ABSTRACT
Artificial insemination is the foundation of Brazilian intensive pig farming and the semen quality is a key point for the success of the productive chain. Several bacteria have already been reported as semen contaminants and may cause morphological and functional changes in sperm, decreasing male fertility, in addition to predisposing females to reproductive failures. The objective of this study was to perform a bacteriological examination of boar semen (Sus scrofa) obtained from an Artificial Insemination Centre in the southwestern region of Paraná. One hundred semen samples were assessed for volume, colour, smell, motility, agglutinated sperm cells and for bacterial contamination. Bacterial species were further identified by matrix-assisted laser desorption/ionization time-of-flight (MALDI-TOF) mass spectrometry. The results revealed positive bacterial isolation in 43% of the samples, with predominance of Gram-negative pathogens. The identified species were: Pseudomonas aeruginosa, Proteus mirabilis, Escherichia coli, Kerstersia gyiorum, Aerococcus viridans, Brevibacterium casei, Providencia stuartii, Citrobacter koseri and Staphylococcus pasteuri. The E. coli contamination was associated with decreased sperm motility (p < 0.01) and vigour (p = 0.002). Despite the frequent usage, the antimicrobial resistance tests showed that few isolates were resistant to gentamycin or neomycin, drugs that are commonly used to extend semen viability in Brazil.
1. Introduction
Currently, artificial insemination (AI) is the technology of reproduction most used in swine breeding farms (Knox Citation2016). While trading of seminal doses for AI has become a key factor in the exchange of genetic potential, several hygienic precautionary measures must be taken to avoid disease transmission between farms or even countries (Kuster and Althouse Citation2016).
Contamination can occur as a result of the infection of male reproductive tract, but also through semen collection and processing to obtain seminal doses (Úbeda et al. Citation2013; Kuster and Althouse Citation2016; Santos and Silva Citation2020). In boar semen, bacteria belonging to at least 25 different genera have been detected as contaminants, with the most frequently occurring ones being Escherichia, Pseudomonas, Staphylococcus and Proteus (Althouse and Lu Citation2005).
Several studies have shown that bacteria impair sperm quality by altering the structure of the sperm, by affecting its motility or sperm agglutination, and membrane integrity, or by provoking a premature acrosome reaction (Diemer et al. Citation1996; Althouse et al. Citation2000; Oberlender et al. Citation2013; Sepúlveda et al. Citation2014; Chavez Citation2016).
The importance of the pig industry and the necessity of maintaining good hygienic status in pig semen require detailed knowledge of the bacterial microbiota in boar semen and their resistance profile. Therefore, the objectives of the present study were to perform bacteriological examination and quality analysis of boar semen samples, obtained from an artificial insemination centre, using MALDI-TOF (Matrix-Assisted Laser Desorption/ Ionization – Time of Flight mass spectrometry) for strains identification and further determine their resistance profile.
2. Materials and methods
2.1. Origin of samples
Semen samples were collected from 100 boars, ranging in age from 12 to 36 months. The animals were of proven fertility and originated from commercial lineage, belonging to an artificial insemination centre in the state of Paraná, Brazil. The centre produces an average of 5500 semen doses per month that are sent to several swine herds located around the state. The herd meets the requirements of biosecurity contained in Normative Instruction/SDA number 19 (Brazil – MAPA). The study was approved by the Ethics Committee under protocol number 1875170317.
2.2. Sample collection
The collection of ejaculates was performed using the gloved-hand technique (Hancock and Howell Citation1959). Prior to collection, sanitization of the preputial area was carried out by manual pressure of the prepuce in the direction of the preputial opening. The pre-sperm fraction of the raw ejaculations was discarded to maintain only the sample’s sperm-rich fractions. An aliquot of 100 μL of each semen sample in natura were added to sterile microtubes containing 2 mL of Stuart transport medium (sodium glycerophosphate 10 g/l, sodium thioglycolate 1.0 g/l, calcium chloride 0.1 g/l, methylene blue 2.0 mg/l, agar 3.0 g/L). The remainder was used for the preparation of the insemination doses.
2.3. Semen evaluation
For each semen sample, the volume, colour and smell were evaluated. Colour alterations were assessed visually by observing the semen in a transparent flask in search specifically for mucous streaks (that can compromise motility and agglutination analysis) and blood presence. Smell was evaluated directly on the top of the flask (typical, no smell, urine smell, putrid, or other) and putrid odour was a criterion for sample exclusion. Immediately after semen collection, the concentration of sperm cells and their motility were evaluated. The concentration of sperm cells was determined using the standard Neubauer haemocytometer and observations were made with a light microscope (400X). Sperm vigour and motility were determined by microscopic observation of diluted semen samples. Motility results are expressed as percentage of cells displaying forward motility (the average of five different fields). A subjective score (0 – immovable to 5 – higher velocity) was applied to evaluate the spermatic vigour. Morphological characteristics of spermatozoa were evaluated using wet chamber technique. For this, semen was diluted and fixed in pre-heated, buffered saline formol at 37°C, and an aliquot was evaluated under differential interference contrast microscope (1000×). Sperm cells with head or tail deformations or showing the presence of cytoplasmic droplets were considered as abnormal. The agglutination of sperm cells (the adherence of exclusively motile sperms to each other) was assessed in the semen samples using a light microscope and the extent of agglutination was scored as 0 (no agglutination), 1 (5–10% of cells agglutinated), 2 (10–15% agglutinated) and 3 (15–23% agglutinated). Clumping of non-moving sperm cells was not considered as a sign of sperm agglutination (Martín et al. Citation2010).
2.4. Bacterial isolation
Culture was performed inoculating aliquots of semen samples in BHI broth (Brain Heart Infusion – Difco), incubated for 24 h at 37°C. The isolation was performed in trypticase soy agar (TSA) supplemented with 5% of sheep blood and MacConkey agar, with incubation at 37°C for 24 h in aerobiosis.
2.5. Bacterial identification
The isolated colonies were identified by matrix-assisted laser desorption ionization–time of flight mass spectrometry (MALDI-TOF MS). The MALDI-TOF MS sample preparation, data processing and analysis were done as previously described by Hijazin et al. (Citation2012). Mass spectra were acquired using a Microflex™ mass spectrometer (Bruker) and identified with manufacturer’s software MALDI BioTyper™ 3.0. Standard Bruker interpretative criteria were applied; scores ≥ 2.0 were accepted for species assignment and scores ≥ 1.7 but ≤ 2.0 for genus identification.
2.6. Escherichia coli genotypic profiling
The identified Escherichia coli isolates were cultured overnight in BHI (Difco-BBL, Detroit, MI, U.S.A.) at 37°C and DNA was purified as previously described (Boom et al. Citation1990). Briefly, 200 µl of cultured broth were added to 1ml of lysis buffer (120 g guanidium thiocyanate, 10 mL Tris-HCl 1 M [pH 6.4], 8.8 ml EDTA 0.5 M [pH 8], 1 ml Triton 100×, 100 mL ultrapure H2O) and 40 μL diatom suspension (1 g diatom, 50 μL HCl 37%, 5 mL ultrapure H2O), agitated and incubated at room temperature for 20 min. The suspension was centrifuged at 12,800× rpm for 1.5 min; the resulting pellet was washed twice with washing buffer (120 g guanidium thiocyanate, 10 mL Tris-HCl 1 M [pH 6.4], 100 mL ultrapure H2O), twice with ethanol 70% (−20°C) and once with acetone. Pellets were dried at 56°C, resuspended in elution buffer (1 mL Tris-HCl 1 M [pH 6.4], 0.2 mL EDTA 0.5 M [pH 8], 100 mL ultrapure H2O) and further incubated at 56°C for 10 min. The suspension was centrifuged at 12,800× rpm for 7 min and supernatant (nucleic acid) was stored at −20°C.
The strains were assigned to phylogenetic groups (A, B1, B2, C, D, E, F, and Escherichia cryptic clade I) according to Clermont et al. (Citation2013) protocol based on the presence of chuA, yjaA and arpA genes and the TSPE4.C2 fragment. The identified E. coli isolates were further characterized for virulence profiling. A PCR and a set of three multiplex reactions were performed to assess the traditionally recognized virulence markers: pap (pilus associated with pyelonephritis – P fimbriae), tsh (temperature-sensitive hemagglutinin), iss (increased serum survival), sfa (S fimbriae), cnf (cytotoxic necrotizing factor), hly (α-Hemolysin) and aer (aerobactin).
The PCR reactions contained 20 pmoles of each primer (Invitrogen Corporation, Carlsbad, CA, U.S.A.), 1.5 mM MgCl2, 200 mM of dNTP, 1 U of Taq DNA polymerase (Fermentas Inc., Glen Burnie, MD, U.S.A.), 1X PCR buffer and ultra-pure water. The amplification cycle consisted of 94°C for 5 min followed by 30 cycles of denaturation (30 sec at 94°C), annealing (55°C for 30 sec), extension (72°C for 30 sec) and 7 min at 72°C for final extension. The amplified products were separated by electrophoresis in agarose gel (1.5%), stained with SYBR Safe (Invitrogen®), and identified through 100 bp DNA ladder (LGC Biotecnologia, São Paulo, Brazil).
2.7. Antimicrobial susceptibility test
Susceptibility profiles were determined using disc diffusion method according to the CLSI (Clinical and Laboratory Standards Institute) protocol (CLSI Citation2015). The antimicrobial agents tested included amoxicillin (10 µg), ceftiofur (30 µg), florfenicol (30 µg), lincomycin (2 µg), fosfomycin (300 µg), enrofloxacin (5µg), ciprofloxacin (5µg), marbofloxacin (5 µg), sulfisoxazole (300µg), trimethoprim-sulfamethoxazole (1.25/23.75µg), tetracycline (30 µg), doxycycline (30 µg), gentamycin (10µg), neomycin (30 µg), spectinomycin (100µg) and streptomycin (10µg).
The strain Escherichia coli ATCC 25922 was used as quality control. The interpretative breakpoints were obtained in the supplements VET01S (CLSI Citation2017), VET06 (CLSI Citation2009a) and M100-S19 (CLSI Citation2009b).
2.8. Statistical analyses
The data were processed using SPSS 16.0 (SPSS Inc) and the level of significance of p ≤ 0.05 was adopted. The Mann–Whitney and Kruskal–Wallis tests were applied to assess difference in semen concentration and motility in regard to bacterial isolation and E. coli identification. The Fisher–Freeman–Halton test was employed to assess the association between the bacterial isolation and E. coli identification with spermatic vigour.
The cluster analyses for antimicrobial resistance and E. coli virulence profiles were performed with Bionumerics 7.6 (Applied Maths NV, Sint-Martens-Latem, Belgium). Profiles were analysed as categorical data using different values coefficient and Ward method. Multiresistance was determined according to Schwarz et al. (Citation2010).
3. Results
No alterations regarding colour and smell were identified among studied samples, and therefore no sample was discarded. The observed mean concentration and motility were: 376.8 ± 137.5 (× 106 mL−1) and 92.9 ± 7.5 (%), respectively. The sperm vigour classification is presented in . No agglutination was detected among the evaluated samples, and only six of these presented alterations in sperm morphology (mostly acrosome defects, and head and tail abnormalities).
Table 1. Sperm vigour classification of boar semen samples in regard to bacterial isolation and E. coli identification – N (%).
A total of 43 semen samples (43.0%) were positive for bacterial isolation. There was no effect of bacterial contamination on semen concentration (p = 0.088), although it significantly decreased motility (p = 0.003). The spermatic vigour also presented different proportions among contaminated samples (p = 0.05) ().
Among the semen samples positive for bacterial isolation, only 16.3% (7/43) presented mixed contamination (more than one bacterial species isolated). Ten different bacterial genera were identified and only three of these were Gram-positive; a total of 50 strains were isolated from the 43 positive semen samples. The identified species and mixed contamination profiles are described in . Pseudomonas aeruginosa, E. coli and Proteus mirabilis stand out with over 65% of the identified single contamination. Only four mixed contamination profiles were identified and, interestingly, E. coli was present in only one of them ().
Table 2. Bacterial species identified from boar semen samples isolated in pure culture or in mixed cultures as described.
It is noteworthy that E. coli contamination resulted in a drastic effect on sperm motility (p < 0.01) and vigour (p = 0.002). Interestingly, the six samples that presented alterations in sperm morphology were also contaminated with E. coli. The 10 E. coli strains isolated from the positive animals were further genotypically characterized. Regarding the phylogenetic classification, 7/10 isolates were classified as phylogenetic group B2, 2/10 as B1 and 1/10 as group A. The virulence profiling resulted in four profiles (P1 to P4) () in which all isolates were sfa+/cnf+ and negative for the aer gene. No association was observed between virulence profiles and the isolates phylogenetic classification and infection type.
The antimicrobial susceptibility characterization was performed in all 50 bacterial isolates obtained (). The resistance profiles cluster analysis resulted in two main groups – A and B (). Group A comprised 17 isolates with heterogeneous resistance profiles, including 8/10 E. coli; these were resistant to one or up to six antimicrobial classes with 58.8% (10/17) of the isolates classified as multiresistant. The remaining 33 isolates comprised group B that is characterized by resistance to over five antimicrobial classes. Group B may be further divided in two clusters – B1 and B2, where the B1 cluster presented the majority of the Pseudomonas isolates and was characterized by resistance to five or up to seven antimicrobial classes, while the B2 cluster comprised most of the Proteus isolates resistant to seven or eight antimicrobial classes. The main difference observed between B1 and B2 clusters is due to fluoroquinolones resistance.
Figure 2. Resistance profiles cluster analysis of isolated strains. The grey scale (black, grey and white) corresponds to resistance, intermediate and sensitivity to antimicrobials, respectively. The coloured squares indicate the identified resistance groups (blue – A, orange – B1, red – B2).
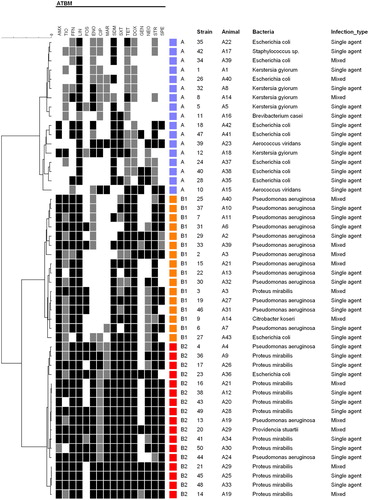
Table 3. Number of strains from different species resistant to the antimicrobial tested using disk diffusion method.
4. Discussion
The bacteria usually present in the reproductive microbiota of boars can be found in the ejaculate after collection. Althouse and Lu (Citation2005) described that bacterial strains belonging to 25 different genera have already been detected as semen contaminants, with the most frequent being Escherichia, Pseudomonas, Staphylococcus and Proteus.
Here we detected 43% of the semen samples positive for bacterial isolation with predominance of Pseudomonas aeruginosa (25.6%), Proteus mirabilis (20.9%) and Escherichia coli (18.6%) in single contamination. Bennemann et al. (Citation2018), in contrast, reported even higher bacterial contamination rate. From 336 semen samples, the authors observed that 86% were contaminated with at least two different microorganisms; and the most frequent species among raw semen samples were Staphylococcus hyicus, Escherichia coli and Alcaligenes faecalis.
Our results also differ from the findings of Martín et al. (Citation2010) that reported E. coli (79%) as the most frequently found microorganism in healthy swine ejaculate, followed by Proteus spp. (36%), Staphylococcus spp. (12%), Streptococcus spp. (9%) and Pseudomonas spp. (8%). In addition, even though Morrell et al. (Citation2019) reported the identification of E. coli, Proteus spp., Staphylococcus spp. and Citrobacter spp. in their control samples, which are in agreement with our findings, the Pseudomonas genus was not detected among their evaluated samples.
Pseudomonas aeruginosa can cause damage to the spermatozoa, not only by direct contact, through the fimbriae, but also because of the large number of extracellular factors, such as proteases and phospholipases, which directly affect the extracellular matrix of sperm membrane (Ben Haj Khalifa et al. Citation2011). Sepúlveda et al. (Citation2014) evaluated the spermicide effects of different concentrations of Pseudomonas aeruginosa and reported reduction of motility and viability and integrity of the spermatozoa acrosome.
Similarly, the Proteus genus has also been related with the reduction of semen quality (Yániz et al. Citation2010). Chavez (Citation2016) observed high frequency of Proteus mirabilis in Brazilian boar semen (96%), which was also presented a positive correlation with the total sperm morphological changes detected.
Although E. coli is classified as a commensal bacterium of the male reproductive system, some pathogenic strains can induce sperm agglutination and, consequently, a decrease of motility, and also a high frequency of morphologically abnormal spermatozoa or single defect that may reduce fertility (Bussalleu et al. Citation2011; Pinart et al. Citation2017; Bonet et al. Citation2018). In this study, the sperm motility and vigour were significantly affected by E. coli contamination. This can be attributed to the action of bacterial toxins (Sone et al. Citation1982), pH change, substrate competition (Rideout et al. Citation1982), or by direct bacterial action, which will lead to structural defects in the sperm cell membrane (Diemer et al. Citation1996).
Althouse et al. (Citation2000) correlated the presence of bacteria in the semen with significant reduction of sperm motility (usually greater than 30%), sperm agglutination, high rate of changes in acrosome (values greater than 20%) and viability of sperm cells after 24–36 h of collection and processing (regardless of diluent to be of short, medium or long-term) and also the acidification of the medium (pH between 5.7 and 6.4). These deleterious effects associated with the presence of bacteria in the ejaculate also appear to be dose dependent, i.e. the higher the contamination, the worse the seminal quality (Althouse et al. Citation2000; Oberlender et al. Citation2013).
In this study, it was not possible to apply the computer-assisted sperm analysis (CASA) system for the evaluation of sperm motility. The CASA system enables to assess sperm motility in an objective manner, including the evaluation of sperm cells speed and behaviour, and so evaluate the fertilizing ability of the ejaculations (Broekhuijse et al. Citation2012). Therefore, this technique could further enhance the motility results of the present study. Nevertheless, the traditional motility assessment (visual with a light microscope), which is practical and simple, still proved to be a useful technique in this study.
It is noteworthy that E. coli contamination also results in alterations in sperm morphology. In the present study, acrosome and tail abnormalities were only detected in the samples positive for E. coli contamination. The species has already been described to induce disturbances in the acrosome integrity of liquid-stored spermatozoa. However, this effect does not appear to be specific to E. coli, since it has also been described in boar semen samples contaminated with other Enterobacteriaceae members, such as Enterobacter cloacae (Prieto-Martínez et al. Citation2014) and Pseudomonas aeruginosa (Sepúlveda et al. Citation2014).
Contamination by E. coli can also cause pregnancy interruption, leading to endometrial lesions and litter size reduction (Mobley et al. Citation1994). Foetal mummification or abortion may also occur due to septicemia, toxemia and pyrexia (Gresham Citation2003). In addition, infection before 35 days of gestation can lead to embryonic or foetal death, resulting in reabsorption, maceration, and abortion (Vanroose et al. Citation2000). Martín et al. (Citation2010) reported a reduction in the number of piglets from AI with seminal samples with increasing concentrations of E. coli.
The urinary tract is among the most common sites of bacterial infection and E. coli is by far the most common infecting agent. E. coli from a small number of O serogroups (six O groups cause 75% of UTIs) have phenotypes that are epidemiologically associated with cystitis and acute pyelonephritis in the normal urinary tract, which include expression of P fimbriae, haemolysin, aerobactin, serum resistance and encapsulation (Manges et al. Citation2001). Specific adhesins, including P (pap), type 1 and other fimbriae, seem to aid in colonization (Nowicki et al. Citation1989). Several toxins are produced, including haemolysin, cytotoxic necrotizing factor and an autotransporter protease known as Sat. These virulence factors are found in differing percentages among various subgroups of uropathogenic E. coli (Johnson and Stell Citation2000).
In this study, the isolated E. coli were positive for at least three of the seven virulence genes screened. The sfa and cnf genes were detected in all E. coli isolates; these genes are commonly found in uropathogenic Escherichia coli isolated from humans (Johnson et al. Citation2003) and pigs (Ding et al. Citation2012). Six strains were further positive for tsh and iss that have been described in septicemic E. coli in human and poultry, suggesting that these strains may present a more invasive characteristic. The remaining strains were positive for pap that has already been associated with swine bacteriuria and pyelonephritis (Krag et al. Citation2009). These results suggest that this contaminated semen can cause cystitis in sows as well as prostatitis (Rippere-Lampe et al. Citation2001).
The Kerstersia gyiorum has not been isolated from swine semen before, but it has been associated with human infection (Pence et al. Citation2013). With the emergence of MALDI-TOF MS for microbial identification from clinical specimens, the reported frequency of this microorganism and other species may increase in the future. The MALDI-TOF MS is a high-throughput, highly accurate, and relatively cost-effective technique which is being extensively employed for routine clinical microbiology. The application of MALDI-TOF MS, with more comprehensive databases, for bacterial identification enables the detection of new and atypical taxa from different clinical specimens; and the more frequent identification of these isolates may help to understand their distribution and epidemiological context.
Semen contamination becomes relevant when it is associated with reduction of male fertility or with the quality of the inseminating dose. Microbial contamination may arise from animal infection (systemic and/or reproductive) or during ejaculation when semen has contact with the reproductive tract microbiota. Also environmental contamination may occur during semen processing considering the possibility of contaminated materials, equipment and solvents, as well as inadequate semen conditioning (Oberlender et al. Citation2013; Kuster and Althouse Citation2016).
Goldberg et al. (Citation2013) assessed the risk factors for microbiological contamination of semen and reported that the prolonged collection time (6–7 min) and dripping of the preputial liquid inside the collection cup were responsible for significantly higher levels of contamination compared to the other evaluated variables, including hygiene of preputial ostium and collection glove, and even the size of preputial diverticulum. The same authors indicated that contamination can be avoided with planning and strategic training and recycling of the collaborators in the reproduction centres.
Furthermore, the bacterial contamination of the sow reproductive tract, instigated by AI, can cause return to the oestrus, vulvar discharges, reduction of litter size, and increase the number of stillbirths and mummies. It can also be related to the birth of weak piglets, which will directly affect productivity, as well as lead to a possible discard of the female (Prieto and Castro Citation2005).
The antimicrobials usage in semen diluents aims to reduce the bacterial contamination; however, it has already been shown that 90% of the bacteria isolated from doses of swine semen present some level of antimicrobial resistance (Bolarín Citation2011). In this study, all isolates analysed presented resistance phenotype against at least one of the antimicrobial classes tested.
The majority of the E. coli isolated in this study had a low resistance rate against the tested antimicrobials, and only two isolates were classified in group B1 and B2 (). What stands out here is the multiresistance profile of the Pseudomonas aeruginosa and Proteus mirabilis isolates. All Proteus mirabilis strains analysed showed resistance to streptomycin, spectinomycin, lincomycin and florfenicol (, ). The multidrug resistance pattern presented by Pseudomonas aeruginosa may be explained by the presence of efflux pump systems conferring resistance to sulfonamides, trimethoprim, tetracycline, chloramphenicol, quinolones and some β-lactams, due to lack of uptake resulting from inability of antibiotics to achieve effective intracellular, which extrude drugs having the potential to damage the cell (Paulsen et al. Citation1996).
The diluent used at the artificial insemination centre examined contain gentamycin (80 mg/mL); considering that from the 50 studied isolates only 13 were resistant to gentamycin (), this still is an effective antibiotic to be added in the diluent. This result corroborates Bennemann et al. (Citation2018) findings that reported over 80% of gentamycin susceptibility. However, Althouse et al. (Citation2000) described in their study that most of the bacteria isolated in the inseminating doses were resistant to gentamycin. According to Scheid (Citation2000) and Santos and Silva (Citation2020), it is essential to maintain a strict hygienic standard and to add antibiotics to the semen diluents. Gentamycin, penicillin, streptomycin, neomycin and lincomycin are the most commonly used antimicrobials. Nevertheless, not all genera isolated in this study were sensitive to these antibiotics (), which can be considered a problem in obtaining inseminating doses free from bacterial contamination. The emergence of resistant bacteria in boar semen may be further aggravated considering the lack of specific governmental directives for the addition of antibiotics in boar semen diluents.
5. Conclusion
In this study, 43% of the evaluated semen samples were positive bacterial isolation. It was observed a predominance of Gram-negative bacteria in the contaminated samples, with Pseudomonas aeruginosa, E. coli and Proteus mirabilis standing out with over 65% of the identified single contamination. It is highlighted that 86.0% of the isolated strains were classified as multidrug resistant; however, low resistance to gentamycin and neomycin, that are common antibiotics in boar semen extenders, was observed. Therefore, although antimicrobials are routinely added to the seminal ejaculate to control contaminant bacteria that does not mean in any way that the hygiene measures related to the facilities, housing, animal care, collection processing, storage and distribution of semen may be neglected.
Disclosure statement
No potential conflict of interest was reported by the author(s).
Data availability statement
The data that support the findings of this study are available from the corresponding author upon reasonable request.
Additional information
Funding
References
- Althouse GC, Kuster CE, Clark SG, Weisiger RM. 2000. Field investigations of bacterial contaminants and their effects on extended porcine semen. Theriogenology. 53(5):1167–1176.
- Althouse GC, Lu KG. 2005. Bacteriospermia in extended porcine semen. Theriogenology. 63(2):573–584.
- Ben Haj Khalifa A, Moissenet D, Vu Thien H, Khedher M. 2011. Virulence factors in Pseudomonas aeruginosa: mechanisms and modes of regulation. Ann Biol Clin. 69(4):393–403.
- Bennemann PE, Machado SA, Girardini LK, Sonálio K, Tonin AA. 2018. Bacterial contaminants and antimicrobial susceptibility profile of boar semen in southern Brazil Studs. Revista MVZ Córdoba. 23(2):6637–6648.
- Bolarín A. 2011. Bacteriología en semen de porcino. Avances en Tecnología Porcina. 8(79):20–30.
- Bonet S, Delgado-Bermúdez A, Yeste M, Pinart E. 2018. Study of boar sperm interaction with Escherichia coli and Clostridium perfringens in refrigerated semen. Anim Reprod Sci. 197:134–144.
- Boom R., Sol C. J., Salimans M. M., Jansen C. L., Wertheim-Van Dillen P. M., Van Der Noordaa J. 1990. Rapid and simple method for purification of nucleic acids. J Clin Microbiol. 28(3):495–503.
- Broekhuijse ML, Šoštarić E, Feitsma H, Gadella BM. 2012. Application of computer-assisted semen analysis to explain variations in pig fertility. J Anim Sci. 90(3):779–789.
- Bussalleu E, Yeste M, Sepúlveda L, Torner E, Pinart E, Bonet S. 2011. Effects of different concentrations of enterotoxigenic and verotoxigenic E. coli on boar sperm quality. Anim Reprod Sci. 127(3–4):176–182.
- Chavez BR. 2016. População bacteriana no sêmen suíno e seus efeitos sobre a qualidade da dose [unpublished MSc thesis]. Universidade Federal de Lavras.
- Clermont O., Christenson J. K., Denamur E., Gordon D. M. 2013. The Clermont Escherichia coli phylo-typing method revisited: improvement of specificity and detection of new phylo-groups. Environ Microbiol Rep. 5(1):58–65.
- CLSI. 2009a. Methods for antimicrobial susceptibility testing of infrequently isolated or fastidious bacteria isolated from animals. 1st ed. Wayne (PA): CLSI. Supplement VET06.
- CLSI. 2009b. Performance standards for antimicrobial susceptibility testing. 19th ed. Wayne (PA): CLSI. Supplement M100-S19.
- [CLSI] Clinical and Laboratory Standards Institute. 2015. Performance standards for antimicrobial disk and dilution susceptibility tests for bacteria isolated from animals. VET01S. 3th ed. Wayne (PA).
- CLSI. 2017. Performance standards for antimicrobial disk and dilution susceptibility tests for bacteria isolated from animals. 3rd ed. Wayne (PA): CLSI. Supplement VET01S.
- Diemer T, Weidner W, Michelmann HW, Schiefer HG, Rovan E, Mayer F. 1996. Influence of Escherichia coli on motility parameters of human spermatozoa in vitro. Int J Androl. 19(5):271–277.
- Ding Y, Tang X, Lu P, Wu B, Xu Z, Liu W, Zhang R, Bei W, Chen H, Tan C. 2012. Clonal analysis and virulent traits of pathogenic extraintestinal Escherichia coli isolates from swine in China. BMC Vet Res. 8(1):140.
- Goldberg AMG, Argenti LE, Faccin JE, Linck L, Santi M, Bernardi ML, Bortolozzo FP. 2013. Risk factors for bacterial contamination during boar semen collection. Res Vet Sci. 95(2):362–367.
- Gresham A. 2003. Infectious reproductive disease in pigs. In Pract. 25(8):466.
- Hancock JL, Howell GJR. 1959. The collection of boar semen. Veterinary Record. 71:664–665.
- Hijazin M, Alber J, Lämmler C, Weitzel T, Hassan AA, Timke M, Kostrzewa M, Prenger-Berninghoff E, Zschöck M. 2012. Identification of Trueperella (Arcanobacterium) bernardiae by matrix-assisted laser desorption/ionization time-of-flight mass spectrometry analysis and by species-specific PCR. J Med Microbiol. 61(3):457–459.
- Johnson J. R., Kuskowski M. A., Owens K., Gajewski A., Winokur P. L. 2003. Phylogenetic origin and virulence genotype in relation to resistance to fluoroquinolones and/or extended-spectrum cephalosporins and cephamycins among Escherichia coli isolates from animals and humans. J Infect Dis. 188(5):759–768.
- Johnson JR, Stell AL. 2000. Extended virulence genotypes of Escherichia coli strains from patients with urosepsis in relation to phylogeny and host compromise. J Infect Dis. 181(1):261–272.
- Knox RV. 2016. Artificial insemination in pigs today. Theriogenology. 85(1):83–93.
- Krag L, Hancock V, Aalbæk B, Klemm P. 2009. Genotypic and phenotypic characterisation of Escherichia coli strains associated with porcine pyelonephritis. Vet Microbiol. 134(3–4):318–326.
- Kuster CE, Althouse GE. 2016. The impact of bacteriospermia on boar sperm storage and reproductive performance. Theriogenology. 85:21–25.
- Manges AR, Johnson JR, Foxman B, O'bryan TT, Fullerton KE, Riley LW. 2001. Widespread distribution of urinary tract infections caused by a multidrug-resistant Escherichia coli clonal group. N Engl J Med. 345(14):1007–1013.
- Martín LOM, Muñoz EC, De Cupere F, Van Driessche E, Echemendia-Blanco D, Rodríguez JMM, Beeckmans S. 2010. Bacterial contamination of boar semen affects the litter size. Anim Reprod Sci. 120(1–4):95–104.
- Mobley HL, Island MD, Massad G. 1994. Virulence determinants of uropathogenic Escherichia coli and Proteus mirabilis. Kidney Int Suppl. 47:S129–S136.
- Morrell JM, Núñez-González A, Crespo-Félez I, Martínez-Martínez S, Alborcia MM, Fernández-Alegre E, Martínez-Pastor F. 2019. Removal of bacteria from boar semen using a low-density colloid. Theriogenology. 126:272–278.
- Nowicki B, Svanborg-Eden C, Hull R, Hull S. 1989. Molecular analysis and epidemiology of the Dr hemagglutinin of uropathogenic Escherichia coli. Infect Immun. 57(2):446–451.
- Oberlender G, Zangeronimo MG, da Silva AC, de Alcantara Menezes T, Pontelo TP. 2013. Bacteriologia do sêmen suíno–aspectos relacionados: revisão de literatura. Revista Científica Eletrônica de Medicina Veterinária. 20:15.
- Paulsen IT, Brown MH, Skurray RA. 1996. Proton-dependent multidrug efflux systems. Microbiol Mol Biol Rev. 60(4):575–608.
- Pence MA, Sharon J, Tekippe EM, Pakalniskis BL, Ford BA, Burnham CAD. 2013. Two cases of Kerstersia gyiorum isolated from sites of chronic infection. J Clin Microbiol. 51(6):2001–2004.
- Pinart E, Domènech E, Bussalleu E, Yeste M, Bonet S. 2017. A comparative study of the effects of Escherichia coli and Clostridium perfringens upon boar semen preserved in liquid storage. Anim Reprod Sci. 177:65–78.
- Prieto-Martínez N, Bussalleu E, Garcia-Bonavila E, Bonet S, Yeste M. 2014. Effects of Enterobacter cloacae on boar sperm quality during liquid storage at 17 C. Anim Reprod Sci. 148(1-2):72–82.
- Prieto C, Castro JM. 2005. Porcine reproductive and respiratory syndrome virus infection in the boar: a review. Theriogenology. 63:1–16.
- Rideout MI, Burns SJ, Simpson RB. 1982. Influence of bacterial products on the motility of stallion spermatozoa. J Reprod Fertil. 32:35–40.
- Rippere-Lampe KE, Lang M, Ceri H, Olson M, Lockman HA, O'Brien AD. 2001. Cytotoxic necrotizing factor type 1-positive Escherichia coli causes increased inflammation and tissue damage to the prostate in a rat prostatitis model. Infect Immun. 69(10):6515–6519.
- Santos CS, Silva AR. 2020. Current and alternative trends in antibacterial agents used in mammalian semen technology. Animal Reproduction. 17(1):e20190111.
- Scheid RI. 2000. Aspectos de biossegurança e de higiene associados à inseminação artificial em suínos. Encontro Técnico ABRAVES-SC. 1:44–55.
- Schwarz S, Silley P, Simjee S, Woodford N, Van Duijkeren E, Johnson AP, Gaastra W. 2010. Assessing the antimicrobial susceptibility of bacteria obtained from animals. Vet Microbiol. 141:1–4.
- Sepúlveda L, Bussalleu E, Yeste M, Bonet S. 2014. Effects of different concentrations of Pseudomonas aeruginosa on boar sperm quality. Anim Reprod Sci. 150(3–4):96–106.
- Sone M, Ohmura K, Bamba K. 1982. Effects of various antibiotics on the control of bacterial in boar semen. Veterinary Record. 111:11–14.
- Úbeda JL, Ausejo R, Dahmani Y, Falceto MV, Usan A, Malo C, Perez-Martinez FC. 2013. Adverse effects of members of the Enterobacteriaceae family on boar sperm quality. Theriogenology. 80(6):565–570.
- Vanroose G, De Kruif A, Van Soom A. 2000. Embryonic mortality and embryo–pathogen interactions. Anim Reprod Sci. 60:131–143.
- Yániz JL, Marco-Aguado MA, Mateos JA, Santolaria P. 2010. Bacterial contamination of ram semen, antibiotic sensitivities, and effects on sperm quality during storage at 15 C. Anim Reprod Sci. 122(1-2):142–149.