in Australia. In: Benetti DD, Partridge GJ, Buentello A, editors. Advances in tuna Aquaculture. Amsterdam: Elsevier; p. 233–252.
- Die DJ. 2016. Challenges faced by management of the Atlantic bluefin tuna stock related to the development of Mediterranean bluefin tuna farming. In: Benetti DD, Partridge GJ, Buentello A, editors. Advances in tuna Aquaculture. Amsterdam: Elsevier; p. 43–58.
- Draper HH, Hadley M. 1990. Malondialdehyde determination as index of lipid peroxidation. Methods Enzymol. 186:421–431.
- Ferrante I, Ricci R, Aleo E, Passi S, Cataudella S. 2008. Can enzymatic antioxidant defences in liver discriminate between wild and sea cage-reared Bluefin Tuna quality? Aquaculture. 279(1–4):182–187. doi:10.1016/j.aquaculture.2008.03.035.
- Fu ZY, Yang R, Chen X, Qin JG, Gu ZF, Ma ZH. 2019. Dietary non-protein energy source regulates antioxidant status and immune response of barramundi (Lates calcarifer). Fish Shellfish Immunol. 95:697–704. doi:10.1016/j.fsi.2019.11.018.
- Graham BS, Grubbs D, Holland K, Popp BN. 2007. A rapid ontogenetic shift in the diet of juvenile yellowfin tuna from Hawaii. Mar Biol. 150(4):647–658. doi:10.1007/s00227-006-0360-y.
- Hong J, Chen X, Liu S, Fu Z, Han M, Wang Y, Gu Z, Ma Z. 2019. Impact of fish density on water quality and physiological response of golden pompano (Trachinotus ovatus) flingerlings during transportation. Aquaculture. 507:260–265. doi:10.1016/j.aquaculture.2019.04.040.
- Kolayli S, Keha E. 1999. A comparative study of antioxidant enzyme activities in freshwater and seawater-adapted rainbow trout. J Biochem Mol Toxicol. 13(6):334–337. doi:10.1002/(sici)1099-0461(1999)13:6<334::Aid-jbt7>3.0.Co;2-m.
- Kordon AO, Karsi A, Pinchuk L. 2018. Innate immune responses in fish: antigen presenting cells and professional phagocytes. Turkish J Fish Aquat Sci. 18(9):1123–1139. doi:10.4194/1303-2712-v18_9_11.
- Metian M, Pouil S, Boustany A, Troell M. 2014. Farming of bluefin tuna-reconsidering global estimates and sustainability concerns. Rev Fish Sci Aquaculture. 22(3):184–192. doi:10.1080/23308249.2014.907771.
- Mila-Kierzenkowska C, Woźniak A, Woźniak B, Drewa G, Chęsy B, Drewa T, Krzyżyńska-Malinowska E, Ceraficki R. 2005. Activity of superoxide dismutase (SOD) and concentration of thiobarbituric acid reactive substances (TBARS) in liver and muscles of some fish. Acta Biol Hung. 56(3–4):399–401. doi:10.1556/ABiol.56.2005.3-4.21.
- Mylonas CC, De La Gandara F, Corriero A, Rios AB. 2010. Atlantic bluefin tuna (Thunnus thynnus) farming and fattening in the Mediterranean Sea. Rev Fish Sci. 18(3):266–280. doi:10.1080/10641262.2010.509520.
- Olsvik PA, Lie KK, Jordal AEO, Nilsen TO, Hordvik I. 2005. Evaluation of potential reference genes in real-time RT-PCR studies of Atlantic salmon. BMC Mol Biol. 6:9. doi:10.1186/1471-2199-6-21.
- Ozbayer C, Kurt H, Ozdemir Z, Tuncel T, Saadat SM, Burukoglu D, Senturk H, Degirmenci I, Gunes HV. 2014. Gastroprotective, cytoprotective and antioxidant effects of Oleum cinnamomi on ethanol induced damage. Cytotechnology. 66(3):431–441. doi:10.1007/s10616-013-9594-y.
- Peng S, Wang L, Wang Y, Zheng H, Wang L, Min M, Shi Z. 2019. Research progress on artificial culture and breeding of tuna in the World. Open J Fish Res. 6(03):118. doi:10.12677/ojfr.2019.63015.
- Rooker JR, Alvarado Bremer JR, Block BA, Dewar H, De Metrio G, Corriero A, Kraus RT, Prince ED, Rodríguez-Marín E, Secor DH. 2007. Life history and stock structure of Atlantic bluefin tuna (Thunnus thynnus). Rev Fish Sci. 15(4):265–310. doi:10.1080/10641260701484135.
- Saurabh S, Sahoo PK. 2008. Lysozyme: an important defence molecule of fish innate immune system. Aquac Res. 39(3):223–239. doi:10.1111/j.1365-2109.2007.01883.x.
- Zaibel I, Appelbaum Y, Arnon S, Britzi M, Schwartsburd F, Snyder S, Zilberg D. 2019. The effect of tertiary treated wastewater on fish growth and health: laboratory-scale experiment with Poecilia reticulata (guppy). Plos One. 14(6):25. doi:10.1371/journal.pone.0217927.
- Zhao ZX, Song CY, Xie J, Ge XP, Liu B, Xia SL, Yang S, Wang Q, Zhu SH. 2016. Effects of fish meal replacement by soybean peptide on growth performance, digestive enzyme activities, and immune responses of yellow catfish Pelteobagrus fulvidraco. Fish Sci. 82(4):665–673. doi:10.1007/s12562-016-0996-6.