ABSTRACT
To identify the specific biomarkers of exposure of DON in chickens, a toxicokinetic study was performed via oral or intravenous application of deoxynivalenol (DON). Doses of 0.75 and 2.25 mg DON/kg of body weight (BW) were administrated intravenously or orally to the chickens. Next, blood samples were collected at several time points and plasma was obtained. Liquid chromatography–tandem mass-spectrometry (LC–MS/MS) was used to quantify plasma levels of DON and its metabolite DON-3-sulphate (DON-3S). A non-compartmental analysis was performed to study the main toxicokinetic parameters after intravenous or oral application of the toxin. Regarding oral administration, DON plasma level was below the limit of detection (LOD) of the method (1.5 ng/mL) and DON-3S could not be identified. After intravenous administration of DON at 0.75 and 2.25 mg DON/kg BW, the elimination half-life was 57.1 and 47.7 min, respectively, indicating the rapid elimination of DON. The metabolite DON-3S was found in plasma of broilers exposed to DON intravenously. The absence of DON in chicken plasma after oral bolus application suggests the low absorption of this mycotoxin. The presence of DON-3S in plasma indicates that this metabolite could be the appropriate biomarker of DON exposure in chickens.
1. Introduction
The occurrence of mycotoxins in animal feed presents a threat for animal health and can result in important economic loss. Mycotoxin formation subsequent to fungal growth is dependent on several factors which the most important are temperature and water activity (Filtenborg et al. Citation1996). Deoxynivalenol (DON) is a mycotoxin, mainly produced by Fusarium graminearum and Fusarium culmorum that belongs to the trichothecenes family under conditions of high moisture and low temperature (Bertero et al. Citation2018). DON is the most commonly detected mycotoxin in contaminated food and feedstuffs worldwide (Gruber-Dorninger et al. Citation2019). Poultry species are highly exposed to DON mycotoxin. The maximum recommended level of DON in poultry feed was fixed by the European Union in complete feed at 5 mg/kg whilst in pigs was fixed at 0.9 mg/kg (European Commission Citation2006).
In general, poultry species are considered to be tolerant to DON Fusarium mycotoxin, which could be explained by differences in toxicokinetic properties. Indeed, the absorption and distribution of DON by poultry species are limited and its elimination is rapid (Payros et al. Citation2016). With regard to the metabolism, the analysis of suitable metabolites of exposure in blood plasma using validated analytical methods, upon toxicokinetics studies, revealed the formation of DON-3-sulphate (DON-3S) as a metabolite phase II in poultry species (Devreese et al. Citation2015).
At molecular and cellular levels, DON inhibits protein biosynthesis and induces ribotoxic and oxidative stress and apoptosis (Payros et al. Citation2016). The toxic effects of DON are manifested by feed intake reduction, alteration of intestinal functions and immunotoxicity in poultry species (Aguzey et al. Citation2019; Riahi et al. Citation2020, Citation2021; Azizi et al. Citation2021). To more understand the mode of action and to evaluate animal and human health risks, knowledge of the kinetic parameters of toxins is essential. The toxicokinetic studies have to be performed to elucidate the absorption, distribution, metabolism and excretion (ADME) pathways of the target mycotoxin in the target species.
The aim of this study was to assess the toxicokinetics of DON at two concentrations (0.75 and 2.25 mg DON/kg BW), after intravenous or oral bolus administration, in broilers plasma and to determine the most suitable metabolite (s) of exposure of DON.
2. Materials and methods
2.1. Animals and experimental design
A total of 24 one-day-old male Ross 308 broiler chickens were randomly distributed into 6 cages at 4 chickens per cage for 28 days. The experimental model was according to the one described by Osselaere et al. (Citation2013) with minor modifications. The study was approved by the Ethical Committee for Animal Experimentation of IRTA, and carried out in accordance with current regulations on the use and handling of experimental animals (Decree 214/97, Generalitat de Catalunya, Catalonia, Spain). Birds were housed at temperatures of 34°C for the first two days and temperature decreased gradually 3°C per week until reaching 22°C at 28 d. The applied light programme was 24 h of light per day the first two days, 18 h of light per day until 7 days, and 14 h of light per day afterwards. Birds were received water and starter blank feed ad libitum during the whole trial. The diet was formulated according to the nutrient requirements for Ross 308 strain broilers and based on maize and soybean meal (). The DON and aflatoxin B1 (AFB1) contents of the feed provided were evaluated by high performance liquid chromatography (HPLC) method (). The determination of other mycotoxins such as: zearalenone (ZEN), total fumonisins (FBs) and ochratoxin A (OTA) was performed by the Ridascreen® Zearalenon, Ridascreen® Fumonisin and Ridascreen® Ochratoxin A enzyme-linked-immunosorbent assay (ELISA) kits (R-Biopharm), following the manufacturer’s instructions, with detection limits of 1.75, 25 and 2.5 µg/kg for ZEN, FBs and OTA, respectively.
Table 1. The formulation and the proximate analysis of the blank control feed.
At d 28, birds were weighed individually. From all animals, 16 birds were used and divided into 4 groups. DON used in the trial was obtained from Fermentek (Jerusalem, Israel) and was dissolved in acetonitrile (ACN) (Honeywell, Seelze, Germany) to obtain 2.5 mg/mL DON as stock solution. Next, single doses of 0.75 or 2.25 mg DON/kg of BW were intravenously or orally administrated to the chickens (4 birds for each application and for each dose), taking into account that the daily feed intake of broilers was 150 g feed/kg BW. The intravenous injection was applied in the wing vein using a 25G needle (0.5 × 16 mm, Terumo Europe, Leuven, Belgium). After the toxin administration, blood samples were taken in heparinized tubes at different time points at (0) pre-administration and at 3, 6, 10, 15, 30, 60 and 120 min post-administration. Samples were centrifuged at 2851g for 10 min and plasma was stored at −20°C until further analysis.
2.2. Compounds, standards and solutions
DON analytical standard was supplied by Sigma-Aldrich Chemie GmbH (Steinheim, Germany). DON standard was dissolved in ACN as a stock solution (1 mg/mL), and then diluted with HPLC-grade ACN to obtain an individual working standard solution of 5 µg/mL. The stable internal standard (IS) isotope (13C15-DON) was obtained from Biopure (Bioser, Barcelona, Spain) as 1.2 mL of a solution of 25 µg/mL in ACN. An individual working standard solution of 5 µg/mL was prepared by diluting the above stock solution of 13C15-DON with HPLC-grade ACN and was stored at −15°C.
2.3. Instrumentation, materials and reagents
The separation of the analytes was performed on a Zorbax Plus C18 (1.8 μm × 2.1 × 100 mm) column from Agilent (San Jose, CA, USA). Chicken plasma was analysed on a Transcend 600 LC (Thermo Scientific TranscendTM, Thermo Fisher Scientific, San Jose, CA, USA) coupled to an Orbitrap (ExactiveTM, Thermo Fisher Scientific, Bremen, Germany) with an electrospray ionization (ESI) source (HESI-II, Thermo Fisher Scientific, San Jose, CA, USA). Ammonium formate was obtained from Sigma-Aldrich Chemie GmbH (Steinheim, Germany). ACN and Methanol (MeOH) (HPLC gradient grade) were purchased from Honeywell (Seelze, Germany).
2.4. Sample preparation for DON and DON-3s determination in broilers plasma
Preparation of the plasma extract was carried out according to the method of Broekaert et al. (Citation2014). Briefly, for calibrator samples, to 250 µL of blank plasma, 10 µL of a 5 µg/mL internal standard working solution (13C15-DON), 10 µL of a 5 µg/mL of the standard working solution of DON and ACN was added up to a volume of 1 mL.
For incurred samples, to 250 μL of chicken plasma, 10 μL of 13C15-DON IS solution (at 5 µg/mL) and 750 μL of ACN were added. ACN was added to precipitate plasma proteins. The samples were vortexed for 1 min approximately. Afterwards, the samples were centrifuged at 8517g for 10 min. The supernatant (1 mL) was transferred to a new tube and was evaporated to dryness under nitrogen flow over a heating block and reconstituted in 1 mL of ammonium formate 5 mM/MeOH (50:50, v/v) solution, and 10 μL were injected for (HPLC–MS/MS) analysis.
2.5. LC–MS/MS analysis
Gradient elution was established with a mobile phase consisting of 5 mM ammonium formate in water (eluent A) and methanol (eluent B) at a flow rate of 0.2 mL/min. The gradient elution started at 95% B at 1 min and it was decreased to 0% B at 8–12 min afterwards it increased to 95% at 12.5 min and maintained up to 14 min. The column temperature was set at 25°C and the injection volume was 10 µL. MS analyses were performed using selected reaction monitoring (SRM) mode with positive and negative electrospray ionization (ESI±). The settings on the spectrometer were as follows: compounds were ionized by electrospray ionization in the positive and negative mode and measured first in full scan and then in targeted MS/MS mode at a collision energy of 30 eV (both in the range from m/z 50-500). ESI parameters were as follows: spray voltage, 4 kV; sheath gas (N2, >95%), 35 (adimensional); auxiliary gas (N2, >95%), 10 (adimensional); skimmer voltage, 18 V; capillary voltage, 35 V; tube lens voltage, 95 V; heater temperature, 305°C; capillary temperature, 300°C. The capillary and nozzle voltages were 4000 and 95 V ( −95 V in ESI-), respectively. Finally, the data were processed using XcaliburTM version 3.0 (Quanbrowser and Qualbrowser) and Mass FrontierTM 7.0. The retention time and MS/MS parameters are indicated in ().
Table 2. HPLC–MS/MS parameters.
2.6. Toxicokinetic analysis and data evaluation
The toxicokinetic parameters were analysed using non-compartmental analysis. The area under the concentration–time curve from time zero to the last time point (AUC0–t), the area under the concentration–time curve from time zero to infinity (AUC0–∞), maximum plasma concentration (Cmax); elimination rate constant (kel), the half-life of elimination (T1/2el), the volume of distribution (Vd) and clearance (Cl) were determined.
3. Results
After a single oral bolus of DON at 0.75 and 2.25 mg/kg BW, no plasma above the LOQ could be observed for DON and its metabolite DON-3S. Plasmatic DON concentration vs time profiles after intravenous injection of DON at both doses (0.75 and 2.25 mg/kg BW) are shown in . Moreover, from 2 h p.a. no levels above LOQ could be detected for DON ().
Figure 1. Plasma concentration–time profile of DON after a single intravenous administration of DON of 0.75 (n = 5) and 2.25 mg DON/kg (n = 4) BW to broiler chickens.
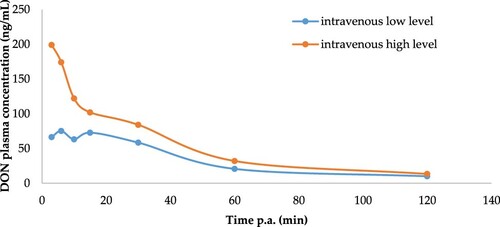
The HPLC–MS/MS coupled with orbitrap analysis revealed the presence of DON-3S in plasma (). Since no commercial standard solution of DON-3S is available, accurate quantitative analysis cannot be performed, and the results were evaluated using the absolute chromatographic peak areas. Average chromatographic peak areas were calculated per treatment and per time ().
Figure 2. Peak area of deoxynivalenol-3-sulphate (DON-3S) at different times after intravenous (IV) administration of 0.75 (n = 5) and 2.25 mg DON/kg (n = 4) BW to broiler chickens.
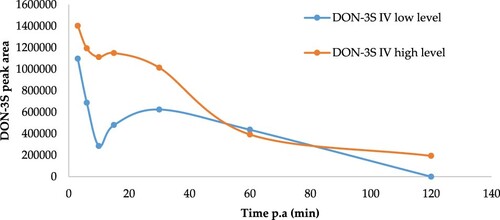
The toxicokinetic analysis of both metabolites was performed based only in data obtained after intravenous administration (). The study of the main toxicokinetic parameters revealed that the AUC0–t and AUC0–∞ after IV administration at 2.25 mg/kg BW were much higher than after IV administration at 2.25 mg/kg BW, which correlates with the doses administered (). The toxin clearance from plasma of broilers intravenously exposed to DON (0.75 or 2.25 mg/kg BW) is rapid (0.16 or 0.33 L/min kg). Furthermore, DON showed a remarkably shorter elimination half-life (T½elimination of 57.1 or 47.7 min) after intravenous injection for both doses.
Table 3. Toxicokinetic parameters of DON and DON-3S after intravenous injection of 0.75 (n = 5) and 2.25 mg DON/kg (n = 4) BW to broiler chickens.
4. Discussion
To understand the sensitivity of broiler chickens to the toxic effects of DON, knowledge of the toxicokinetic properties is necessary. Toxicokinetics deals with what the body does with a DON mycotoxin when given a relatively high dose (2.25 mg/kg BW) relative to the guidance dose (0.75 mg/kg BW). The absorption, distribution, metabolism and elimination termed ADME processes are involved in toxicokinetics.
No detection of DON mycotoxin and its metabolite phase II DON-3S in plasma of birds received the toxin orally even at a high level (2.25 mg/kg BW), presumably due to the low oral bioavailability of this mycotoxin in broilers (Osselaere et al. Citation2013). Prelusky et al. (Citation1986) found low levels of radioactivity in plasma (less than 1% of the dose administrated), when, orally administered 2.2 mg/bird/day of radiolabelled DON (14C), to White Leghorn hens by crop intubation. The absolute oral bioavailability of DON mycotoxin was found to be low and accounts to only 19.3% in broiler chickens, 20.9% in Turkeys and 30.4% in pigeons (Osselaere et al. Citation2013; Devreese et al. Citation2015; Antonissen et al. Citation2016). Furthermore, Broekaert et al. (Citation2014) showed that the absorbed fraction of DON in broiler chickens, after oral DON administration (0.5 mg/kg BW) was only 10.6%, however, in pigs, the absorbed fractions were 100% for DON, which may explain the difference of susceptibility to DON mycotoxicosis between these two species. The concentration of DON in plasma was under LODs for the applied LC–MS/MS method when broilers fed naturally or artificially contaminated diet up to 5 mg DON/kg diet for 21 or 35 d (Dänicke et al. Citation2007; Awad et al. Citation2011; Osselaere et al. Citation2012).
Lun et al. (Citation1988) reported that DON disappeared from the gastrointestinal tract between the crop and the jejunum, and no DON was detected in the blood from the portal vein or heart of laying domestic chickens after intubation with feed containing contaminated corn.
It has been reported that DON has been widely and transiently distributed into different tissues of broiler chickens (plasma, muscle, abdominal fat, stomach, large and small intestine, liver, kidney, heart, lung, skin, spleen, brain, testes, ovary and adrenals) after using oral administration of radio-labelled DON for five days (Wan et al. Citation2014). In the current study, the low Vd may imply the transient distribution of DON in broiler chickens. The transient distribution may be related to the rapid elimination of DON (Wan et al. Citation2014).
In regard to the metabolization of DON in broiler chickens, the biotransformation of DON on DON-3 S has been shown in the current study. Devreese et al. (Citation2015) revealed that after using high-resolution mass spectrometry (HR-MS) analysis of plasma of turkeys and broiler chickens, DON-3S was the major metabolite after oral gavage, as well as after intravenous injection of 0.75 mg/kg BW of DON. In the same way, after a semi-quantitative analysis using HR-MS, it has been seen that DON is metabolized to DON-3S in pigeons after intravenous or oral bolus administration of the parent mycotoxin (Antonissen et al. Citation2016). After a single intra-crop bolus administration of 0.5 mg/kg body weight DON to broiler chickens aged 21 d and weighed 1 kg, DON-3S was detected and no DON was found (Lauwers et al. Citation2019). The maximum peak of the area of DON-3S was achieved in plasma after 30 min post administration (Lauwers et al. Citation2019). According to the recent study of Jurisic et al. (Citation2019), after feeding a dose of 3.8 and 7.5 mg DON /kg of DON feed to laying hens, the analysis of DON in plasma and eggs by LC–MS/MS revealed that DON-3S is the main metabolite, with an average concentration of 6.8 and 10 ng/mL in plasma. Furthermore, the other metabolites, DOM-1, DON-3α-glucuronide, DON-10-sulfonate and DOM-1-10-sulfonate, were only detected at traces or were not detectable in chickens and Turkey poults (Devreese et al. Citation2015; Schwartz-Zimmermann et al. Citation2015).
DON-3S was also detected in excreta after chronic feeding of both levels. Wan et al. (Citation2014) indicated that after the administration of radioactive DON, DON-3S was accounting about 88.6% in excreta of broiler chickens. DON is extensively metabolized to DON-3S in excreta. The conversion of DON to DON-3S could occur in the enterocytes of the intestinal epithelium, in liver and kidney, as hypothesized by Schwartz-Zimmermann et al. Citation2015. These authors found that DON-3S is the major metabolite in excreta of broiler chickens and its biological recovery accounted 80%. Lauwers et al. (Citation2019) found that the maximum peak of area of DON-3S was achieved in dried excreta after 3–6 h post administration.
DON-3S was reported to be a suitable biomarker of DON exposure in broilers. Sulphation was considered as a detoxification pathway (Yi et al. Citation2006). Then, this pathway of biotransformation may explain the low susceptibility of broilers to DON toxicity (Wan et al. Citation2014).
Next to the extensive metabolization on DON-3S, DON is rapidly eliminated in broiler chickens. The results of rapid clearance and short elimination of DON found in the current study were comparable with a previous report in which they found a total body clearance of 0.12 L/min kg and an elimination half-life of 27.9 min after intravenous administration of 0.75 mg/kg BW DON to broiler chickens (Osselaere et al. Citation2013). Similarly, clearance of DON was 0.11 L/min and T1/2elimination was 18.6 min in broiler chickens exposed to the DON mycotoxin at 0.5 mg/kg BW intravenously (Broekaert et al. Citation2017). This is again comparable to turkeys with a clearance of 0.13 L/min and is rapidly eliminated (T1/2elimination = 37.2 min) upon intravenous DON injection at 0.75 mg/kg BW (Devreese et al. Citation2015). Likewise, the toxicokinetic study of DON in pigeon revealed that upon intravenous administration of 0.3 mg/kg BW, the total body clearance was 0.20 L/min kg and the elimination half-life was 20.4 min (Antonissen et al. Citation2016). The rapid elimination is probably linked with the polarity of DON and the lower volume of distribution. The rapid clearance and elimination might be the reason that poultry are less sensitive to DON compared with other species (Antonissen et al. Citation2016).
5. Conclusions
In conclusion, from the results of this research, it could be confirmed the low absorption, the limited accumulation and the rapid clearance and excretion of DON in broilers. DON was metabolized in DON-3S and this latter phase II metabolite can be considered the suitable biomarker of DON exposure in broiler chickens. The characteristics of ADME process of DON in broiler chickens imply the tolerance of this specie to the toxic effects of this mycotoxin.
Acknowledgment
Insaf Riahi gratefully acknowledges Phileo by Lesaffre for their pre-doctoral grant.
Disclosure statement
No potential conflict of interest was reported by the author(s).
Additional information
Funding
References
- Aguzey HA, Gao Z, Haohao W, Guilan C, Zhengmin W, Junhong C. 2019. The effects of deoxynivalenol (DON) on the gut microbiota, morphology and immune system of chicken – a review. Ann Anim Sci. 19(2):305–318.
- Antonissen G, Haesendonck R, Devreese M, Broekaert N, Verbrugghe E, De Saeger S, Audenaert K, Haesebrouck F, Pasmans F, Ducatelle R, et al. 2016. The impact of deoxynivalenol on pigeon health: occurrence in feed, toxicokinetics and interaction with salmonellosis. PLoS ONE. 11(12):e0168205–14. doi:10.1371/journal.pone.0168205.
- Awad WA, Hess M, Twarużek M, Grajewski J, Kosicki R, Böhm J, Zentek J. 2011. The impact of the Fusarium mycotoxin deoxynivalenol on the health and performance of broiler chickens. Int J Mol Sci. 12(11):7996–8012. doi:10.3390/ijms12117996.
- Azizi T, Daneshyar M, Allymehr M, Tukmechi A, Khalilvandi Behroozyar H, Ali Shalizar Jalali AS. 2021. Combination ofLactobacillusspecies and yeast ameliorates adverse effect of deoxynivalenol contaminated diet on immune system, gut morphology and jejunal gene expression in broiler chickens. Ital J Anim Sci. 20(1):59–70. doi:10.1080/1828051X.2020.1865845.
- Bertero A, Moretti A, Spicer LJ, Caloni F. 2018. Fusarium molds and mycotoxins: potential species-specific effects. Toxins (Basel). 10(6):1–27. doi:10.3390/toxins10060244.
- Broekaert N, Devreese M, De Mil T, Fraeyman S, De Baere S, De Saeger S, De Backer P, Croubels S. 2014. Development and validation of an LC-MS/MS method for the toxicokinetic study of deoxynivalenol and its acetylated derivatives in chicken and pig plasma. J Chromatogr B. 971:43–51. doi:10.1016/j.jchromb.2014.09.012.
- Broekaert N, Devreese M, van Bergen T, Schauvliege S, De Boevre M, De Saeger S, Vanhaecke L, Berthiller F, Michlmayr H, Malachová A, et al. 2017. In vivo contribution of deoxynivalenol-3-β-d-glucoside to deoxynivalenol exposure in broiler chickens and pigs: oral bioavailability, hydrolysis and toxicokinetics. Arch Toxicol. 91(2):699–712. doi:10.1007/s00204-016-1710-2.
- Dänicke S, Valenta H, Matthes S. 2007. On the interactions between fusarium toxin-contaminated wheat and nonstarch polysaccharide hydrolyzing enzymes in diets of broilers on performance, intestinal viscosity, and carryover of deoxynivalenol. Poult Sci. 86(1):291–298. doi:10.1093/ps/86.2.291.
- Devreese M, Antonissen G, Broekaert N, De Mil T, De Baere S, Vanhaecke L, De Backer P, Croubels S. 2015. Toxicokinetic study and oral bioavailability of deoxynivalenol in Turkey poults, and comparative biotransformation between broilers and Turkeys. World Mycotox J. 8(4):533–539. doi:10.3920/WMJ2014.1843.
- European Commission. 2006. Commission recommendation of 17 August 2006 on the presence of deoxynivalenol, zearalenone, ochratoxin A, T-2 and HT-2 and fumonisins in products intended for animal feeding (2006/576/EC). Official J Eur Union. L229:7–9.
- Filtenborg O, Frisvad JC, Thrane U. 1996. Moulds in food spoilage. Int J Food Microbiol. 33(1):85–102. doi:10.1016/0168-1605(96)01153-1.
- Gruber-Dorninger C, Jenkins T, Schatzmayr G. 2019. Global mycotoxin occurrence in feed: a ten-year survey. Toxins (Basel). 11:375–325. doi:10.3390/toxins11070375.
- Jurisic N, Schwartz-Zimmermann HE, Kunz-Vekiru E, Reisinger N, Klein S, Caldwell D, Fruhmann P, Schatzmayr D, Berthiller F. 2019. Deoxynivalenol-3-sulphate is the major metabolite of dietary deoxynivalenol in eggs of laying hens. World Mycotox J. 12(3):245–255. doi:10.3920/WMJ2018.2429.
- Lauwers M, Croubels S, Letor B, Gougoulias C, Devreese M. 2019. Biomarkers for exposure as a tool for efficacy testing of a mycotoxin detoxifier in broiler chickens and pigs. Toxins (Basel). 11(4):187. doi:10.3390/toxins11040187
- Lun AK, Moran ET, Young LG, Mcmillan EG. 1988. Disappareance of deoxynivalenol from digesta progressing along the chicken`s gastrointestinal tract after intubation with feed containing contaminated corn. Bull Environ Contam Toxicol. 40:317–324. doi:10.1007/BF01689086.
- Osselaere A, Devreese M, Goossens J, Vandenbroucke V, De Baere S, De Backer P, Croubels S. 2013. Toxicokinetic study and absolute oral bioavailability of deoxynivalenol, T-2 toxin and zearalenone in broiler chickens. Food Chem Toxicol. 51(1):350–355. doi:10.1016/j.fct.2012.10.006.
- Osselaere A, Devreese M, Watteyn A, Vandenbroucke V, Goossens J, Hautekiet V, Eeckhout M, De Saeger S, De Baere S, De Backer P, Croubels S. 2012. Efficacy and safety testing of mycotoxin-detoxifying agents in broilers following the European Food Safety Authority guidelines. Poult Sci. 91(8):2046–2054. doi:10.3382/ps.2012-02245.
- Payros D, Alassane-Kpembi I, Pierron A, Loiseau N, Pinton P, Oswald IP. 2016. Toxicology of deoxynivalenol and its acetylated and modified forms. Arch Toxicol. 90(12):2931–2957. doi:10.1007/s00204-016-1826-4.
- Prelusky DB, Hamilton RMG, Trenholm HL, Millerf JD. 1986. Tissue distribution and excretion of radioactivity following administration of 14C-labeled deoxynivalenol to White Leghorn hens*1. Fundam Appl Toxicol. 7:635–645. doi:10.1016/0272-0590(86)90113-2.
- Riahi I, Marquis V, Pérez-Vendrell AM, Brufau J, Esteve-Garcia E, Ramos AJ. 2021. Effects of deoxynivalenol-contaminated diets on metabolic and immunological parameters in broiler chickens. Animals (Basel). 11:147. doi:10.3390/ani11010147.
- Riahi I, Marquis V, Ramos AJ, Brufau J, Esteve-Garcia E, Pérez-Vendrell AM. 2020. Effects of deoxynivalenol-contaminated diets on productive, morphological, and physiological indicators in broiler chickens. Animals (Basel). 10:1795. doi:10.3390/ani10101795.
- Schwartz-Zimmermann HE, Fruhmann P, Dänicke S, Wiesenberger G, Caha S, Weber J, Berthiller F. 2015. Metabolism of deoxynivalenol and deepoxy-deoxynivalenol in broiler chickens, pullets, roosters and turkeys. Toxins (Basel). 7(11):4706–4729. doi:10.3390/toxins7114706.
- Wan D, Huang L, Pan Y, Wu Q, Chen D, Tao Y, Wang X, Liu Z, Li J, Wang L, Yuan Z. 2014. Metabolism, distribution, and excretion of deoxynivalenol with combined techniques of radiotracing, high-performance liquid chromatography ion trap time-of-flight mass spectrometry, and online radiometric detection. J Agric Food Chem. 62(1):288–296. doi:10.1021/jf4047946.
- Yi L, Dratter J, Wang C, Tunge JA, Desaire H. 2006. Identification of sulfation sites of metabolites and prediction of the compounds’ biological effects. Anal Bioanal Chem. 386(3):666–674. doi:10.1007/s00216-006-0495-1.