ABSTRACT
Melanin is the key functional ingredient of silky fowl that has nutritional and medicinal benefits. Chishui silky fowl has unique black feather, black skin, green shell egg and black claws, while the melanin deposition of main tissues in the Chishui silky fowl remains unknown. This study aimed to investigate the pigmentation in four tissues of Chishui silky fowls compared with Taihe silky fowl from the lightness value of different tissues black degree, melanin content and the mRNA expression levels of pigmentation related genes such as ASIP, MC1R, MITF, TYR and TYRP1 by using colorimeter, enzymatic hydrolysis and RT–PCR, respectively. The results revealed that the lightness values of the chest muscle and liver, and the melanin content of leg muscle in the Taihe silky fowl were significantly higher than Chishui silky fowl hens (P < 0.05). Compared with Chishui silky fowl, the mRNA expression levels of MITF, TYR and TYRP1 were significantly upregulated in the skin of Taihe silky fowl (P < 0.05). In conclusion, the comparative analysis from lightness value, melanin content and mRNA expression levels of pigmentation-related genes indicated that Chishui silky fowl has equivalent pigmentation in the skin, muscles (chest and leg) and liver compared with Taihe silky fowl.
Introduction
Black skin is the most distinctive characteristic and important trait of the silky fowl. In China, the nutritional and medicinal benefits of silky fowl have been confirmed and most people prefer to purchase silky fowl because it can nourish the body (Tu et al., Citation2009). Chishui silky fowl is one of the native chicken breeds of Chishui city in the Guizhou Province of China. The four most unique characteristics of the Chishui silky fowl are black feathers, black skin, laying green shell eggs and black claws. Compared with Chishui silky fowl, Taihe silky fowl is the most famous silky fowl in China from Taihe counties in the Jiangxi Province of China, which has purely white feathers (Tu et al., Citation2009). Interestingly, we found that the skin black degree of Chishui silky fowl was below that of Taihe silky fowl and the feather colour was significantly different from Taihe silky fowl (black vs. white). Skeletal muscles (breast muscle and leg muscle) are the main parts of meat production. For consumer purposes, hyperpigmentations in muscle are highly requested by customers. Liver is one of the representatives of internal organs and also is the most metabolic organ. However, silky fowls are known for not only their special phenotypes but also the atypical distribution of melanocytes among the internal organs. Melanocytes are the main cell type present in avian species and mammals, which produce melanin that is responsible for determining body colour (Serbedzija et al. Citation1989, Slominski et al. Citation2004). The production of melanin is mainly regulated by pigmentation related-gens such as ASIP, MC1R, TYR, TYRP1 and MITF, which can regulate the synthesis of melanin and promote the formation of black feathers and black skin, eventually.
Melanin deposition is a complex trait that depends on genetics and other factors, including the environment and nutrition or some specific drugs (Sturm and Duffy Citation2012, Dereure et al. Citation2001, Jablonski et al. Citation2010). Melanin, including eumelanin and pheomelanin, is responsible for the formation of feather colour and black skin of silky fowl (Slominski et al. Citation2004). Pigmentation is mainly affected by a large number of genes expression involved in the different stages of melanogenesis, migration and maturation of the melanocytes to the production of melanin (Hoekstra. Citation2006). Many genes have been found to play important roles in pigmentation by genome-wide association scans (GWAS), based on the previous reports which included ASIP, TYR, MC1R, MITF, TYRP1, KIT and SLC24A (Hart et al. Citation2013, Duffy et al. Citation2010, Nan et al. Citation2009, Sulem et al. Citation2007, Minvielle et al. Citation2010 ). Melanocortin 1 receptor (MC1R) is responsible for the switch from pheomelanin to eumelanin synthesis, through increasing tyrosinase activity via cAMP signalling after the αmelanocyte-stimulating hormone (α-MSH) bind to the receptor (Robbins et al. Citation1993). The Agouti locus encodes the agouti signalling protein (ASIP), a small paracrine signalling molecule that antagonizes with α-MSH by the MC1R interaction (Yang et al. Citation2015, Oribe et al. Citation2012). ASIP gene inhibits the cascade of signals that activate melanogenic enzymes, leading to a decrease in eumelanin production (Graham et al. Citation1997). Melanin synthesis in melanocytic cells is ultimately regulated by tyrosinase (TYR) which is the critical rate-limiting enzyme. It mainly oxidized tyrosine to dopa and further oxidized dopa to dopaquinone, and then the dopaquinone produced melanin by biochemical reactions (Ito.Citation2006, Ando et al. Citation2007). TYRP1 plays an important role in stabilizing TYR in addition to its catalytic function in oxidizing 5,6-dihydroxyindole-2-carboxylic acid (DHICA) and is a critical rate-limiting enzyme in the regulation of melanin formation (Kobayashi et al. Citation1998). MITF is involved in the development of melanocytes and responsible for the plumage colour both in the Japanese quail, gallus, and in determining skin/coat colours in chickens Minvielle et al. Citation2010). Previous studies only focused on the pigmentation-related gene expression levels in animals with different coat colours or different stages in the same breeds. Whereas few reports have investigated the pigmentation in the different tissues or organs of Chishui silky fowl, which directly affects the selective breeding and market consumption of Chishui silky fowl, and causes economic losses every year. Considering their significant difference of feather colour and the degree of darkness in the skin between Chishui silky fowl and Taihe silky fowl, we hypothesize that the differential expression of pigmentation-related genes and the pigmentation difference in the tissues or organs are the main inducing factors. Thus the objective of the present study was to comparatively analyse the darkness degree, melanin contents and the mRNA expression levels of pigmentation-related genes in the skin, muscle (breast muscle and leg muscle) and liver of Chishui silky fowl and Taihe silky fowl.
Materials and methods
Animals and sample collection
A total of 60 six-month-old Taihe silky fowl and Chishui silky fowl were randomly selected for the sample collection, which were fed with the same nutrition level diet at the poultry farm of Guizhou University, Guizhou, China. All animals were divided into two groups according to breed (30 birds per breed and 15 per gender). The back skin, chest muscle, leg muscle and liver were used for the measurement of lightness value and the extraction of melanin after slaughter. Briefly, 3 × 3 cm2 area of back skin (refers to the skin on the back of the ribcage, and the hair follicles were removed), chest muscle (superficial pectoral muscle), leg muscle (superficial thigh muscle) and liver (right lobe liver) were used to detect the lightness value and then 15 g of above these tissues or organs were collected for the extraction of melanin after measurement. Likewise, these tissues or organs were processed and washed by ice-cold PBS to remove the blood and immediately placed in liquid nitrogen, and stored at −80℃ until they were processed for mRNA expression.
Ethics statement
All works in the current experiment were followed by the Chinese Guidelines for Animal Welfare and approved by the Guizhou University Institutional Animal Care and Use Committee (Guiyang, China).
Measurement of lightness value
First, the skin, liver and muscles (chest muscle and leg muscle) degree of darkness of each experimental chicken were determined using a WSC-S colorimeter (Shanghai Precision Scientific Instrument Co., Ltd.). All lightness values were obtained from two areas of each tissue. A higher reading of L value indicated a lower degree of blackness and lower melanin deposits, and vice versa. The WSC-S colorimeter was calibrated and adjusted to L*a*b mod before measurement. To eliminate the effect of the bottom colour of the bottom plate on the lightness value, all tissues were placed on the white filter paper before each sample measurement. Briefly, each sample was measured in two areas and keep a measuring distance of 2 cm between the colorimeter and the tissue.
Extraction and determination of melanin
The extraction of melanin was performed by enzymatic hydrolysis and the melanin content was determined using UV spectrophotometry. Melanin extraction of four tissues and evaluation was performed according to Ozeki et al. (Citation1996), Ito et al. (Citation1985, Citation2006) and Hsieh et al. (Citation2012) reports. Briefly, 15 g back skin, chest muscle, leg muscle and liver samples from each chicken were weighed after the fascia, membrane and fat were removed. These samples were crushed and mixed with 30 mL PBS and 0.6 g papain enzyme, and then water bath and stirring at 65℃ for 4 h. The enzymatic hydrolysate was incubated at 90–100℃ for 20 min, and the clear solution was removed after centrifugation of 5000×g for 20 min at 25℃. The melanin mixture was filtered by gauze and replicated centrifugation three times at 5000×g for 10 min after mixed with 3 mL petroleum ether and shaken well. Likewise, 3 mL absolute ethanol and 10 mL distilled water were separately added to wash the melanin mixture and replicated centrifugation three times at 5000×g for 10 min, and a crude melanin product was obtained by precipitation vacuum freeze-drying. Melanin standards, including 5, 6-dihydroxyindole (DHI) (Sigma, USA), Melanin from Sepia Officinalis also known as pheomelanin (Sigma, USA), and 5, 6-dihydroxyindolecarboxylic acid (DHICA) (Sigma, USA) were used to construct standard curves to calculate melanin content using UV spectrophotometry. The oxidative degradation of crude melanin was referenced to Ito et al. (Citation1985, Citation2006), Hsieh et al. (Citation2012) and Ozeki et al.’s (Citation1996) methods, respectively. Briefly, 150 mg melanin was mixed with 10 mL 1 mol/L K2CO3 and 0.4 mL 30% H2O2, and then water bathing at 100°C for 20 min. 0.6 mL 10% Na2SO3 was added to stop the reaction after running water-cooled, and then mix with 4 mL of 6 mol/L HCl and keep still for 10 min. Finally, melanin standards were diluted to 0, 50, 100, 250, 500, 1000, 2000, 4000, 6000, 8000, 10,000 μg/mL, and crude melanin in the present study was detected by UV spectrophotometry at 340, 350 and 380 nm. Three replicates were detected in each sample. 5, 6-dihydroxyindole (DHI) and 5, 6-dihydroxyindolecarboxylic acid (DHICA) are the oxidative degradation product of eumelanin, and the melanin from sepia officinalis represents the pheomelanin content of the tissue.
Total RNA extraction and Real-Time PCR
The mRNA expression levels of pigmentation-related genes in different tissues were determined using Real-Time PCR. Total RNA was extracted using TRIzol reagent (Takara code: 9109, Shiga, Japan). RNA quality and quantity were determined using a NanoDrop 2000 spectrophotometer (Thermo Fisher Scientific, Massachusetts, USA). cDNA was synthesized with a HiScript II qRT SuperMix Reverse Transcriptase (CoWin Biosciencs, Beijing, China) according to the manufacturer’s instructions. Briefly, a total volume of 20 μl consisting of 1 μg total RNA, 4 μl dNTP Mix (CoWin Biosciencs, Beijing, China), 2 μl Primer Mix, 4 μl 5 × RT Buffer, 2 μl DTT, 2 μl HiFiScript, and double-distilled water up to 20. The reverse transcription was conducted at 42℃ for 30 min and 85℃ for 5 min. The real-time PCR was performed on a CFX96TM Real-Time System (Bio-Rad, Hercules, CA, USA) in triplicate, in a total volume of 20 μl consisting of 10 μl SYBR Premix PCR kit (Thermo Fisher, USA), 0.4 μl each of primer (10 μM), 2 μl of cDNA template and 9.2 μl double-distilled water. The PCR cycle conditions were 95℃ for 30 s, followed by 40 cycles of 95℃ for 5 s and 60℃ for 30 s. Melting curve analysis was used to confirm the specificity and reliability of PCR products. There were six to eight samples in each group, each sample was conducted in duplicate and no template control was included. GAPDH was used as a housekeeping genes to normalize target gene levels. The relative mRNA expression was calculated using the 2-△△Ct method. Premiers used in this study were designed with Premier 5.0 () and synthesized in Tsingke (Chongqing, China).
Table 1. Primers used for quantitative real-time PCR.
Statistical analysis
Statistical analysis was performed with Student’s t-test with Welch’s correction with SPSS 20.0 (SPSS, Chicago, IL, USA). Data are represented as mean ± SD and differences are considered significant at P value < 0.05.
Results
Comparative analysis of lightness value
The lightness values in different tissues were evaluated by colorimeter in the current study. As shown in , the lightness values of the chest muscle and liver in the Taihe silky fowl were significantly higher than Chishui silky fowl hens (P < 0.05), whereas there is no significant difference between the Taihe silky fowl and Chishui silky fowl rooster (P > 0.05). No significant difference was observed in the skin and leg muscle among the Taihe silky fowl and Chishui silky fowl (P > 0.05).
Tissues melanin content
The comparative analysis of melanin contents in four tissues of Taihe silky fowl and Chishui silky fowl is summarized in . As shown in , the melanin content in the leg muscle of Chishui silky fowl’s hen was significantly lower than Taihe silky fowl (P < 0.05), whereas the rooster of Chishui silky fowl was not different (P > 0.05). No difference was observed in chest muscle, skin and liver among Chishui silky fowl and Taihe silky fowl (P > 0.05).
Table 2 . Melanin content of different tissues in the Taihe silky fowl and Chishui silky fowl.
mRNA expression levels of pigmentation-related genes
The mRNA expression levels of pigmentation-related genes are shown in . Compared with Taihe silky fowl, the mRNA expression levels of ASIP in the liver of Chishui silky fowl’s rooster were significantly increased, but the expression levels of Chishui silky fowl’s hen were lower than Taihe silky fowl (P < 0.05). The ASIP mRNA expression levels in the liver of the rooster were lower compared with hen’s (P < 0.05). No significant difference was observed in the ASIP mRNA expression levels of skin, chest muscle and leg muscle between Taihe silky fowl and Chishui silky fowl (P > 0.05). Consistently, differential expression levels of MC1R only showed in the liver of Taihe silky fowl and Chishui silky fowl. Compared with Chishui silky fowl, the mRNA expression levels of MC1R in the liver of Taihe silky fowl were significantly increased (P < 0.05). However, compared with rooster of Taihe silky fowl, the MC1R mRNA expression level of the liver in Taihe silky fowl’s hen was significantly increased (P < 0.05), which was inconsistent with the ASIP expression level in the liver of Taihe silky fowl’s hen. In the present study, the mRNA of MITF, TYRP1 and TYR were differentially expressed in the skin and liver of Taihe silky fowl and Chishui silky fowl. In the skin, the expression levels of MITF, TYRP1 and TYR in the Taihe silky fowl were significantly higher than the Chishui silky fowl (P < 0.05). The mRNA expression levels of TYRP1 and TYR were differentially expressed in the roosters and hens of the Taihe silky fowl but the TYR was only differentially expressed in Chishui silky fowl (P < 0.05). However, no difference was observed in the MITF mRNA expression levels among Taihe silky fowl and Chishui silky fowl or gender (P > 0.05).
Figure 2. The mRNA expression levels of ASIP, MC1R, MITF, TYRP1 and TYR in the four tissues of Taihe silky fowl and Chishui silky fowl. a-dColumns with different superscript letters are significantly different between breeds (P < 0.05). Values are represented as mean ± SD (n = 6–8). a: ASIP mRNA expression level in Taihe silky fowl and Chishui silky fowl; b: MC1R mRNA expression level in Taihe silky fowl and Chishui silky fowl; c: MITF mRNA expression level in Taihe silky fowl and Chishui silky fowl; d: TYRP1 mRNA expression level in Taihe silky fowl and Chishui silky fowl; e: TYR mRNA expression level in Taihe silky fowl and Chishui silky fowl.
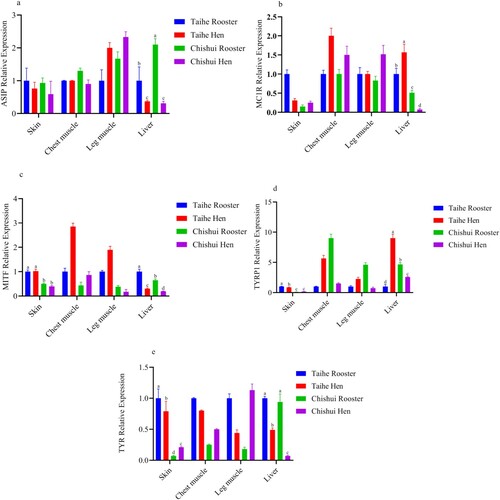
Discussion
The most apparent difference between silky fowls and common chickens is that the skin, muscle, periosteum and other tissues of black-bone chickens contain high levels of melanin (Tian et al. Citation2007). Thus we first evaluated whether the black degree of different tissues of Chishui silky fowl was significantly different from Taihe silky fowl. The results of the comparative analysis showed that the lightness in the chest muscle and liver of Chishui silky fowl was significantly decreased compared to the Taihe silky fowl. However, the degree of darkness in the skin and the leg muscle was not significant different. The black degree of tissues was mainly determined by melanin levels which is a type of high-protein molecule synthesized from melanin bodies in melanocytes (Mackintosh, Citation2001). In the current study, compared with Taihe silky fowl, we found the skin degree of darkness in the Chishui silky fowl was not a significant difference, although they have distinctly different feather colours. Recent reports from Kriangwanich et al (Citation2021) and Nganvongpanit et al (Citation2020)’s study evaluated the muscle darkness and the distribution of melanin pigmentation in 33 Organs of Thai Black-Bone Chickens. They found the dark muscles with the highest degree of darkness mainly included iliotibialis lateralis pars preacetabularis, gastrocnemius, fibularis longus, and puboischiofemoralis pars medialis, and the least dark muscles included serratus profundus, pectoralis, iliotibialis cranialis, flexor cruris lateralis and flexor cruris medialis (Kriangwanich et al. Citation2021). Besides, they also found that the highest and lowest amounts of melanin pigment were noted in the flexor carpi ulnaris and pectoralis. Their results showed that specified muscles of black-bone chickens showed an uneven distribution of darkness due to the differing accumulations of melanin pigments of each muscle. In the present study, we found no significant difference was observed in the black degree of leg muscle between Chishui silky fowl and Taihe silky fowl. However, the important limitation of the present study was that we did not analyse the lightness values of other muscles such as Kriangwanich et al. report. In particular, we found the degree of darkness in the liver of Taihe silky fowl was significantly higher than Chishui silky fowl, which is inconsistent with Nganvongpanit et al (Citation2020)’s report. They found the liver was the only organ in which the melanin pigment had not accumulated among all 33 organs of Thai Black-Bone Chickens. They also found that there was not a uniform pattern of melanin pigment accumulation throughout the organs of the chickens. Regrettably, herein, we only evaluated the lightness values of the liver in the Chishui silky fowl and Taihe silky fowl. Previous studies have indicated that the melanin pigment accumulated in many internal organs of the black-bone chicken (Silky fowls) such as the skin, connective tissues, skeletal muscle, respiratory tract, immune organs, intestines and reproductive organs, but not included liver, pancreas, pituitary gland, adrenal gland, etc. (Han et al. Citation2015). Melanin deposition of silky fowl is mainly affected by both genetics and the environment. The variations in feather and skin colour occurring from mutations of pigmentation genes have been reported in recent studies such as MITF and TYR. For example, MITF, a major pigmentation-related gene involved in determining skin/coat colour in chickens (Li et al. Citation2011). It has been confirmed that the promoter polymorphisms (SNP c.-638T > C) of MITF affected chicken skin colour, and the CC and TC genotypes had significantly lower L* value than those with TT genotype (Wang et al. Citation2018). A recent report found that dietary supplementation with different doses of tyrosine significantly improved breast muscle blackness (Li et al. Citation2011). In the current study, we found there is no significant difference in the blackness of skin and leg muscle between Chishui silky fowl and Taihe silky fowl. Whether the obvious difference of feather colour was induced by gene mutation still needs further investigation in the future.
Melanin is the most important active ingredient of silky fowl, and studies have shown that nutritional and genetic factors can affect the deposition of melanin in the body (Li et al. Citation2011). In the current study, we found that the melanin (included eumelanin and pheomelanin) content of leg muscle from Taihe silky fowl’s hen was significantly higher than Chishui silky fowl’s hen, but had no significant difference in other tissues. The causes of the variations in melanin content may have also occurred because of mutations in the pigmentation-related genes, such as in the TYR and MITF that control the skin or feather colour and the melanin deposition (Wang et al. Citation2018). In Lueyang black-boned chicken, the mRNA of ASIP, TYR, TYRP1 and MITF were differentially expressed in white and black skin (Zhang et al. Citation2015). Besides, black-bone chicken melanin was produced by TYR through a series of biochemical reactions, so the melanin deposition of silky fowl was also affected by nutrition levels such as dietary tyrosine. Li et al. (Citation2019) found that the basal diet supplemented with 0.8% tyrosine significantly improved the blackness degree of breast muscle tissue and yielded the highest melanin content in breast muscle. The difference of melanin content in leg muscle of Taihe silky fowl and Chishui silky fowl may be explained by the differential expression of pigmentation-related genes.
Agouti signalling protein (ASIP) is a paracrine factor that stimulates yellow/red pigment (pheomelanin) synthesis and inhibits black/brown pigment (eumelanin) synthesis in follicular melanocytes, which lead to brown to black and yellow to red colourations (Yang et al. Citation2015, Oribe et al. Citation2012). ASIP is regarded as a competitive inhibitor and antagonist of the melanocyte-stimulating hormone a-MSH and it is centrally involved in the expression of coat-colour traits in animals (Oribe et al. Citation2012). In the present study, we found that the ASIP gene was differentially expressed in the liver of Chishui silky fowl and the Taihe silky fowl, and the expression levels in the rooster were higher than the hen. Previous studies showed that the ASIP gene is responsible for the skin colour of both white- and black-coated sheep (Norris et al. Citation2008). However, our results showed that there was no difference in the skin mRNA expression levels of ASIP between Chishui silky fowl and the Taihe silky fowl, although they have different feathers (black vs white). In the epidermal tissues, the expression level of ASIP was suppressed by MC1R, and it was significantly decreased in the black skin compared with the white-skinned chickens (Zhang et al. Citation2015,Minvielle et al. Citation2010 ). Whereas we found the expression levels of MC1R in the Taihe silky fowl’s liver were significantly higher than Chishui silky fowl and displayed sexual difference among them. MC1R is responsible for binding melanocyte-stimulating hormone (MSH), which stressing keratinocytes and initiating the cascade of melanogenesis (Minvielle et al. Citation2010). Recently, the gene expression of MC1R, which has been identified in several studies as a gene related to pigmentation, showed no difference between the black-skinned and white-skinned Lueyang chickens, and/or the white skin and black spots of sheep (Zhang et al. Citation2015, Penagaricano et al. Citation2012). Consistent with our current study, the mRNA expression levels of MC1R and ASIP showed no significant differences in the skin of Taihe silky fowl and Chishui silky fowl. Consistently, the data of lightness value and melanin content also showed similar results. Whether the feather difference of Taihe silky fowl and Chishui silky fowl was associated with the mutation of ASIP or MCAR is of particular interest for future studies that aim to elucidate their functional role in the regulation of feather colour.
MITF is an important transactivator in melanocytes and regulates the transcription of three melanogenesis-controlling enzymes, which included tyrosinase(TYR), dopachrome tautomerase(DCT) that was controlled by Tyrosinase-related protein 2 (TYRP2) and Tyrosinase-related protein 1 (TYRP1) (Vachtenheim et al. Citation2010). Besides, MITF also regulated the melanocortin 1 receptor (MC1R) and melanosome formation-related proteins (Shah et al. Citation2010; Denecker et al. Citation2014). In this study, the mRNA expression levels of MITF, TYR and TYRP1 were differentially expressed in the skin and liver of Taihe silky fowl and Chishui silky fowl. In the skin, the mRNA expression levels of MITF, TYR and TYRP1 were all significantly increased in the Taihe silky fowl. MITF gene deficiency resulted in the inhibited expression of endogenous tyrosinases (TYR) and TYRP1, which blocked cAMP-induced melanin synthesis (Li et al. Citation2011). Previous studies suggested that MITF was involved in the development of melanocytes and responsible for the plumage colour both in the Japanese quail, gallus and in determining skin/coat colour in chickens (Minvielle et al. Citation2010, Wang et al. Citation2018). Consistently, our results indicated that the mRNA expression levels of MITF, TYR and TYRP1 showed similar trends in the skin of Taihe silky fowl and Chishui silky fowl. Recent reports found that TYRP1 was showing higher expression levels in the skin of black-coated sheep versus white-coated sheep (Fan et al. Citation2013). In our study, TYRP1 showed a higher expression level in the skin of Taihe silky fowl, which is inconsistent and still requires additional research. TYRP1 plays an important role in stabilizing TYR in addition to its catalytic function in oxidizing DHICA and is a critical rate-limiting enzyme in the regulation of melanin formation (Kobayashi et al. Citation1998). It is well known that the rate-limiting enzyme in the formation of melanin is tyrosinase (TYR), which is responsible for several oxidative steps in the synthesis of the melanin (Parvez et al. Citation2006, Olivares et al. Citation2009). In our study, TYR showed a significantly higher expression level in the skin of Taihe silky fowl, which is inconsistent with studies of sheep coat colour (Fan et al. Citation2013, Norris et al. Citation2008). MITF promoted a continuous transcription of the tyrosinase gene under normal circumstances and after hormonal stimulation through the cAMP signalling (Galibert et al. Citation2001). Considering the key regulatory role of MITF in the expression levels of tyrosinase genes, we think it was due to the up-regulation of MITF expression level resulted in the up-regulation of TYR expression level.
Interestingly, we found that the MITF, TYR and TYRP1 were differentially expressed in the liver of Taihe silky fowl and Chishui silky fowl. In the liver, the expression levels of MITF and TYR showed consistent trends, whereas the expression level of TYRP1 in the Taihe silky fowl’s rooster was significantly decreased. In the white silky fowl, Zheng et al. (Citation2015) found the expression of MITF and TYR was significantly lower than other tissues in the liver, but the ASIP was the opposite. Wang et al. (Citation2018) found that MITF expression level was highest in the liver of the Muchuan black-boned chickens and MITF promoter polymorphisms affected chicken skin colour. On the contrary, Kriangwanich et al (Citation2021) and Nganvongpanit et al (Citation2020) found that hyperpigmentation was not observed in the liver, which is inconsistent with the present study. Consistently, our lightness value of the liver showed a significant difference between Taihe silky fowl and Chishui silky fowl, but there was no difference in the melanin content. In the Silky Fowl chickens, the melanocytes present in the internal organs of stage III melanosomes (the intermediate phase of melanosome production associated with deposits of melanin during the production of matrix proteins) produce and maintain melanosomes inside the cells (Ortolani-Machado C F et al. Citation2009). Besides, pigmentation is known to occur in black-bone chickens via two different migration pathways: the dorsolateral and ventral pathways (Reedy M V et al.Citation1998). It still needs further investigation in the future study whether the differential expression of pigmentation-related genes was induced by the differential migration pathways between Taihe silky fowl and Chishui silky fowl. More importantly, the fact that the differential expression of pigmentation-related genes in the liver between the Taihe silky fowl and Chishui silky fowl is of particular interest for future studies that aim to elucidate their functional roles in the regulation of internal organ melanin deposition.
Conclusion
In summary, this study reported the pigmentation difference in the four tissues of the Taihe silky fowl and Chishui silky fowl by qualitative and quantitative methods. Moreover, our comparative analysis revealed that four tissues or organs of Taihe silky fowl and Chishui silky fowl have different expression levels of pigmentation-related genes in the skin and liver. However, their black degree of liver and chest muscle, and the melanin content of leg muscle showed a slight difference among them. This study provided vital information on the black degree of tissues and the melanin content in Chishui silky fowl. Compared with Taihe silky fowl, Chishui silky fowl showed equivalent pigmentation in the skin, chest muscle and leg muscle, and liver. It also provided a valuable theoretical basis for future breeding schemes for Chishui silky fowl and is highly preferable by consumers.
Acknowledgments
The authors wish to acknowledge members of our laboratory, and Yinlian Chen member of Houqiang Xu Laboratory for his technical support and suggestion about the experiment.
Disclosure statement
No potential conflict of interest was reported by the author(s).
Additional information
Funding
References
- Ando H, Kondoh H, Ichihashi M, Hearing VJ. 2007. Approaches to identify inhibitors of melanin biosynthesis via the quality control of tyrosinase. J Invest Dermatol. 127(4):751–761.
- Denecker G, Vandamme N, Akay O, Koludrovic D, Taminau J, Lemeire K, Gheldof A, De Craene B, Van Gele M, Brochez L, et al. 2014. Identification of a ZEB2-MITF-ZEB1 transcriptional network that controls melanogenesis and melanoma progression. Cell Death Differ. 21(8):1250–1261.
- Dereure O. 2001. Drug-induced skin pigmentation. Epidemiology, diagnosis and treatment. Am J Clin Dermatol. 2(4):253–262.
- Duffy DL, Zhao ZZ, Sturm RA, Hayward NK, Martin NG, Montgomery GW. 2010. Multiple pigmentation gene polymorphisms account for a substantial proportion of risk of cutaneous malignant melanoma. J Invest Dermatol. 130(2):520–528.
- Fan R, Xie J, Bai J, Wang H, Tian X, Bai R, Jia X, Yang L, Song Y, Herrid M, et al. 2013. Skin transcriptome profiles associated with coat color in sheep. BMC Genomics. 14(1):1–12.
- Galibert MD, Carreira S, Goding CR. 2001. The Usf-1 transcription factor is a novel target for the stress-responsive p38 kinase and mediates UV-induced tyrosinase expression. EMBO J. 20(17):5022–5031.
- Graham A, Wakamatsu K, Hunt G, Ito S, Thody AJ. 1997. Agouti protein inhibits the production of eumelanin and phaeomelanin in the presence and absence of alpha-melanocyte stimulating hormone. Pigment Cell Res. 10(5):298–303.
- Han D, Wang S, Hu Y, Zhang Y, Dong X, Yang Z, Wang J, Li J, Deng X. 2015. Hyperpigmentation results in aberrant immune development in silky fowl (Gallus gallus domesticus Brisson). PLoS One. 10(6):e0125686.
- Hart KL, Kimura SL, Mushailov V, Budimlija ZM, Prinz M, Wurmbach E. 2013. Improved eye- and skin-color prediction based on 8 SNPs. Croat Med J. 54(3):248–256.
- Hoekstra HE. 2006. Genetics, development and evolution of adaptive pigmentation in vertebrates. Heredity (Edinb). 97(3):222–234.
- Hsieh P-H, Lien T-F. 2012. Study of the physico-chemical properties and antioxidant activity of extracted melanins. J Agri Sci. 4(9):217–229.
- Ito S. 2006. Encapsulation of a reactive core in neuromelanin. Proc Natl Acad Sci USA. 103(40):14647–14658.
- Ito S, Fujita K. 1985. Microanalysis of eumelanin and pheomelanin in hair and melanomas by chemical degradation and liquid chromatography. Anal Biochem. 144(2):527–536.
- Jablonski NG, Chaplin G. 2010. Colloquium paper: human skin pigmentation as an adaptation to UV radiation. Proc Natl Acad Sci USA. 107(2):8962–8973.
- Kobayashi T, Imokawa G, Bennett DC, Hearing VJ. 1998. Tyrosinase stabilization by Tyrp1 (the brown locus protein). J Biol Chem. 273(48):31801–31805.
- Kriangwanich W, Piboon P, Sakorn W, Buddhachat K, Kochagul V, Pringproa K, Mekchay S, Nganvongpanit K. 2021. Consistency of dark skeletal muscles in Thai native black-bone chickens (Gallus gallus domesticus). PeerJ. 9:e10728.
- Li D, Wang X, Fu Y, Zhang C, Cao Y, Wang J, Zhang Y, Li Y, Chen Y, Li Z, et al. 2019. Transcriptome analysis of the breast muscle of xichuan black-bone chickens under tyrosine supplementation revealed the mechanism of tyrosine-induced melanin deposition. Front Genet. 10:457.
- Li Y, Zhu X, Yang L, Li J, Lian Z, Li N, Deng X. 2011. Expression and network analysis of genes related to melanocyte development in the silky fowl and white leghorn embryos. Mol Biol Rep. 38(2):1433–1441.
- Mackintosh JA. 2001. The antimicrobial properties of melanocytes, melanosomes and melanin and the evolution of black skin. J Theor Biol. 211(2):101–113.
- Minvielle F, Bed'hom B, Coville JL, Ito S, Inoue-Murayama M, Gourichon D. 2010. The “silver” Japanese quail and the MITF gene: causal mutation, associated traits and homology with the “blue” chicken plumage. BMC Genet. 25:1–8.
- Nan H, Kraft P, Hunter DJ, Han J. 2009. Genetic variants in pigmentation genes, pigmentary phenotypes, and risk of skin cancer in Caucasians. Int J Cancer. 125(4):909–917.
- Nganvongpanit K, Kaewkumpai P, Kochagul V, Pringproa K, Punyapornwithaya V, Mekchay S. 2020. Distribution of melanin pigmentation in 33 organs of Thai black-bone chickens (Gallus gallus domesticus). Animals (Basel). 10(5):777.
- Norris BJ, Whan VA. 2008. A gene duplication affecting expression of the ovine ASIP gene is responsible for white and black sheep. Genome Res. 18(8):1282–1293.
- Olivares C, Solano F. 2009. New insights into the active site structure and catalytic mechanism of tyrosinase and its related proteins. Pigment Cell Melanoma Res. 22(6):750–760.
- Oribe E, Fukao A, Yoshihara C, Mendori M, Rosal KG, Takahashi S, Takeuchi S. 2012. Conserved distal promoter of the agouti signaling protein (ASIP) gene controls sexual dichromatism in chickens. Gen Comp Endocrinol. 177(2):231–237.
- Ortolani-Machado CF, Freitas PF, Faraco CD. 2009. Melanogenesis in dermal melanocytes of Japanese silky chicken embryos. Tissue Cell. 41(4):239–248.
- Ozeki H, Ito S, Wakamatsu K, Thody AJ. 1996. Spectrophotometric characterization of eumelanin and pheomelanin in hair. Pigment Cell Res. 9(5):265–270.
- Parvez S, Kang M, Chung HS, Cho C, Hong MC, Shin MK, Bae H. 2006. Survey and mechanism of skin depigmenting and lightening agents. Phytother Res. 20(11):921–934.
- Peñagaricano F, Zorrilla P, Naya H, Robello C, Urioste JI. 2012. Gene expression analysis identifies new candidate genes associated with the development of black skin spots in Corriedale sheep. J Appl Genet. 53(1):99–106.
- Reedy MV, Faraco CD, Erickson CA. 1998. Specification and migration of melanoblasts at the vagal level and in hyperpigmented Silkie chickens. Dev Dyn. 213(4):476–485.
- Robbins LS, Nadeau JH, Johnson KR, Kelly MA, Roselli-Rehfuss L, Baack E, Mountjoy KG, Cone RD. 1993. Pigmentation phenotypes of variant extension locus alleles result from point mutations that alter MSH receptor function. Cell. 72(6):827–834.
- Serbedzija GN, Bronner-Fraser M, Fraser SE. 1989. A vital dye analysis of the timing and pathways of avian trunk neural crest cell migration. Development. 106(4):809–816.
- Shah M, Bhoumik A, Goel V, Dewing A, Breitwieser W, Kluger H, Krajewski S, Krajewska M, Dehart J, Lau E, et al. 2010. A role for ATF2 in regulating MITF and melanoma development. PLoS Genet. 6(12):e1001258.
- Slominski A, Tobin DJ, Shibahara S, Wortsman J. 2004. Melanin pigmentation in mammalian skin and its hormonal regulation. Physiol Rev. 84(4):1155–1228.
- Sturm RA, Duffy DL. 2012. Human pigmentation genes under environmental selection. Genome Biol. 13(9):248–253.
- Sulem P, Gudbjartsson DF, Stacey SN, Helgason A, Rafnar T, Magnusson KP, Manolescu A, Karason A, Palsson A, Thorleifsson G, et al. 2007. Genetic determinants of hair, eye and skin pigmentation in europeans. Nat Genet. 39(12):1443–1452.
- Tian YG, Xie MY, Wang WY, Wu HJ, Fu ZH, Lin L. 2007. Determination of carnosine in black-bone silky fowl (Gallus gallus domesticusBrisson) and common chicken by HPLC. Eur Food Res Technol. 226(1-2):311–314.
- Tu YG, Sun YZ, Tian YG, Xie MY, Chen J. 2009. Physicochemical characterisation and antioxidant activity of melanin from the muscles of taihe black-bone silky fowl (Gallus gallus domesticus brisson). Food Chem. 114(4):1345–1350.
- Vachtenheim J, Borovanský J. 2010. “Transcription physiology” of pigment formation in melanocytes: central role of MITF. Exp Dermatol. J. 19(7):617–627.
- Wang G, Liao J, Tang M, Yu S. 2018. Genetic variation in the MITF promoter affects skin colour and transcriptional activity in black-boned chickens. Br Poult Sci. 59(1):21–27.
- Yang C, Ge J, Chen S, Liu Y, Chen B, Gu Z. 2015. Sequence and gene expression analysis of the agouti signalling protein gene in Rex rabbits with different coat colours. Ital J Anim Sci. 14(3):563–565.
- Zhang J, Liu F, Cao J, Liu X. 2015. Skin transcriptome profiles associated with skin color in chickens. PLoS One. 10(6):e0127301.
- Zheng NZ, Li LI, Xin QW, Miao ZW, Zhu ZM, Chen L. 2015. Genetic effect of tyrosinase(TYR), microphthalmia- associated transcription factor(MITF) and agouti signaling protein(ASIP) genes on melanin deposition of white Silky Fowl (Gallus gallus domesticus Brisson). J Agri Biotech. 23(8):1076–1083. (in Chinese).