ABSTRACT
The aim of this research was to evaluate the antibacterial activity of the muscle wall extracts of sea cucumber, Stichopus horrens, against Lactococcus garvieae, Streptococcus iniae, Aeromonas hydrophila, and Yersinia ruckeri bacteria in rainbow trout using the disk-diffusion and well-diffusion methods. In this study, nine sea cucumbers (with the mean weight of 1690 ± 12.18 g) were randomly obtained from two locations in Chabahar Bay, Iran in November 2018. The muscle wall of the body was extracted with each of the ethyl acetate, methanol, and acetone organic solvents. The antibacterial activities of extracts were determined. Only the ethyl acetate extracts of S. horrens in the concentrations at 8 and 12 mg/mL had an inhibitory effect on all the examined bacteria. Y. ruckeri and A .hydrophila bacteria were sensitive to the acetone extracts. The best property was recorded with the S.horrens ethyl acetate extracts, with the MIC value of 0.625 mg/mL against S. iniae and L. garvieae. The MIC values ranging from 0.626 to 1.25 mg/mL were also displayed with the acetone extracts against Y. ruckeri and A. hydrophila, respectively. In conclusion, the ethyl acetate extracts of S. horrens displayed the best spectrum of bactericidal effect obtained from four bacteria strains examined.
1. Introduction
To achieve the sustainable development of rainbow trout (Oncorhynchus mykiss) culture in the aquaculture industry of Iran, maintaining its health status is of great importance (Soltani et al. Citation2012). Pathogenic organisms, particularly Lactococcus garvieae (Haghighi Karsidani et al. Citation2010), Aeromonas hydrophila (John et al. Citation2011), Yersinia ruckeri (Tobback et al. Citation2007), and Streptococcus iniae (Akhlaghi et al. Citation2011), have rapidly spread in rainbow trout culture farms and have suffered serious economic losses. Today, the increasing failure of chemotherapy and antibiotic resistance displayed by pathogenic bacteria infectious agents have caused the screening of new secondary metabolites with various chemical structures of marine crustaceans, molluscs and echinoderms with desirable antibacterial activity (Kijjoa and Pichan Citation2004).
Recent years, the role of natural compounds of marine origin has become utmost important (Mancini et al. Citation2007). Natural compounds are usually used to refer to natural chemicals with medicinal properties (Hassanshahian et al. Citation2020). This is typically used for the secondary metabolites produced by living organisms (Sipkema et al. Citation2005). Natural compounds found in marine animals can be accordingly used as a rich source of compounds in food, medicine, pigments, and perfumes (Farjami et al. Citation2013). So far, researchers have studied a variety of antibacterial compounds, including polyhydroxylated sterols (Isaac Dhinakaran and Lipton Citation2014), naphthoquinone pigments lysozymes, peptide antibiotics (Cusimano et al. Citation2019), and steroidal glycosides (Claereboudt et al. Citation2019) from enchinoderms.
Bioactive compounds can be also isolated from various animal groups such as corals, crabs, tonics, fish thorns, and sponges (Kijjoa and Pichan Citation2004). Studies on the biological properties of the marine invertebrates have further demonstrated that most of the chemical compounds with biological properties belong to sea cucumbers (Farjami et al. Citation2013). As well, the presence of a variety of bioactive compounds, such as essential fatty acids, lectins, glycosaminoglycans (GAGs), phenolics, chondroitin sulphates, sulphated polysaccharides, cerberosidespeptides, glycoproteins, glycosphingolipids, terpenoids, triterpene glycosides (saponins), and sterols (glycosides and sulphates) can be effective on pharmacological and therapeutical properties of sea cucumbers (Datta et al. Citation2015). Numerous research studies have been performed on the antibacterial activities of different body wall extracts of Parastichopus parvimensis (Villasin and Pomory Citation2000), Actinopyja echimites, Aularches miliaris, Holothuria arta, Holothuria scabra (Abraham et al. Citation2001), Bohadschia marmorata (Mokhlesi et al. Citation2012), and Holothuria leucospilota (Farjami et al. Citation2013) against various pathogenic bacteria.
Sea cucumbers are also a large group of aquatic animals scattered all over the oceans around the world (Barnes Citation1987). They usually live near coral reefs or sea-grasses in warm and shallow waters (Yatnita and Syamsudin Citation2014). In this respect, sea cucumbers have a high economic value as well as significant economic applications in East Asia in traditional food and pharmaceutical industries (Bordbar et al. Citation2011).
The aim of the present study was to evaluate the antibacterial activities of the ethyl acetate, methanol, and acetone extracts of the muscle wall of sea cucumber, Stichopus horrens, from Chabahar coastal area, Iran to develop alternative drugs for the prevention or the treatment of diseases especially Streptococcus iniae, Lactococcus garvieae, (Haghighi Karsidani et al. Citation2010), Aeromonas hydrophila, and Yersinia ruckeri in rainbow trout culture farms using both disk-/well-diffusion methods.
2. Materials and methods
2.1. Sample collection
A total of nine live samples of sea cucumber, S. horrens, with the mean weight of 1690 ± 12.18 g and the mean length of 16 ± 1.89 cm were randomly obtained from two locations in Chabahar Bay, in south-eastern Iran (25° 16′ N, 60° 40′ E and 25° 21′ N, 60° 35′ E) in November 2018, and then transferred to the laboratory. Identification of the collected sea cucumbers was performed by applying the Food and Agricultural Organization (FAO) authentication keys. The visceral organs and the coelomic fluids were subsequently discarded (). Afterwards, the muscle wall of the body was cut into pieces of 1 cm2, packed, and then kept at −20°C until extraction (Haug et al. Citation2002).
2.2. Extraction and phytochemical analysis of S. horrens
The S. horrens extracts were prepared using the maceration method (Ridzwan et al. Citation1995). Briefly, 75 g of the frozen specimen was dried in an oven at 45°C for two days, grounded, and extracted with 200 mL of each of the ethyl acetate, methanol, and acetone organic solvents for 72 h. After shaking for one week at 150 rmp, the mixtures were passed through a 45 µm nylon membrane filter and evaporated under vacuum conditions at 40°C by a rotary evaporator (Heidolph Hei VAP Core, Germany) and then the collected supernatant of each sample was kept at 4°C for further analysis.
The extracts obtained were quantitatively examined for their phytochemical constituents using various analytical tests and reagents. The phytochemicals examined were sterol (Liu et al. Citation2007), flavonoid (Mahmoudi et al. Citation2010), phenol (Ebrahimzadeh et al. Citation2009), and saponin (Sun and Pan Citation2005). In the most commonly used approach for sterol analysis requires many steps including grinding, acid hydrolysis using HCl, alkaline saponification using KOH, solvent extraction using hexane and derivatization Gas chromatography (GC) analysis. And, 50 mg of grounded S. horrens was mixed with 200 µg cholestane (Sigma Aldrich, Steinheim, Germany) and 5 mL ethanolic HcL solution (4 mol/L). Then the sample was strongly shaken and refluxed for 1 h at 80°C. After cooling at room temperature, 10 mL ethanolic KOH solution (4 mol/L) was added into the mixture (50 mg sea coccumber sample containing 200 µg cholestane). Again, the sample was shaken and refluxed at 70°C for 1 h. The sample was cooled at room temperature before 5 mL deionized water, 1 mL potassium chloride and 10 mL hexane were added into the mixture for 5 min and then placed into a 100 mL separator funnel. After adding hexane into the mixture three times, hexane phase per samples was collected. Then hexane phase was washed with 3 mL KOH solution (0.25 mol/L) three times and then adjusted to pH 6. The hexane extracts were taken into a 100 mL flat-bottom flask and anhydrous potassium sulphates were added and finally, using vacuum rotator evaporator (Heidolph Hei VAP Core, Germany) were evaporated to 1 mL and shifted into a septum-capped vial. To determine the free sterols, 100 mg of dried S. horrens taken into conical flask, was mixed with 200 µg cholestane and 20 mL dichlormethane. After vibrating by a vibrator machine for one hour at 150 rpm, the mixtures were passed through a 45 µm nylon membrane filter and evaporated to 1 mL using a vacuum rotator evaporator and shifted to septum-capped vials. After drying the extract under nitrogen steam, 50 µL of redistilled dry pyridine and 50 µL of BSTFA (N, O-Bis(trimethylsilyl) trifluoroacetamide) containing 1% TMCS (trimethychlorosilane) were added to it. The mixtures were held overnight at room temperature and 1 mL dichloromethane was added to them and finally analysed by gas chromatographic methods.
To prepare saponin, 500 g of powered sample was extracted with 70% EtOH three times under reflux for 2 h, and then concentrated in vacuum (40°C) to evaporate the solvent to give a small volume. After extracting with ether (3 × 0.5 L), the water layer portion was extracted with n-BuOH until the n-BuOH layer became colourless. The n-BuOH solution was concentrated and dried in vacuum (60°C). The dried extract was subjected to D101 resin column chromatography, washed with H2O, and eluted with EtOH to give about 30.68 of S. horrens solution (yield 6.14%, w/w). Quantitative determination of sterol and saponin was also performed through a G (GC-2010, Shimadzu, Tokyo, Japan) equipped with an auto injector (AOC-20i, Shimadzu, Tokyo, Japan). The temperatures of the injector and the flame ionization detector were 270 and 300 °C, respectively. The silica capillary column (Supelco SP-2560: 100, 0.25 mm, film thickness 0.20 l m) temperature was then raised from 240°C to 260°C at a rate of 2°C per min. Afterwards, it was fixed at 260°C for 30 min. Phenol was determined by the Folin–Ciocalteu reagent. In addition, 100 µL aliquot of extract of S. horrens was blended with 0.8 µL of Folin–Ciocalteu reagent (the Sigma Aldrich, Steinheim, Germany) with dilution 1:10 and put aside for standing for 5 min at room temperature. 0.8 µL of 6% Na2CO3 (Sigma Aldrich, Steinheim, Germany) was added to the mixture and then put aside to incubate for 2 h at the room temperature in dark. The measurement of absorbing the resulting solution was done at 725 nm with a spectrophotometer (Shimadzu, uv-1800, Tokyo, Japan). Finally, the phenol content was calculated as the gallic acid equivalent in mg/g of the extract. For flavonoid assessment colorimetric aluminium chloride method was used. Resin (50 µL of 1:10 g/mL) in methanol was mixed with 150 µL of methanol, 10 µl of 10% AlCL3, 10 µL of 1 M potassium acetate, and 20 µL of distilled water. The solution remained at room temperature for 90 min; the absorbance of the reaction mixture was measured at 415 nm with a spectrophotometer (Shimadzu, uv-1800, Tokyo, Japan). Finally, the flavonoid content was calculated as quercetin equivalent in mg/g of the extract ().
Table 1. Phytochemical compositions of S. horrens extract (number of samples = 3).
2.3. Bacterial strains
The bacterial strains used in the present study were the gram-positive bacteria, namely, Lactococcus garvieae PTCC1884 and Streptococcus iniae PTCC188 and the gram-negative ones, i.e. Aeromonas hydrophila ATCC7966 and Yersinia ruckeri PTCC1887. All culture stocks were also grown in the Mueller-Hinton broth (MHB) (Merck KGaA, Darmstadt, Germany) at room temperature.
2.4. Determination of antibacterial activity of S. horrens extracts by the disk-diffusion method
The antibacterial activities of S. horrens extracts on the tested bacteria were determined using the disk-diffusion method (Villasin and Pomory Citation2000). For each extract, 4, 8, and 12 mg/mL concentrations were also used to provide three disks. Upon the preparation of the suspensions of the tested bacteria through the 0.5 MCFarland standard under a hood, the bacterial strains were inoculated with a swab on the Mueller-Hinton broth (MHB). Then, the discs containing different concentrations were placed on bacterial culture media and kept in a 25°C incubator for two days. Antibiotics, namely, tetracycline and enrofloxacin (30 µg/mL) were further employed as a positive control. Also, dimethyl sulphoxide-impregnated discs (DMSO) were used as a negative control. The bacterial growth inhibition zone was finally measured with a calliper.
2.5. Determination of antibacterial activity of S. horrens extracts by the well-diffusion method
The minimum inhibition concentration (MIC) values of the S. horrens extracts on the above-mentioned bacteria were determined by the well-diffusion method with some modification (Thornsberry and McDougal Citation1983). After adjusting the overnight cultures of the tested bacteria to 1 × 106 colony-forming units (CFU)/mL, 100 µL of two-fold serial dilutions of each S. horrens extract was added to the well of the sterile 96-well microtiter plates, containing 100 µL of each of the bacterial suspensions in the MHB, then incubated. In this study, the serial dilutions of each S. horrens extract ranged from 0.019 to 10 mg/mL. After incubation for two days at 25°C, the MIC values were evaluated by a microtitre plate reader (MRP4 plus, Hiperion Co. UK). The MIC value was further distinguished as the lowest concentration of the S. horrens extract inhibiting bacterial growth. To measure the minimum bactericidal concentration (MBC), 100 µL of the wells showing no bacterial growth was cultured on the MHB and incubated at 25°C for 24 h. The MBC value of S. horrens was then demonstrated as the lowest concentration that decreased the viability of the bacterium to ≥99.9% (Kang et al. Citation2011). The tests were performed in triplicate.
2.6. Statistical analysis
The data were analysed using the IBM SPSS Statistics (version 16.0) software (Armonk, NY, USA) by parametric tests. Significant differences in growth inhibition zones, MIC, and MBC values of bacterial strains, extract types, and different extract concentrations were determined using one-way analysis of variance (ANOVA) at the 5% confidence interval using the Duncan’s Multiple Range Test.
3. Results
3.1. The phytochemical constitutes of S. horrens extract
The phytochemical components of S. horrens crude extract S. horrens are presented in . The results confirmed that the predominant compounds of S. horrens extract were phenol compounds.
3.2. Designation of antibacterial activity of S. horrens extracts by the paper disc-diffusion method
The results given in proved that only the ethyl acetate extracts of S. horrens in the concentrations at 8 and 12 mg/mL had an inhibitory effect on all the examined bacteria. The ethyl acetate extract displayed the most inhibition zones on Y. ruckeri ( (c)) and S. iniae with 12.03 and 12 mm, respectively. However, the methanol extracts did not show inhibitory effects on the tested bacteria except for Y. ruckeri ((a) and ). Only Y. ruckeri ((b)) and A. hydrophila bacteria were sensitive to the acetone extracts. But, the sensitivity of A. hydrophila to the acetone extracts was more than that of Y. ruckeri. The results of antibacterial activity of tetracycline and enrofloxacin, summarised in , revealed that A. hydrophila and S. iniae were the most sensitive ones against tetracycline and enrofloxacin, respectively.
Figure 2. Effect of methanol, acetone, and ethyl acetate extracts on the growth inhibition of Y. ruckeri.
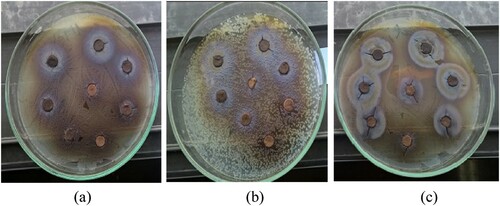
Table 2. Inhibition zone diameter (mm) in different concentrations of three S. horrens extracts, tetracycline, and enrofloxacin on all tested bacteria.
3.3. Designation of antibacterial activity of S. horrens extracts by the well-diffusion method
According to , the optical density (OD) absorption of the tested bacterial strains decreased as the concentration of S. horrens extracts augmented. The ethyl acetate extract of S. horrens in different concentrations had the best OD absorption of the tested bacterial strains, while only OD absorption of Y. ruckeri and A. hydrophila bacteria decreased as the concentration of acetone extract of S. horrens augmented. The results presented in demonstrated that S. horrens extracts exhibited selective antibacterial properties. The best property was recorded with the S. horrens ethyl acetate extracts, with the MIC value of 0.625 mg/mL against S. iniae and L. garvieae. The MIC values ranging from 0.626 to 1.25 mg/mL were also displayed with the acetone extracts against Y. ruckeri and A. hydrophila, respectively. Only the methanol extracts of S. horrens were effective in Y. ruckeri with the MIC value of 0.625 mg/mL. The ethyl acetate extracts of S. horrens also illustrated the best range of bactericidal effect with a ratio of MBC/MIC≤4 gained on four bacterial strains examined in this study.
Figure 3. OD-600 nm absorption of tested bacterial strains in different concentrations of three S. horrens extracts.
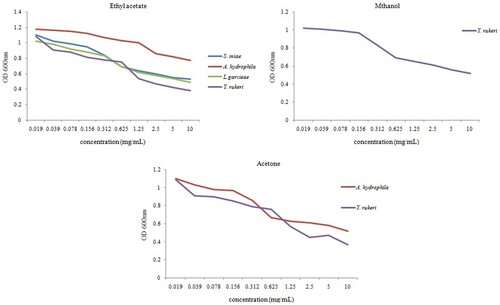
Table 3. MIC, MBC values (mg/mL), and MBC/MIC ratio of three S. horrens extracts on all bacteria.
4. Discussions
The multi-resistant nature of pathogens to antibiotic is a significant challenge for pathogenic bacterial infections. Therefore, the search for novel antimicrobial agents from various natural sources has become an essential and urgent need (Hassanshahian et al. Citation2020). On the other hand, there is an essential and continuous demand to extract new secondary metabolites with a variety of chemical structures of sea cucumbers with potential antibacterial activities (Hamayeli et al. Citation2019). Also, it seems that sea cucumber species, extract types, as well as bacterial strains are effective in forming the growth inhibition zone (Shakouri et al. Citation2017).
In the present study, some of the antibacterial compounds of S. horrens were determined. The results confirmed that the dominant compounds of S. horrens extracts were phenol compounds. Similar to our results, Suleria et al. (Citation2015) concluded that brown phenolic compounds, including eckol, dieckol and phloroglucinol contributed to the antibacterial activities. Also, Manal et al. (Citation2015) explained that the phenolic contents of the Nitraria retusam extract had a positive relationship with the potential antimicrobial activity. Tamokou et al. (Citation2011) and Hamayeli et al. (Citation2019) evaluated the chemical components of Brillantaisia lamium and Stichodactyla haddoni, respectively. Their results detected that the major antibacterial agents in this plant and sea anemone were a mixture of sterols (Tamokou et al. Citation2011; Hamayeli et al. Citation2019). In our study, we recognised these components as antibacterial agents in sea cucumber. Likewise, the antimicrobial potential of sea cucumber extract can be attributed to the presence of antimicrobial agents such as steroidal saponins (Bordbar et al. Citation2011). Other metabolites, such as polyunsaturated fatty acids (PUFAs) (Svetashev et al. Citation1991), glycolipids (Vaskovsky et al. Citation1970), polyamines (Hamana et al. Citation1990), carotenoids (Bullock and Dawson Citation1970), and sterols (Makarieva et al. Citation1993), may additionally act as bioactive compounds. So, the antibacterial activities of sea cucumber extracts may be due to the accumulation of several bioactive compounds.
Numerous pharmacological and chemical studies on various species of sea cucumber have demonstrated that such invertebrates contain triterpene tetraglycosides with antibacterial, antifungal, and cytotoxic properties (Kalinin et al. Citation2015; Cuong et al. Citation2017). The results obtained in our study indicated that ethyl acetate extracts were able to isolate the bioactive compounds present in S. horrens and had the most significant antibacterial effect on gram-positive bacteria such as L. garvieae and S. iniae. Moreover, the methanol extracts did not show inhibitory impacts on the tested bacteria except for Y. ruckeri. Our results are in agreement with those obtained by Hirimuthugoda et al. (Citation2006) reported that the methanol–acetone extracts obtained from the body wall of sea cucumber, Parastichopus parvimensis, from Santa Catalina Island, in southern California, the United States, was found effective in gram-negative bacteria, i.e. Escherichia coli and Bacillus subtilis using the disk-diffusion method. Sedov et al. (Citation1990) and Mulyndin and Kovalev (Citation2001) showed that the presence of secondary metabolites such as triterpene-glycosides can also boost the production of antibodies, protective effect of vaccines, and stimulated antibacterial resistance in mice against conditional pathogenic gram-negative microorganisms. Likewise, the antimicrobial potential of these extracts can be attributed to the presence of antimicrobial agents such as steroidal saponins (Bordbar et al. Citation2011) and sterols (Makarieva et al. Citation1993) which may additionally act as bioactive compounds. The present study explained that only the ethyl acetate extracts of S. horrens in the concentrations at 8 and 12 mg/mL had an inhibitory effect on all the examined bacteria and displayed the most inhibition zones on Y. ruckeri and S. iniae, respectively. Contrary to our results, Mokhlesi et al. (Citation2012) reported that aqueous ethyl acetate extracts had failed to form growth inhibition zones on the tested bacteria (e.g. E.coli, Salmonella aureus, and Pseudomonas aeruginosa). Accordingly, it seems that species of sea cucumbers, extract types, and different extract concentrations can be effective in antibacterial properties. The discrepancy in the effect of sea cucumbers on different bacterial strains is associated with different amino acid sequences extracted from them (Shakouri et al. Citation2017). These results are in agreement with earlier reports proved that different concentrations of extracts from sea cucumber species have been effective in antimicrobial properties. Furthermore, the diameter of the bacterial growth inhibition zone has often reduced as the extract concentrations have dropped (Mokhlesi et al. Citation2012; Omran and Allam Citation2013; Shakouri et al. Citation2017).
Based on the study results, the best property was recorded with the S. horrens ethyl acetate extracts, with the MIC value of 0.625 mg/ mL against S. iniae and L. garvieae. In addition, the ethyl acetate extracts established a bactericidal effect against S. iniae and L. garvieae at concentrations of 1.25 and 2.5 mg/mL, respectively. This indicated that ethyl acetate extract was able to isolate the bioactive compounds presented in S. horrens and had the most significant antibacterial effect on gram-positive bacteria such as L. garvieae and S. iniae, and they could lead to the death of S. iniae at the lowest concentration. Our results are in agreement with earlier studies (Villasin and Pomory Citation2000; Nazemi et al. Citation2014).
5. Conclusions
It seems that different concentrations of the extract, extract types, as well as bacterial strains are effective in forming the growth inhibition zone. This study revealed that the ethyl acetate extracts of S. horrens displayed the best spectrum of bactericidal effect with a ratio of MBC/MIC≤4 obtained from four bacteria strains examined by both disk-diffusion and well-diffusion methods. Thus, the ethyl acetate extracts of S. horrens with a concentration of 12 mg/g can be used as bioactive compounds for growth inhibition and finally lead to the death of bacteria in rainbow trout breeding farms. Ultimately, the results of this study can provide the basis for further research in the future to isolate the effective ingredients of sea cucumber, S. horrens, and prepare appropriate drug formulations of the best active ingredients.
Ethical statement
This article does not contain any studies on animals performed by any of the authors.
Acknowledgements
The authors’ special thanks go to the personnel of the laboratory affiliated to the Faculty of Aquatic Health at XXX University, XXX, Iran, for their valuable assistance in providing instrumentation to this study.
Disclosure statement
No potential conflict of interest was reported by the author(s).
References
- Abraham TJ, Nagarajan J, Shanmugan SA. 2001. Antimicrobial substances of potential biomedical importance from holothurian species. Indian J Mar Sci. 31:161–164.
- Akhlaghi M, Hoseini A, Nematollahi A, Hoseini SM. 2011. Humoral and non-specific immune responses in rainbow trout (Oncorhynchus mykiss) naturally exposed to and immunized with streptococcus iniae. Iran J Vet Med. 5:218–224. doi:10.22059/ijvm.2011.23577.
- Barnes RD. 1987. Invertebrate zoology, 5th ed. New York (NY): Saunders.
- Bordbar S, Anwar F, Saari N. 2011. High-value components and bioactives from sea cucumbers for functional foods–a review. Mar Drugs. 9:1761–1805. doi:10.3390/md9101761.
- Bullock E, Dawson CJ. 1970. Carotenoid pigments of the holothurian psolus fabricii dubenet koren (the scarlet psolus). 799–804. Comp Biochem Physio. 34:700–804.
- Claereboudt EJS, Caulier G, Decroo C, Colson E, Gerbaux P, Claereboudt MR, Eeckhaut I. 2019. Triterpenoids in echinoderms: fundamental differences in diversity and biosynthetic pathways. Mar Drugs. 17:352. doi:10.3390/md17060352.
- Cuong NX, Hoang L, Hanh TT, Van Thanh N, Nam NH, Thung DC, Van Kiem P, Van Minh C. 2017. Cytotoxic triterpene diglycosides from the sea cucumber stichopus horrens. Bioorg Med Chem Lett. 27:2939–2942. doi:10.1016/j.bmcl.2017.05.003.
- Cusimano MG, Spinello A, Barone G, Schillaci D, Cascioferro S, Magistrato A, Vitale MA. 2019. Synthetic derivative of antimicrobial peptide holothuroid in 2 from mediterranean sea cucumber (holothuria tubulosa) in the control of listeria monocytogenes. Mar Drugs. 17:159–164. doi:10.3390/md17030159.
- Datta D, Talapatra SN, Swarnakar S. 2015. Bioactive compounds from marine invertebrates for potential medicines – An overview. Int Lett Nat Sci. 34. doi:10.18052/www.scipress.com/ILNS.34.42.
- Ebrahimzadeh M, Nabavi SM, Nabavi SF, Eslami B. 2009. Free radical scavenging ability of methanolic extract of hyoscyamus squarrosus leaves. Pharmacologyonline. 2:796–802.
- Farjami B, Nematollahi MA, Moradi Y, Irajian G, Nazemi M, Ardebili A, Pournajaf A. 2013. Antibacterial activity of the sea cucumber holothuria leucospilota. Int J Mol Clin Microbiol. 3:225–230.
- Haghighi Karsidani S, Soltani M, Nikbakhat-Brojeni G, Ghasemi M, Skall HF. 2010. Molecular epidemiology of zoonotic streptococcosis/lactococcosis in rainbow trout (Oncorhynchus mykiss) aquaculture in Iran. Iran J Microbiol. 2:198–209.
- Hamana K, Niitsu M, Samejima K, Matsuzaki S. 1990. Thermopentamine, a novel linear pentaamine found in thermus thermophilus. FEMS Microbiol Lett. 68:27–30. doi:10.1111/j.1574-6968.1990.tb04116.x.
- Hamayeli H, Hassanshahian M, Askari Hesni M. 2019. The antibacterial and antibiofilm activity of sea anemone (Stichodactyla haddoni) against antibiotic-resistant bacteria and characterization of bioactive metabolites. Int Aquat Res. 11:85–97. doi:10.1007/s40071-019-0221-1.
- Hassanshahian M, Saadatfar A, Masoumipour F. 2020. Formulation and characterization of nanoemulsion from alhagi maurorum essential oil and study of its antimicrobial, antibiofilm, and plasmid curing activity against antibiotic-resistant pathogenic bacteria. J Environ Health Sci Eng. 18:1015–1027. doi:10.1007/s40201-020-00523-7.
- Haug T, Kjuul A, Stensvåg K, Sandsdalen E, Styrvold O. 2002. Antibacterial activity in four marine crustacean decapods. Fish Shellfish Immunol. 12:371–385. doi:10.1006/fsim.2001.0378.
- Hirimuthugoda N, Chi Z, Ling Z. 2006. Probiotics and sea cucumber farming. SPC Bechedemer Inf Bull. 24.
- Isaac Dhinakaran D, Lipton AP. 2014. Pharmacological potentials of sea cucumber holothuria atra extracts from the Indian ocean. Asian J Biomed Pharm Sci. 4:36–43. doi:10.15272/ajbps.v4i35.537.
- John G, Rathna Kumari P, Balasundaram A. 2011. Health promoting biochemical effects of three medicinal plants on normal and aeromonas hydrophila infected labeo rohita. J Fish Aquat Sci. 6:633–641. doi:10.3923/jfas.2011.633.641.
- Kalinin VI, Avilov SA, Silchenko AS, Stonik VA. 2015. Triterpene glycosides of sea cucumbers (holothuroidea, echinodermata) as taxonomic markers. Nat Prod Commun. 10:21–26.
- Kang C-G, Hah D-S, Kim C-H, Kim Y-H, Kim E, Kim J-S. 2011. Evaluation of antimicrobial activity of the methanol extracts from 8 traditional medicinal plants. Toxicol Res. 27:31–36. doi:10.5487/TR.2011.27.1.031.
- Kijjoa A, Pichan S. 2004. Drugs and cosmetics from the Sea. Mar Drugs. 2:73–82. doi: 10.3390/md202073.
- Liu W-H, Ding B, Ruan X-M, Xu H-T, Yang J, Liu S-M. 2007. Analysis of free and conjugated phytosterols in tobacco by an improved method using gas chromatography-flame ionization detection. J Chromatogr A. 1163:304–311. doi:10.1016/j.chroma.2007.06.043.
- Mahmoudi M, Ebrahimzadeh MA, Nabavi SF, Hafezi S, Nabavi SM, Eslami SH. 2010. Antiinflammatory and antioxidant activities of gum mastic. Eur Rev Med Pharmacol Sci. 14:765–769.
- Makarieva TN, Stonik VA, Kapustina II, Boguslavsky VM, Dmitrenoik AS, Kalinin VI, Djerassi C. 1993. Biosynthetic studies of marine lipids. 42. biosynthesis of steroid and triterpenoid metabolites in the sea cucumber eupentacta fraudatrix. Steroids. 58:508–517. doi:10.1016/0039-128X(93)90026-J.
- Manal Y, Sameeh AA, Mohamed SI, Ali OM, Darwesh SM, El-Hallouty MY. 2015. Chemical compositions, potential cytotoxic and antimicrobial activities of Nitraria retusamethanolic extract sub-fractions. International J Toxicol Pharmacol Res. 7:204–217.
- Mancini I, Defant A, Guella G. 2007. Recent synthesis of marine natural products with antibacterial activities. Anti-Infect Agents Med Chem. 600:17–48. doi:10.2174/187152107779314151.
- Mokhlesi A, Saeidnia S, Gohari AR, Shahverdi AR, Nasrolahi A, Farahani F, Khoshnood R, Es’haghi N. 2012. Biological activities of the sea cucumber holothuria leucospilota. Asian J Anim Vet Adv. 7:243–249. doi:10.3923/ajava.2012.243.249.
- Mulyndin VA, Kovalev VV. 2001. Effects of the extraction of internal organs of the holothurian cucumaria japonica on the indices of nonspecific resistance. Russ J Mar Biol. 27:406–408. doi:10.1023/A:1013717907602.
- Nazemi M, Alidoost Salimi M, Alidoost Salimi P, Motallebi A, Jahromi S, Ahmadzadeh O. 2014. Antifungal and antibacterial activity of haliclona sp. from the persian gulf, Iran. Journal de Mycologie MéDicale/J Med Mycol. 24:220–224. doi:10.1016/j.mycmed.2014.03.005.
- Omran NE, Allam NG. 2013. Screening of microbial contamination and antimicrobial activity of sea cucumber holothuria polii. Toxicol Ind Health. 29:944–954. doi:10.1177/0748233712448116.
- Ridzwan BH, Kaswandi MA, Azman Y, Fuad M. 1995. Screening for antibacterial agents in three species of sea cucumbers from coastal areas of Sabah. Gen Pharmacol: Vasc Syst. 26:1539–1543. doi:10.1016/0306-3623(95)00041-0.
- Sedov A, Apollonin A, Sevastyanova E. 1990. Stimulation of Non-specific antibacterial resistance of mice against conditional pathogenic gram-negative microorganisms by triterpene glycosides. Anti KiiK. 1:23–26.
- Shakouri A, Shoushizadeh MR, Nematpour F. 2017. Antimicrobial activity of sea cucumber (Stichopus variegatus) body wall extract in chabahar bay, Oman Sea. Jundishapur J Nat Pharm Prod. 12:1–5. doi:10.5812/jjnpp.32422.
- Sipkema D, Franssen MCR, Osinga R, Tramper J, Wijffels RH. 2005. Marine sponges as pharmacy. Mar Biotechnol. 7:142–162. doi:10.1007/s10126-004-0405-5.
- Soltani M, Pirali E, Shayan P, Eckert B, Rouholahi S, Sadr SN. 2012. Development of a reverse line blot hybridization method for detection of some treptococcal/lactococcal species, the causative agents of zoonotic streptococosis/lactococosis in farmed fish. Iran J Microbiol. 4:70–74.
- Suleria HAR, Osborne S, Masci P, Gobe G. 2015. Marine-based nutraceuticals: An innovative trend in the food and supplement industries. Mar Drugs. 13:6336–6351. doi:10.3390/md13106336.
- Sun HX, Pan HJ. 2005. Immunological adjuvant effect of glycyrrhiza uralensis saponins on the immune responses to ovalbumin in mice. Vaccine. 24:1914–1920. doi:10.1016/j.vaccine.2005.10.040.
- Svetashev VI, Levin VS, Cham Ngok L, Do Tuet N. 1991. Lipid and fatty acid composition of holothurians from tropical and temperate waters. Comp Biochem Physiol Part B: Comp Biochem. 98:489–494. doi:10.1016/0305-0491(91)90242-6.
- Tamokou JDD, Kuiate JR, Tene M, Nwemeguela TJK, Tane P. 2011. The antimicrobial activities of extract and compounds isolated from Brillantaisia lamium. Iran J Med Sci. 36:24–31.
- Thornsberry C, McDougal LK. 1983. Successful use of broth microdilution in susceptibility tests for methicillin-resistant (heteroresistant) staphylococci. J Clin Microbiol. 18:1084–1091. doi:10.1128/jcm.18.5.1084-1091.1983.
- Tobback E, Decostere A, Hermans K, Haesebrouck F, Chiers K. 2007. Yersinia ruckeri infections in salmonid fish. J Fish Dis. 30:257–268. doi:10.1111/j.1365-2761.2007.00816.x.
- Vaskovsky VE, Kostetsky EY, Svetaschev VI, Zhukova IG, Smirnova GP. 1970. Glycolipids of marine invertebrates. Comp Biochem Physiol. 34:163–177. doi:10.1016/0010-406X(70)90064-2.
- Villasin, J., Pomory C. M. 2000. Antibacterial activity of extracts from the body wall of parastichopus parvimensis (echinodermata: holothuroidea). Fish Shellfish Immunol. 10:465–467. doi:10.1006/fsim.2000.0265.
- Yatnita PC, Syamsudin A. 2014. Antibacterial activities of bacterial symbionts of soft corals collected from the water of Wai-Sai island. Papua Pharmacia Lett. 6:8–13.