ABSTRACT
Microbiota is an integral component of the intestinal tract and plays a significant role in health status of fish. However, diet and feeding habits are the key factors affecting the intestinal microbiota. Here, a 90-day feeding trial was conducted to investigate the effects of a plant-based β-mannanase supplemented diet on the growth, gut microbiome, and mRNA level of key digestion and immunity-related genes of common carp (Cyprinus carpio). Fish were evenly distributed in 3 groups: control, A1 and A2, and fed 35% CP supplemented with β-mannanase at the rate of 0, 500, and 1000 units/kg diet respectively. At the end of the feeding trial, the intestinal microbiota was profiled by sequencing the v4 region of bacterial 16S rRNA and internal transcribed spacer (ITS) regions of fungal 18S rRNA. Results indicated improved growth performance, changes in richness and diversity of intestinal microbiota and up-regulation of intestinal digestion (Amy, Lip, Tryp, FAS, FABP), and immunity-related (SOD, NK-lys, Def, Lys, IL1-β) genes of A1 and A2 groups of fish as compared to control. This study first time demonstrates that β-mannanase in a plant-based diet could improve the intestinal health of C. carpio via modulation of gut microbiota and up-regulation of host digestion and immunity-related genes.
1. Introduction
In aquaculture feeds, fishmeal is the most prevalent and best source of protein. It has a high content of crude protein (65–72%), as well as all the ten necessary amino acids, essential fatty acids, some vitamins, and minerals (Tacon et al. Citation2009; Samaddar et al. Citation2015). However, for the past decade due to growing demand for fish meal in both livestock and aquaculture industry as well as a drop in its worldwide production and supply has made fish meal the most costly source of protein for fish feeds (El Sayed Citation1998). Furthermore, fishmeal contains significantly more phosphorus than is required for optimal fish development, resulting in the discharge of excess phosphorus into the environment causing eutrophication (Kalhoro et al. Citation2018). Because of these issues, replacing fishmeal with alternative sources of protein that function as well as or nearly as well as fishmeal is the current priority in the aquaculture industry.
At present, among different alternatives to fish meal, plant-based feedstuffs appear to be readily available and least expensive. Currently, worldwide cereal grain production is at 2790 million tonnes, legume production is at 150 million tonnes, and fibrous material production is about 115 million tons (FAO Citation2020). Although the carbohydrate content of grains and legumes provides a cheap source of energy for fish, most fish species do not use it as efficiently as protein and lipids (Turchini et al. Citation2019). Furthermore, plant-based feeds such as rapeseed meal, soybean meal, corn glutton meal, root tuber meal, sunflower meal, and legume seeds have some anti-nutritional factors such as protease inhibitors, tannins, saponins, phytates, lectins, and non-starch polysaccharide (NSP), which negatively affect digestion and fish growth (Francis et al. Citation2001).
Non-starch polysaccharides (NSP) are one of the major anti-nutritional compounds in plant-based feed, which are present in the cell wall as complex carbohydrates. They are responsible for increasing digesta viscosity, changes in morphology and physiology of the intestine, and alteration of the intestinal microbiota (Chen et al. Citation2016). However, it has not been reported that NSP directly inhibits digestive enzymes, but their activities might be affected due to increased digesta viscosity caused by NSP in the feed, thus restricting the enzyme to access the substrate (Sinha et al. Citation2011).
Among NSP mannan appear in the form of Glucomannan and Galactomannan. Mannan present in soybean meal, cottonseed meal, rapeseed meal, and corn gluten meal has shown to be a strong anti-nutritive factor in various monogastric animals and fish species (Wu et al. Citation2005). Therefore, there is an increasing interest in the exploration of feed additives that could neutralize the anti-nutritional effects of mannan in the feed. Recent studies have shown that β-Mannanase can hydrolyze mannan present in the feed to manno-oligosaccharides (MOS), which act as prebiotics (Huang et al. Citation2003; Wu et al. Citation2005). For instance, the positive effects of dietary β-mannanase supplementation on growth performance and biological functioning of turkeys (Odetallah et al. Citation2002) laying hens (Jackson et al. Citation1999) broilers (Mussini et al. Citation2011), and swine (Pettey et al. Citation2002) have already been reported. However, there have been very few reports about the effect of β-mannanase supplementation on fish (Chen et al. Citation2016), and no study has been reported about its effect on intestinal health.
The intestine of an omnivorous fish like common carp (Cyprinus carpio) harbours a diverse community of microbes, plays an important role in digestion, and is often exposed to foreign agents present in food like microorganisms and toxic substances. The gut microbiota of fish plays a very important role in the nutrient digestion and maintenance of fish health (Chakrabarti and Sharma Citation2014). Ringø and Olsen (Citation1999) suggested that dietary changes can affect the gut microbiota composition. Nevertheless, the relationship between β-mannanase supplementation and fish gut microbiota has not been explored yet. Studies have reported that a diet high in NSP may lead to increased production of organic acids like acetic acid, which lowers the intestinal pH and thus upsets the microbial ecosystem in the gut (Sinha et al. Citation2011). Gastric acidity can also decrease the mineral bioavailability, mainly by varying the mechanism through which minerals are transported. In addition, NSP intake in feed increases the residence time of digesta, which may lower oxygen tension and thus create a suitable environment for the proliferation of anaerobic microorganisms (Choct Citation1997). Although it has not been clearly established that a sudden change in gut microbiota balance can hurt the efficiency of nutrient utilization, but many investigators have reported the importance of normal endogenous gut microbiota for the health status of fish (Sinha et al. Citation2011). Thus, it’s of utmost importance to investigate the effect of a feed additive on the microbiota of the gut.
Digestive enzymes like trypsin (Tryp), lipase (Lip), and amylase (Amy) play an important role in digestion and absorption of nutrients which in turn affects fish growth. Fatty acid-binding protein (FABP) and Fatty acid synthase (FAS) are important for lipid metabolism and biosynthesis (Yang et al. Citation2011). Lysozyme (Lys) is a part of the innate immune system of fish. It protects the fish from the attack of pathogenic bacteria by breaking the linkages in the peptidoglycan of the bacterial cell wall. This breakage of the bacterial cell wall weakens the integrity of bacteria leading eventually to its lysis (Zheng et al. Citation2016). Defensin (Def) in fish, are peptides with antimicrobial activity. They act as microbicidal agents and prevent attacks from different pathogenic microorganisms. Nk-lysin (Nk-lys) has broad antimicrobial activity against fungi, bacteria, protozoa, and parasites. Superoxide dismutase (SOD) is a key antioxidant enzyme and protects the fish from oxidative stress and reactive oxygen-mediated diseases. IL1-β is an important immunity-related mucosal gene. Thus, these digestive and immune-related indices can be used to study the effect of mannanase supplementation on fish intestinal health regulation.
Studies conducted on supplementation of exogenous carbohydrase enzyme in fish feed usually focus on the effect of the enzyme on growth performance, digestibility, and a few immunological indices. No study has assessed the effect of a carbohydrase enzyme on the intestinal health of fish which is very important information about a feed additive. In the present study, we supplemented β-mannanase enzyme as a feed additive to common carp and studied its effect on intestinal health in terms of growth, digestion and immune-related gene expression, and gut microbiota. The study aims to provide novel data that would contribute towards the sustainability of aquaculture by an understanding of the role of β-mannanase as a feed additive in plant-based fish feed. As a representative of the omnivorous fish, the information on dietary β-mannanase supplementation in C. carpio will provide references for other fish.
2. Materials and methods
2.1. Ethics statement
All experimental procedures were approved by the Quaid-I-Azam University’s animal welfare committee in compliance with local legislation (BEC-FBS-67-QAU-2019).
2.2. Fish and culture conditions
Healthy Juvenile C. carpio with no sign of infection was bought from a commercial Fish farm and transferred to QAU Fisheries and Aquaculture research station. The fish were stocked in a concrete raceway and acclimatized for a week. Feeding was done twice a day to apparent satiation.
2.3. Preparation of diet
A plant-based 35% crude protein diet was formulated. Its formulation and proximate composition are shown in . All ingredients used for the preparation of feed were purchased from a local feed mill (Oryza Organics Pvt, Ltd). The locally isolated β-mannanase having 78U/ml activity at 37°C and pH 6.0 was isolated from Aspergillus niger AD-01 in our lab. The detailed procedure of isolation and purification has been reported earlier (Dawood et al. Citation2019). To obtain a 35% crude protein diet, all feed ingredients were mixed in a fixed ratio. Dry ingredients were made into the dough by mixing them with water and oil and then passed through a meat grinder. The resulting noodles were cut and air-dried at low temperatures. The prepared feed was divided into three dietary treatment groups (A1, A2, and control). For β-mannanase supplementation, the proper amount of enzyme was dissolved in distilled water and sprayed on treatment groups at the rate of 1000, 500, and 0 units/kg, respectively. The feed was dried once again. The pellets were transferred to a Ziploc bag and stored in the refrigerator.
Table 1. Formulation and proximate composition of 35% crude protein basal diet.
2.4. Experimental design and feeding trial
A completely randomized experiment was designed and conducted in replicates of four under controlled conditions. Fish having an initial weight of 12.32 ± 0.11 g were randomly selected and distributed into 12 circular fibreglass tanks (250 L) at the rate of 1.5 g/L (15 fish per tank). Each tank was well equipped with a heater and air stones connected to the air pump for maintaining the temperature at 22°C and DO level at 5.5 ± 0.5 mg/L, respectively. To prevent the fish from jumping out, the top of each tank was covered with a net. After acclimatization to experimental conditions for three days, fish were divided into three groups (A1, A2, and control). Subsequently, the feeding trial was started and fish in each group were fed their respective diet at 4% of body weight twice (8:00 am and 4:00 pm) a day (Inayat and Salim Citation2005). The fish in each tank were weighed after every 14 days using an electronic balance (Shimadzu BL2200H) and the feeding rate was adjusted accordingly. To maintain optimum water quality, one-third water of each tank was renewed daily. Furthermore, uneaten feed and feces were also removed. The feeding trial lasted for 12 weeks.
2.5. Percentage survival and growth
A day before the end of the experiment, feeding was restricted and on the day of sampling, all fish of each tank were collected separately and weighed for the evaluation of survival and growth performance. The survival (%) and growth performance in terms of, specific growth rate (SGR), percent weight gain (WG%), increase in average weight of fish, protein efficiency ratio (PER) and feed conversion ratio (FCR) were determined by using standard formulas reported by Ibrar et al. (Citation2017).
2.6. Sampling
At the end of the 90-day feeding experiment, six fish from each tank were randomly sampled and anesthetized with Tricaine methane sulphonate (MS-222) solution prepared in NAHCO3 buffer (0.10 gL−1). The fish were dissected at low temperature by following the standard aseptic method. The whole intestine of each fish was dissected out carefully with sterile scissors and forceps, snap-frozen in liquid nitrogen, and stored at −80°C for the study of gene expression and composition of intestinal microbiota.
2.7. Gene expression analysis
RNA was extracted from the intestine of 3 fish per tank, with buffer RLT (Qiagen, Mississauga, Canada) and 0.12 m β-mercaptoethanol (Sigma). An RNeasy Protect Mini kit (Qiagen Inc., U.S.A.) was used according to the manufacturer’s instructions to purify the extracted RNA. The quality of RNA was checked by using 1.0% agarose gel electrophoresis and RNA samples were quantified by spectrophotometry at 260 nm by using Nanodrop-1000 spectrophotometer (Thermo Scientific, U.S.A.). For the synthesis of first-strand cDNA, total RNA (8.0 μg) was reverse transcribed by using Invitrogen SuperScriptTM IV Reverse Transcriptase kit (Thermo Fisher Scientific, U.S.A.), following the manufacturer’s instructions. Real-time RT-qPCR was run using an SYBR Premix ExTagTM II kit (TaKaRa, Japan) with a LightCyler 480 system. Specific primer sequences (listed in ) of target genes were designed using the primer blast tool and RT-qPCR was run by following the method reported by Duan et al.(Citation2017) for investigation of the expression of digestion and immune-related genes including, Amy, Tryp, Lip, FAS, FASB, SOD, Lys, Def, NK-lys, and IL-β. For the calibration of the cDNA template and confirmation of successful reverse transcription, the β-actin gene of common carp was chosen as an internal control. 2−ΔΔCT comparative CT method was used to calculate relative gene expression, and shown as a fold change in expression, in comparison to the control group.
Table 2. Primer sequences used in this study.Table Footnotea
2.8. Fish gut microbiome analysis
Intestinal DNA was extracted from 3 fish per tank, using DNeasy PowerSoil®Kit (Qiagen Inc., U.S.A.) according to manufacturers’ instructions. The extracted DNA samples were qualitatively analyzed in 1.0% agarose gel electrophoresis and then quantity was determined at 260 nm by using a Nanodrop 1000 spectrophotometer (Thermo Scientific, U.S.A.). To generate a DNA library, the extracted DNA samples were subjected to PCR. The v4 region of bacterial 16S rRNA gene and internal transcribed spacer (ITS) regions of fungal 18S rRNA gene were amplified by using 515F (GTGCCAGCMGCCGCGGTAA)/806R (GGACTACHVGGGTWTCTAAT) and ITS2 primer pairs (ITS-3F, 5′-GCATCGATGAAGAACGCAGC-3′; ITS-4R, 5′-TCCTCCGCTTATTGATATGC-3′) respectively (Moncada et al. Citation2013; Walters et al. Citation2016). HotStarTaq Plus Master Mix Kit (QIAGEN, U.S.A.) was used to perform the PCR program. The PCR reaction conditions were initial denaturation at 95°C for 5 min, followed by 27 cycles of denaturation at 95°C for 30 s, 55°C for 30 s, and 72°C for 45 s. The final step was carried out at 72°C and lasted for 10 min. For the determination of length differences, the PCR fragments were subjected to 2.0% agarose gel electrophoresis. A PCR purification kit (QIAquick PCR Purification Kit) was used to purify the target bands. The purified PCR product was pooled and subjected to sequencing by Macrogen, U.S.A. using Illumina Miseq platform according to the manufacturer’s instructions. The resulting sequences were processed and analyzed using the Bioinformatics package QIIME, version 2.0. For this, the raw data was imported into QIIME and the sequences for each sample were demultiplexed using the q2-cutadapt plugin. All the primer sequences and barcodes were removed. The sequences were also denoised and merged following the recommended protocol (Hall and Beiko Citation2018). The resulting reads were clustered into operational taxonomic units (OTUs), defined at different similarity cut-off levels. The taxonomic classification of the final OTUs was done by using BLAST against a curated database derived from RDPI, RDPII, and NCBI.
2.9. Statistical analysis
GraphPad prism5 software was used for statistical analysis. One-way analysis of variance (ANOVA) followed by Bonferroni’s multiple range test was used to carry out a comparison among treatments. Microbial diversity was assessed among samples using OTUs, Simpson, and Shannon indices. Significance was set at P < 0.05.
3. Results
3.1. Fish growth parameters
After 12 weeks feeding trial, One-way ANOVA indicated that the carp fed β-mannanase enriched diet showed a significantly higher increase in the final weight, SGR, PER, and lower FCR compared to control (P < 0.05; ). However, the observed parameters did not show any significant difference between treatment groups (A1 and A2).
Table 3. Effect of β-Mannanase supplementation on growth parameters of carp after 12 week feeding trial (means ± SEM).*
3.2. Expression levels of intestine immunity-related genes
The relative expression of immune-related genes, including NK-lys, Def, IL1-β, Lys and SOD increased in both treatment groups A1 and A2 compared to the control group (P < 0.05) (). However, there was no significant difference between antioxidant-related genes (SOD, Lys) between treatment groupA1 and groupA2 (P > 0.05). However, the expression of the mucosal immunity-related gene (IL1-β) and antimicrobial genes (Def, NK-lys) increased in a dose-dependent manner and thus was highest in the A2 group (P < 0.05).
Figure 1. Expression level of immunity-related genes in the intestine of common carp fed control and two different doses (500 &1000 units/kg) of mannanase supplemented diet for 90 days. The reference gene is β-actin. Each bar represent data as mean ± SE (N = 3). Bars marked with different letters have significant differences (P < 0.05).
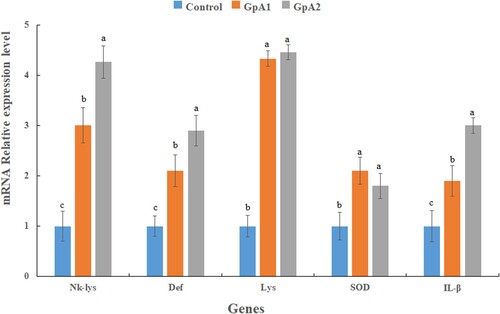
3.3. Expression levels of intestine digestion-related genes
The relative expression level of all the five genes related to digestion of nutrients, including Amy, Lip Tryp, FAS, and FABP was increased in the treatment groups (A1 and A2) as compared to control (P < 0.05) (). The highest expression level of all the five genes was detected in group A2. There was a significant difference (P < 0.05) between the expression level of the genes Amy and FAS in group A2 as compared to group A1. But no significant differences were observed in the expression level of Tryp, Lip, and FABP genes between groups A1 and A2 (P > 0.05).
Figure 2. Expression level of digestion related genes in the intestine of common carp fed control and two different doses (500 &1000 units/kg) of mannanase for 90 days. The reference gene is β-actin. Vertical bars represent the mean ± SE (N = 3). Bars marked with different letters have significant differences (P < 0.05) among groups.
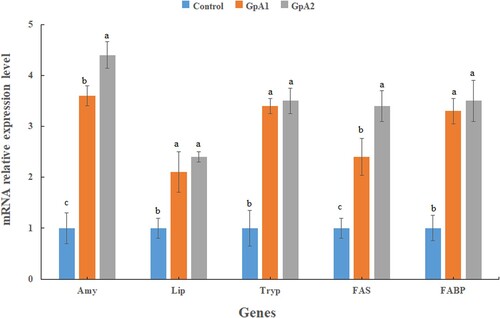
3.4. Fish gut microbial analysis
3.4.1. Richness and diversity
DNA was extracted from 3 fish per tank (12 per treatment group). However, a few samples showed poor PCR amplification and thus were discarded, leaving 10 samples per treatment group for Illumina Miseq sequencing. After optimization and filtration of the raw data, a total of 2,524,849 high-quality sequencing reads were obtained from 60 samples with an average of 42,080 per sample. The total number of observed OTUs ranged from 81 to 169. Diversity analysis in terms of Simpson (alpha diversity) and Shannon (beta diversity) indices was increased in the treatment groups (A1 and A2) compared to control ().
Table 4. Diversity measure of intestine microbial used in the study.
3.4.2. Genera and phyla performances
Six phyla were detected in the control group: Proteobacteria (81.05%), Actinobacteria (12.6%), Bacteroidetes (4.21%), Firmicutes (1.03%), Planctomycetes (0.68%), and Chlamydia (0.0034%). Dominating bacterial genera were Pseudomonas (74.27%), Streptomyces (9.08%), Desulfobulbus (1.78%) and Haliobacteriovorax (0.8%) ((a)). The control group showed five fungal phyla: Ascomycota (3.02%), Basidiomycota (96.28%) Glomeromycota (0.0085%), Neocallimastogomycota (0.0064%) and Cryptomycota (0.002%). Dominating fungal genera were Debaryomyces (80.23%) Cryptococcus (5.25%) and Malassezia (3.98%) ((b)).
Metagenomic analysis revealed that A1 group had 7 phyla Proteobacteria (91.7%), Firmicutes (3.06%), Actinobacteria (2.88%) Cyanobacteria (0.95%), Planctomyces (0.78%) Bacteroidetes (0.0456%) and Spirochete (0.0031%). At the genus level, dominating bacteria were Achromobacter (60.03%) Klebsiella (28.2%) and Lactococcus (3.02%) ((a)). Dominating fungal phyla in group A1 were Ascomycota (80.06%), Basidiomycota (19.02%) and Cryptomycota (0.0056%) ((b)). Dominating fungal genera were Galactomyces (42.75%) Geotrichum (26.31%) and Cryptococus (16.78%). However, group A2 was represented by six phyla Proteobacteria (96.08%), Firmicutes (3.29%), Fusobacteria (0.0078%), Actinobacteria (0.0051%), Planctomycetes (0.0044%) and Bacteroidetes (0.0034%). Dominating bacterial genera were Pseudomonas (81.56%), Serratia (11.23%) Lactobacillus (1.50%), Lachnospira (1.34%) and Bacillus (0.45%) ((a)). Among fungal phyla, three phyla were dominant Ascomycota (97.05%) Basidiomycota (2.08%) and Glomeromycota (0.08%). Dominating fungal genera were Galactomyces (76.78%) Geotrichum (9.97%) Knufia (2.76%) and Debaryomyces (1.73%) ((b))
4. Discussion
The intestine of an omnivorous fish like C. carpio is exposed to a variety of feeding materials. It possesses a large surface area and acts as a barrier against pathogen-caused inflammation and infection. In animals, the intestine has structural integrity and hosts immune proteins in addition to a stable microbiome (Duan et al. Citation2017). The diversity of the gut microbiota has a considerable impact on the health and growth performance of the host. The dysbiosis in gut microbiota can negatively affect host physiology and overall wellbeing. Among different factors, feeding can also alter the gut microbial composition (Rajoka et al. Citation2017). In the present study, supplementation of mannanase enzyme in plant-based fish feed influenced the gut microbiota, immune and digestion-related genes in C. carpio. Illumina Miseq sequencing of gut microbiota of carp fed different doses of mannanase enzyme showed that supplementation with the enzyme altered its intestinal microbiota composition.
The metagenomic analysis revealed the presence of Protobacteria and Firmicutes as dominant bacteria in the gut of C. carpio of all groups. These bacteria make up the dominant phyla in the gut of most fish. It has been reported that Firmicute bacteria provide a good measure of intestinal health status. For example, Lactococcus, Bacillus, and Lactobacillus can not only provide protection against pathogen-induced intestinal function disruption but can also prevent inflammatory cytokines from being produced (Zheng et al. Citation2017), while Lachnospiraceae has been reported to take part in the fermentation of carbohydrates and production of short-chain fatty acids (SCFAs) and gasses (H2 and CO2) (Duncan et al. Citation2007). In the present study, dietary mannanase supplementation increased the growth of beneficial bacteria of phylum Firmicutes like Bacillus, Lactobacillus, and Lachnospira in both treatment groups.
Furthermore, some opportunistic pathogens like Aeromonas, Desulfobulbus and Desulfovibrio were not observed in the mannanase supplemented groups. Desulfobulbus and Desulfovibrio are the chief sulfate-reducing bacteria and are ubiquitously present in both animals and humans (Ichiishi et al. Citation2010). The H2S gas produced by these sulfate-reducing bacteria can damage the intestine epithelial cells. Thus, their absence in the treatment groups may indicate a reduced risk of opportunistic pathogen invasion in C. carpio. Bacterial diseases like Vibriosis are a major constraint affecting the sustainability and production of the aquaculture industry. The risk of the disease is especially high when the fish is under stress. We did not find Vibrio spp. in both the treatment groups which might be due to supplementation of feed with a carbohydrase enzyme-like β-mannanase that reduces the residence time of digesta in the gut of fish. An increase in residence time of digesta has been associated with the decrease in oxygen tension which may favour the growth of opportunistic pathogens like Vibrio and Aeromonas (Sinha et al. Citation2011). However, supplementation with carbohydrase enzyme reverses these effects and may prevent opportunistic pathogens to invade. Interestingly, we did not find Vibrio spp. in the control group. The absence of Vibrio might be due to the presence of Halobacteriovorax that might suppress its growth (Williams et al. Citation2016). This phenomenon needs to be investigated further.
Metagenomic analysis of 18S rRNA of fish gut microbiota showed an abundance of Geotrichum spp. in both the treatment groups. Geotrichum is a part of normal human flora and infections from Geotrichum in animals are rare (Carter and Cole Citation2012). It has been reported that Geotrichum has broad-spectrum antibacterial activity against pathogenic microorganisms such as E. coli, S. aureus, and Micrococcus spp. (Mefteh et al. Citation2017). Geotrichum has been used as a probiotic in fish feed and its beneficial effects on growth, immunity, and resistance against disease have been demonstrated in different studies (Amir et al. Citation2018, Citation2019; Ghori et al. Citation2018).
The enhanced relative expression of immune-related genes, including, Lys, SOD, NK-lys, Def, and IL1-β in the present study also indicated the positive effect of dietary β- mannanase supplementation. The beneficial effects of dietary carbohydrase enzymes including xylanase and mannanase on the immunity of fish have been reported in other studies (Chen et al. Citation2016; Saputra et al. Citation2016). However, no study has reported mannanase effect on the expression of immune-related genes. β-mannanase enzyme when supplied in the feed hydrolyses the mannan into manno-oligosachrides (MOS), which act as prebiotics (Ringø and Olsen Citation1999; Huang et al. Citation2003). Many investigators have reported modulation of gut microbiota by MOS i.e. favouring the growth of certain bacteria while inhibiting proliferation of others (Wang et al. Citation2018; Zheng et al. Citation2018). The increased expression level of immunity-related genes in the present study might be due to changes in gut microbiota composition of C. carpio. Several studies have reported the causal link between gut microbiota and immune gene expression (Rupnik et al. Citation2009; Nie et al. Citation2017). Good evidence of this causal relationship has been provided by a study conducted on a mouse infected with Clostridium difficile (Rupnik et al. Citation2009). It seems that some factors caused by specific gut microorganisms might influence the immune gene expression of the host, which in turn plays an important role in resistance towards disease in an animal (Nie et al. Citation2017). According to some investigators, SCFAs generated by beneficial bacteria such as Bacillus, Lactobacillus and Lachnospira can enter the pathogenic bacterial cell wall, release its protons (H+), and disrupt bacterial cell metabolism, resulting eventually in bacterial cell death. (De Schryver et al. Citation2010; Meehan and Beiko Citation2014; McLoughlin et al. Citation2017). These mechanisms reveal that mannanase may have played a role in not only improving the host immunity by a selective proliferation of beneficial microorganisms in the treatment groups but also contributed towards inhibition of the pathogenic bacteria by increasing the SCFAs contents in the intestines of C. carpio.
β-Mannanase supplementation also led to an increase in the relative expression level of digestion-related genes, including Amy, Lip, Tryp, FAS, FABP in both the treatment groups compared to control. Similar results have been reported by other investigators supplementing other carbohydrase enzymes to different fish species. Zhou et al. (Citation2013) report that supplementation of cellulase enzyme in the duckweed-based diet increased the digestive enzyme activities of grass carp (Ctenopharyngodon idella). An increase in the intestinal enzyme activities following supplementation of feed with xylanase in Jian carp (Cyprinus carpio var. Jian) has also been reported (Jiang et al. Citation2014). This shows that exogenous enzyme supplementation can promote the production of endogenous enzymes. The consumption of a diet rich in NSP, changes the anatomy and physiology of the gut of the monogastric animal, mainly by changing the digesta viscosities. This leads to an increased residence time of digesta which may alter the pH of the intestine and disturb the microbial ecology within the gut (Sinha et al. Citation2011). Supplementation with β-mannanase may reverse these negative effects. In addition, the prebiotics (MOS) produced as a result of mannanase action promote selective growth of beneficial microorganisms (Markowiak and Śliżewska Citation2017; Zhang et al. Citation2021). These beneficial microorganisms or probiotics have been linked to enhanced digestive enzyme activity. In a study reported by Yang et al. (Citation2005) lipase, Isomaltase, and sucrase activities in rats fed with a high dose of synbiotic were observed to be increased. The reason for the enhanced enzyme activity was attributed to the healthy gastro-intestinal microbial ecology or modified bacterial enzyme secretions. Similarly, Hu et al. (Citation2018) reported that amylase activities in the duodenum and ileum of piglets administered by probiotic Bacillus were substantially higher than those of the piglets fed diets supplemented with antibiotics. Thus, the enhanced gene expression of digestion-related genes Amy, Lip, Tryp in the present study might be due to these beneficial bacteria.
Probiotics also help to boost SCFAs synthesis. Fatty acid production is vital for the maintenance of cellular homeostasis. Fatty acids generated by gut microbiota or host are used as a source/reserve of energy (Laplante and Sabatini Citation2009). By promoting the growth of beneficial gut microbiota, dietary mannanase may enhance the intestinal SCFAs content of C. carpio. The relative expression levels of the FABP and FAS genes were found to be higher in the treatment groups which might be linked to enhanced production of SCFAs by beneficial microorganisms. This shows that mannanase may have a role in C. carpio fatty acid production, specifically SCFAs.
In broilers, supplementation with xylanases and to some extent cellulases have shown good results, while in a soybean-based diet, pectinases and mannanases have been effective (Sinha et al. Citation2011). The positive effects of dietary mannanase supplementation on growth performance of broilers (Zangeneh and Torki Citation2011; Cho and Kim Citation2013) swine (Yoon et al. Citation2010) and turkeys (Jackson et al. Citation2008) have been reported in several studies. However, no comparable study exists related to the effect of mannanase supplementation on the growth of fish except one conducted by Chen et al. (Citation2016) who reported that mannanase addition in fish feed is beneficial for the growth of tilapia. It seems that the significant increase in growth performance observed in the present study was due to improved digestion and availability of nutrients, which is supported by our results related to enhanced digestion-related gene expression. However, higher dietary mannanase concentrations, on the other hand, did not provide improved results. Microbial mannanase can be an excellent feed additive in terms of economic feasibility, and its optimal dosage for C. carpio intestinal health regulation under the current study is 500 U/kg.
5. Conclusion
The addition of β-mannanase to a plant-based diet modulated the gut microbiota of C. carpio, while certain beneficial microorganisms were plentiful, opportunistic pathogens were absent. Mannanase also improved growth and upregulated the expression of key digestive and immune-related genes in the intestine. These findings imply that mannanase may help regulate intestinal health in C. carpio and is a candidate as a suitable fish feed additive; the optimal dietary supplementation dosage is a 500 U/kg diet. However, additional research is needed to better understand the mechanism of action of β-mannanase, as well as to investigate its potential as an immunity enhancer and modulator of gut microbiota.
Disclosure statement
No potential conflict of interest was reported by the author(s).
Additional information
Funding
References
- Amir I, Zuberi A, Imran M, Ullah S. 2018. Evaluation of yeast and bacterial based probiotics for early rearing of Labeo rohita (Hamilton, 1822). Aquac Res. 49:3856–3863. doi:10.1111/are.13852.
- Amir I, Zuberi A, Kamran M, Imran M. 2019. Evaluation of commercial application of dietary encapsulated probiotic (Geotrichum candidum QAUGC01): effect on growth and immunological indices of rohu (Labeo rohita, Hamilton 1822) in semi-intensive culture system. Fish Shellf Immunol. 95:464–472. doi:10.1016/j.fsi.2019.11.011.
- Carter GR, Cole JR. 2012. Diagnostic procedure in veterinary bacteriology and mycology. New York: Academic Press.
- Chakrabarti R, Sharma J. 2014. Digestive enzyme profile of carps: a overview. Adv Fish Res. 5:59–75.
- Chen W, Lin S, Li F, Mao S. 2016. Effects of dietary mannanase on growth, metabolism and non- specific immunity of Tilapia (Oreochromis niloticus). Aquac Res. 47:2835–2843. doi:10.1111/are.12733.
- Cho J, Kim I. 2013. Effects of beta-mannanase supplementation in combination with low and high energy dense diets for growing and finishing broilers. Livest Sci. 154:137–143. doi:10.1016/j.livsci.2013.03.004.
- Choct M. 1997. Feed non-starch polysaccharides: chemical structures and nutritional significance. Feed Mill Int. 191(6):13–26.
- Dawood A, Zuberi A, Ma K, Imran M, Ahmed M. 2019. Production, purification and characterization of a thermostable – Mannanase from Aspergillus niger AD-01: potential application in aquaculture. Int J Biosci. 15(3):314–327. doi:10.12692/ijb/15.3.314-327.
- De Schryver P, Sinha AK, Kunwar PS, Baruah K, Verstraete W, Boon N, De Boeck G, Bossier P. 2010. Poly-β-hydroxybutyrate (PHB) increases growth performance and intestinal bacterial range-weighted richness in juvenile European sea bass, Dicentrarchus labrax. Appl Microbiol Biotechnol. 86(5):1535–1541. doi:10.1007/s00253-009-2414-9.
- Duan Y, Zhang Y, Dong H, Wang Y, Zheng X, Zhang J. 2017. Effect of dietary Clostridium butyricum on growth, intestine health status and resistance to ammonia stress in Pacific white shrimp Litopenaeus vannamei. Fish Shellf Immunol. 65:25–33. doi:10.1016/j.fsi.2017.03.048.
- Duncan SH, Louis P, Flint HJ. 2007. Cultivable bacterial diversity from the human colon. Lett Appl Microbiol. 44:343–350. doi:10.1111/j.1472-765X.2007.02129.x.
- El Sayed AF. 1998. Total replacement of fishmeal with animal protein sources in Nile tilapia, Oreochromis niloticus (L.), feeds. Aquac Res. 29(4):275–280. doi:10.1046/j.1365-2109.1998.00199.x.
- FAO. 2020. The state of world fisheries and aquaculture sustainability in action. doi:10.4060/ca9229en.
- Francis G, Makkar HP, Becker K. 2001. Antinutritional factors present in plant-derived alternate fish feed ingredients and their effects in fish. Aquaculture. 199(3-4):197–227. doi:10.1016/S0044-8486(01)00526-9.
- Ghori I, Tabassum M, Ahmad T, Zuberi A, Imran M. 2018. Geotrichum candidum enhanced the Enterococcus faecium impact in improving physiology, and health of Labeo rohita (Hamilton, 1822) by modulating gut microbiome under mimic aquaculture conditions. Turk J Fish Aquat Sci. 18:1255–1267. doi:10.4194/1303-2712-v18_11_02.
- Hall M, Beiko RG. 2018. .16s rRNA gene analysis with QIIME2. Meth Mol Biol. 1849:113–129. doi:10.1007/978-1-4939-8728-3_8.
- Hu S, Cao X, Wu Y, Mei X, Xu H, Wang Y, Zhang X, Gong L, Li W. 2018. Effects of probiotic Bacillus as an alternative of antibiotics on digestive enzymes activity and intestinal integrity of piglets. Front Microbiol. 9:2427. doi:10.3389/fmicb.2018.02427.
- Huang XW, Liu XS, Xu FQ. 2003. The effect of β-mannanase on growth performance of growing pigs. Feed Res. 6:29–31.
- Ibrar M, Zuberi A, Amir I, Imran M, Noor Z. 2017. Effect of probiotic Geotrichum candidum on early rearing of Labeo rohita (Hamilton, 1822). Turk J Fish Aquat Sci. 17(6):1263–1270. doi:10.4194/1303-2712-v17_6_19.
- Ichiishi S, Tanaka K, Nakao K, Izumi K, Mikamo H, Watanabe K. 2010. First isolation of Desulfovibrio from the human vaginal flora. Anaerobe. 16:229–233. doi:10.1016/j.anaerobe.2010.02.002.
- Inayat L, Salim M. 2005. Feed conversion ratio of major carp, Cirrhinus mrigala fingerlings fed on soybean meal, maize and maize gluten. Pak Vet J. 25(1):13–16.
- Jackson M, Fodge D, Hsiao H. 1999. Effects of beta-mannanase in corn-soybean meal diets on laying hen performance. Poult Sci. 78:1737–1741. doi:10.1093/ps/78.12.1737.
- Jackson M, Stephens K, Mathis G. 2008. The effect of B-Mannanase (Hemicell Feed Enzyme) and high levels of distillers dried grains on turkey hen performance. Poult Sci. 87:65–66.
- Jiang TT, Feng L, Liu Y, Jiang WD, Jiang J, Li SH, Tang L, Kuang SY, Zhou XQ. 2014. Effects of exogenous xylanase supplementation in plant protein-enriched diets on growth performance, intestinal enzyme activities and microflora of juvenile Jian carp (Cyprinus carpio var. Jian). Aquac Nutr. 20(6):632–645. doi:10.1111/anu.12125.
- Kalhoro H, Zhou J, Hua Y, Ng WK, Ye L, Zhang J, Shao Q. 2018. Soy protein concentrate as a substitute for fish meal in diets for juvenile Acanthopagrus schlegelii: effects on growth, phosphorus discharge and digestive enzyme activity. Aquac Res. 49(5):1896–1906. doi:10.1111/are.13645.
- Laplante M, Sabatini DM. 2009. An emerging role of mTOR in lipid biosynthesis. Curr Biol. 19(22):1046–1052. doi:10.1016/j.cub.2009.09.058.
- Markowiak P, Śliżewska K. 2017. Effects of probiotics, prebiotics, and synbiotics on human health. Nutrients. 9(9):1021. doi:10.3390/nu9091021.
- McLoughlin RF, Berthon BS, Jensen ME, Baines KJ, Wood LG. 2017. Short-chain fatty acids, prebiotics, synbiotics, and systemic inflammation: a systematic review and meta-analysis. AJCN. 106(3):930–945. doi:10.3945/ajcn.117.156265.
- Meehan CJ, Beiko RG. 2014. A phylogenomic view of ecological specialization in the Lachnospiraceae, a family of digestive tract-associated bacteria. Genome Biol Evol. 6(3):703–713. doi:10.1093/gbe/evu050.
- Mefteh FB, Daoud A, Chenari Bouket A, Alenezi FN, Luptakova L, Belbahri L. 2017. Fungal root microbiome from healthy and brittle leaf diseased date palm trees (Phoenix dactylifera L.) reveals a hidden untapped arsenal of antibacterial and broad spectrum antifungal secondary metabolites. Front Microbiol. 8:307. doi:10.3389/fmicb.2017.00307.
- Moncada PA, Budvytiene I, Ho DY, Deresinski SC, Montoya JG, Banaei N. 2013. Utility of DNA sequencing for direct identification of invasive fungi from fresh and formalin-fixed specimens. Am J Clin Pathol. 140:203–208. doi:10.1309/AJCPNSU2SDZD9WPW.
- Mussini FJ, Coto CA, Goodgame SD, Lu C, Karimi AJ, Lee JH, Waldroup PW. 2011. Effect of ß-mannanase on broiler performance and dry matter output using corn-soybean meal-based diets. Int J Poult Sci. 10:778–781. doi:10.3923/ijps.2011.778.781.
- Nie L, Zhou Q-J, Qiao Y, Chen J. 2017. Interplay between the gut microbiota and immune responses of ayu (Plecoglossus altivelis) during Vibrio anguillarum infection. Fish Shellf Immunol. 68:479–487. doi:10.1016/j.fsi.2017.07.054.
- Odetallah N, Ferket P, Grimes J, McNaughton J. 2002. Effect of mannan-endo-1, 4-beta-mannosidase on the growth performance of turkeys fed diets containing 44 and 48% crude protein soybean meal. Poult Sci. 81:1322–1331. doi:10.1093/ps/81.9.1322.
- Pettey L, Carter S, Senne B, Shriver J. 2002. Effects of beta-mannanase addition to corn-soybean meal diets on growth performance, carcass traits, and nutrient digestibility of weanling and growing-finishing pigs. J Anim Sci. 80:1012–1019. doi:10.2527/2002.8041012x.
- Rajoka MS, Shi J, Mehwish HM, Zhu J, Li Q, Shao D, Huang Q, Yang H. 2017. Interaction between diet composition and gut microbiota and its impact on gastrointestinal tract health. Food Sci Hum Wellness. 6:121–130. doi:10.1016/j.fshw.2017.07.003.
- Ringø E, Olsen R. 1999. The effect of diet on aerobic bacterial flora associated with intestine of Arctic charr (Salvelinus alpinus L). J Appl Microbiol. 86:22–28. doi:10.1046/j.1365-2672.1999.00631.x.
- Rupnik M, Wilcox MH, Gerding DN. 2009. Clostridium difficile infection: new developments in epidemiology and pathogenesis. Nat Rev Microbiol. 7:526. doi:10.1038/nrmicro2164.
- Samaddar A, Kaviraj A, Saha S. 2015. Utilization of fermented animal by-product blend as fishmeal replacer in the diet of Labeo rohita. Aquac Rep. 1:28–36. doi:10.1016/j.aqrep.2015.03.004.
- Saputra F, Shiu YL, Chen YC, Puspitasari AW, Danata RH, Liu CH, Hu SY. 2016. Dietary supplementation with xylanase-expressing B. amyloliquefaciens R8 improves growth performance and enhances immunity against Aeromonas hydrophila in Nile tilapia (Oreochromis niloticus). Fish Shellf Immunol. 58:397–405. doi:10.1016/j.fsi.2016.09.046.
- Sinha AK, Kumar V, Makkar HP, De Boeck G, Becker K. 2011. Non-starch polysaccharides and their role in fish nutrition – a review. Food Chem. 127:1409–1426. doi:10.1016/j.foodchem.2011.02.042.
- Tacon AG, Metian M, Hasan MR. 2009. Feed ingredients and fertilizers for farmed aquatic animals: sources and composition (No. 540). Food and Agriculture Organization of the United Nations (FAO). http://www.fao.org/ … /i1142e.pdf.
- Turchini GM, Trushenski JT, Glencross BD. 2019. Thoughts for the future of aquaculture nutrition: realigning perspectives to reflect contemporary issues related to judicious use of marine resources in aqua feeds. N Am J Aquac. 81(1):13–39. doi:10.1002/naaq.10067.
- Walters W, Hyde ER, Berg-Lyons D, Ackermann G, Humphrey G, Parada A, Gilbert JA, Jansson JK, Caporaso JG, Fuhrman JA, Apprill A. 2016. Improved bacterial 16S rRNA gene (V4 and V4-5) and fungal internal transcribed spacer marker gene primers for microbial community surveys. mSystems. 1(1):e00009–15. doi:10.1128/mSystems.00009-15.
- Wang H, Zhang X, Wang S, Li H, Lu Z, Shi J. 2018. Mannan-oligosaccharide modulates the obesity and gut microbiota in high-fat diet-fed mice. Food Funct. 9:3916–3929. doi:10.1039/c8fo00209f.
- Williams HN, Lymperopoulou DS, Athar R, Chauhan A, Dickerson TL, Chen H, Young S. 2016. Halobacteriovorax, an underestimated predator on bacteria: potential impact relative to viruses on bacterial mortality. ISME J. 10(2):491–499. doi:10.1038/ismej.2015.129.
- Wu G, Bryant M, Voitle R, Roland D. 2005. Effect of dietary energy on performance and egg composition of Bovans White and Dekalb White hens during phase I. Poult Sci. 84:1610–1615. doi:10.1093/ps/84.10.1610.
- Yang L, Liu J, Liu M, Qian M, Zhang M, Hu H. 2011. Identification of fatty acid synthase from the Pacific white shrimp, Litopenaeus vannamei and its specific expression profiles during white spot syndrome virus infection. Fish Shellf Immunol. 30:744–749. doi:10.1016/j.fsi.2010.12.026.
- Yang SC, Chen JY, Shang HF, Cheng TY, Tsou SC, Chen JR. 2005. Effect of synbiotics on intestinal microflora and digestive enzyme activities in rats. World J Gastroenterol. 11(47):7413. doi:10.3748/wjg.v11.i47.7413.
- Yoon SY, Yang YX, Shinde PL, Choi JY, Kim JS, Kim YW, Yun K, Kwon IK. 2010. Effects of mannanase and distillers dried grain with solubles on growth performance, nutrient digestibility, and carcass characteristics of grower-finisher pigs. J Anim Sci. 88:181–191. doi:10.2527/jas.2008-1741.
- Zangeneh S, Torki M. 2011. Effects of mannanase supplementing of olive pulp included diet on performance of laying hens, egg quality characteristics, humoral and cellular immune response and blood parameters. Global Vet. 7:391–398.
- Zhang R, Li XY, Cen XL, Gao QH, Zhang M, Li KY, Wu Q, Mu YL, Tang XH, Zhou JP, Huang ZX. 2021. Enzymatic preparation of manno-oligosaccharides from locust bean gum and palm kernel cake, and investigations into its prebiotic activity. Electr J Biotechnol. 49:64–71. doi:10.1016/j.ejbt.2020.11.001.
- Zheng J, Li H, Zhang X, Jiang M, Luo C, Lu Z, Xu Z, Shi J. 2018. Prebiotic mannan oligosaccharides augment the hypoglycemic effects of metformin in correlation with modulating gut microbiota. J Agric Food Chem. 66:5821–5831. doi:10.1021/acs.jafc.8b00829.
- Zheng X, Duan Y, Dong H, Zhang J. 2017. Effects of dietary Lactobacillus plantarum in different treatments on growth performance and immune gene expression of white shrimp Litopenaeus vannamei under normal condition and stress of acute low salinity. Fish Shellf Immunol. 62:195–201. doi:10.1016/j.fsi.2017.01.015.
- Zheng Y, Yu M, Liu Y, Su Y, Xu T, Yu M, Zhang XH. 2016. Comparison of cultivable bacterial communities associated with Pacific white shrimp (Litopenaeus vannamei) larvae at different health statuses and growth stages. Aquaculture. 451:163–169. doi:10.1016/j.aquaculture.2015.09.020.
- Zhou Y, Yuan X, Liang XF, Fang L, Li J, Guo X, Bai X, He S. 2013. Enhancement of growth and intestinal flora in grass carp: the effect of exogenous cellulase. Aquaculture. 416:1–7. doi:10.1016/j.aquaculture.2013.08.023.