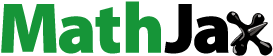
ABSTRACT
The objective of the study was to determine the effects of dietary hempseed or camelina cakes rich in n-3 polyunsaturated fatty acids (PUFA) on the fatty acid profile of liver lipids and carcass characteristics of ducks. A total of 108 one-day-old male ducks were allocated to 3 dietary treatments. The Control (C) group diet was supplemented with 15 and 20% rapeseed cake from days 1 to 23 and 24 to 49, respectively, whereas the rapeseed in Experimental 1 (HE) and Experimental 2 (CA) was replaced by hempseed cake and camelina cake in the same proportion, respectively. Inclusion of dietary hempseed cake resulted in higher carcass yield (P < 0.05) and growth performance (P < 0.05) but did not increase the proportion of liver n-3 PUFA (P > 0.05). Dietary enrichment with camelina cake reduced carcass yield (P < 0.05), feed intake (P < 0.01) but had no significant effect on the body weight and feed conversion ratio (P > 0.05) of ducks. The amount of n-3 PUFA and long-chain (LC) n-3 PUFA in the CA group was 2.47 and 2.35 times (P < 0.01) higher compared with that of the C group, respectively. The inclusion of dietary camelina has helped to produce a healthier human nutrition liver with an n-6/n-3n PUFA ratio of 3.38 .
Introduction
Poultry meat of all meats is mostly preferred by consumers (Association of Poultry Processors Citation2019). Conventionally produced poultry contains small amounts of n-3 polyunsaturated fatty acids (PUFA) (Citation2009; Buckiuniene et al. Citation2016). Research data indicate that poultry can convert dietary α-linolenic (C18:3 n-3; ALA) to long-chain (LC) n-3 PUFA such as eicosapentaenoic (C20:5n-3; EPA) and docosahexaenoic (C22:6n-3; DHA) in a more effective way than cattle, sheep or pigs (Poureslami et al. Citation2010; De Smet and Vossen Citation2016). Feeding strategies have been developed to produce chicken meat and eggs with a higher n-3 PUFA content by using a fish meal or fish oil and linseed or linseed oil (González-Esquerra and Leeson Citation2001; López-Ferrer et al. Citation2001a, Citation2001b; Chen et al. Citation2014; Konieczka et al. Citation2017). However, the addition of the above ingredients reduces oxidative stability of food products and consumer acceptability due to foreign odours (López-Ferrer et al. Citation2001a, Citation2001b; Sirri et al. Citation2003; Rymer et al. Citation2010). Other underexploited oilseed crops rich in n-3 PUFA are sought with the aim to use their by-products in poultry nutrition.
Recently, oilseed crops such as camelina (Camelina sativa (L.) Crantz) and hemp (Cannabis sativa L.) have been rediscovered and reintroduced in agriculture due to their wide-range use in biofuel production, chemistry and food industry (Callaway Citation2004; Nain et al. Citation2015; Della Rocca and Di Salvo Citation2020). Considerable amounts of cakes are left after oil production from camelina and hemp seed.
Camelina cake contains 28.8% ALA and 21.1% linoleic acid (C18: 2n-6; LA), and its n-6/n-3 PUFA ratio is 0.8 (Nain et al. Citation2015). Hempseed cake contains 19.1% ALA and 52.5% LA, and its n-6/n-3 PUFA ratio is 4.08 (Da Porto et al. Citation2012; Juodka et al. Citation2018; Mierlita Citation2018). Camelina and hempseed cakes differ in their PUFA composition, and their effects on poultry could be different too.
Scientific literature indicates that the inclusion of camelina and hempseed in poultry diets resulted in the improved composition of muscle fatty acids (Jing et al. Citation2017; Orczewska-Dudek and Pietras Citation2019; Skřivan et al. Citation2020). However, trials were conducted mostly with chicken, layer-hens and turkeys that dominate in poultry farming. There have been no studies yet to prove the effects of hempseed or camelina cakes on the liver fatty acid composition in ducks.
Duck meat production in the world from 2008 to 2018 increased 16.5%, i.e. from 3.83 to 4.46 million tons, and it accounted for 3.51% of total poultry meat production (FAO Citation2018). Researchers observe that consumption of edible animal by-products decreases worldwide (Toldra et al. Citation2016), though the liver is a highly valuable product. Duck liver has 18.70% protein and 4.6% fat (Wu et al. Citation2012; Scans and Christensen Citation2020); it is rich in vitamins A, B12, folate, pantothenic acid, copper, phosphorus, zinc, selenium and iron (Soriano Citation2010; Kicinska et al. Citation2019). The liver fatty acid profile of ducks fed standard diets is characterized by a low content of n-3 PUFA. Moreover, the n-6/n-3 PUFA ratio is too high (14.77) in human nutrition (Chen et al. Citation2017).
The increase of dietary ALA content leads to the conversion of ALA to EPA and DHA in the bird but the synthesized EPA and DHA more often accumulate in the liver than in other tissues (Ajuyah et al. Citation1993). Thus, our study was based on the assumption that feeding n-3 PUFA rich camelina or hempseed cakes should result in LC n-3 PUFA accumulation in the liver. Therefore, the objective of our study was to investigate the effects of dietary hempseed or camelina cakes on the liver lipid fatty acid profile and carcass yield of ducks.
Materials and methods
Poultry, management and study design
The investigations were conducted according to the EU Directive 2010/63/EU (European Parliament and the Council Citation2010), the law of the Republic of Lithuania for animal welfare and handling, Law No. IX-2271 (Seimas of the Republic of Lithuania Citation2012) and the Requirements for keeping, maintenance and use of animals intended for science and education purposes, approved by the order of the State Food and Veterinary Service, 31/10/2012, No. B1-866 (State Food and Veterinary Service Citation2012).
One hundred and eight Pekin male ducks were obtained from a commercial hatchery as hatched and allotted to three dietary treatment groups with three replicates of 12 ducks per replicate each. All treatment groups were fed the same basic diet composed of wheat–barley–soybean-meal (). Additionally, the Control (C) group diet was supplemented with 15 and 20% rapeseed cake from days 1 to 23 and 24 to 49, respectively, whereas the rapeseed in Experimental 1 (HE) and Experimental 2 (CA) was replaced by hempseed cake and camelina cake in the same proportion, respectively.
Table 1. Composition of the experimental diets.
The stocking rate of ducks was 10 per square metre, and every 1–3-week-old duck had 1.5 cm feeder space and 1 cm watering space. Ducks older than 3 weeks had 2 cm space access to the feeder. The thickness of the litter was 15 cm. Air temperature under the brooder was 35–26°C and 25–22°C, respectively at week 1 and week 2 of rearing and 18–20°C at the last week. The ducks were maintained under 18 h light: 6 h dark photoperiod, except for the first seven days of life (24 h light). The relative humidity in the aviary was 65–75% throughout the entire period. The microclimate parameters were controlled. The air exchange was 7.0 m3/h/kg of live body weight. The air velocity in the building amounted to 0.4 m/s. During the experimental period, water and mash were provided ad libitum.
The feeds were analysed: for dry matter content by oven-drying at 105°C up to constant weight; crude protein was analysed by the Kjeldhal method; crude fibre was analysed by the Fibercap method; calcium was analysed by the method of atomic absorption using caesium chloride and aluminium nitrate; phosphorus was analysed by the photometric method using a molibdovanadate reagent (AOAC Citation1990). Rapeseed, hempseed and camelina cakes were analysed for the fatty acid composition ().
Table 2. Fatty acid composition of rapeseed, hempseed and camelina cakes (% of total fatty acids).
At the age of 49 days, six ducks, from each treatment group, were selected for control slaughtering. The live weight of the ducks for slaughter was equal to the average weight in each group. Prior to slaughter, the birds were fasted for 12 h, but free to water. The ducks were slaughtered in a commercial EU-licensed abattoir.
The carcasses were anatomically dissected according to the methodological recommendation for anatomic carcass dissection and organoleptic evaluation of poultry (Lukashenko et al. Citation1984).
The carcass weight and weight of edible and non-edible internal parts were determined. The carcass yield percentage was calculated as the ratio of the carcass weight with the neck and edible internal parts to the live body weight. The percentage of breast and leg muscles, heart, stomach, liver, abdominal and non-edible parts was calculated as their part in percentage from the carcass weight. At slaughter, the liver was taken, held at 4°C and delivered to the laboratory within 2 h after slaughter for the analysis of the fatty acid composition.
Fatty acid analyses
The extraction of lipids for fatty acid analysis was performed with a mixture of two volumes of chloroform (Chromasolv Plus for high-performance liquid chromatography (HPLC) containing 0.5–1.0% ethanol as a stabilizer) and one volume of methanol (Chromasolv for HPLC ≥ 99.9%) as described by Folch et al.(Citation1957). The sample of 2 g liver was mixed with 40 mL chloroform/methanol mixture and homogenized using disperser T 18 (IKA® – Werke GmbH&Co.KG, Germany). After filtration, 20 mL of 0.74% KCl (Sigma-Aldrich, Reagent Plus ≥ 99.0%) solution was added and allowed to stand for 10–12 h. Then, the bottom layer was collected by syringe into a 15 mL test tube and evaporated under vacuum at 50°C. Methylation of the samples was performed using a sodium methoxide 25 wt% solution in methanol (Sigma-Aldrich). Then, 5 mL sodium methoxide was added to the sample and stirred. After 1 h, 7 mL HCl, 6 mL hexane (Sigma-Aldrich, Chromasolv for HPLC ≥ 97.0%) and 2 mL H2O were added. The top layer was transferred into a new test tube and evaporated. The fatty acid methyl esters (FAMEs) were analysed using a gas–liquid chromatography (GC-2010 Shimadzu) fitted with a flame ionization detector.
Separation of methyl esters of fatty acids was affected on the capillary column Rt 2560 (100 m × 0.25 mm × 0.2 μm; Restek, Bellefonte, PA, U.S.A.) by temperature programming from 140 to 240°C. The column was operated at 140°C for 5 min, and then, the temperature was increased to 240°C at 4°C min−1 and held for 20 min. The temperatures of the injector and detector were held, respectively, at 240 and 260°C. The rate of flow of carrier gas (nitrogen) through the column was 1.06 mL/min−1. The peaks were identified by comparison with the retention times of the standard fatty acids methyl esters 37 Component FAME Mix and trans FAME MIX k 110 (Supelco, U.S.A.). The relative proportion of each fatty acid was expressed as the relative percentage of the sum of the total fatty acids using the GC Solution software for Shimadzu gas chromatograph workstations.
Lipid quality indices analyses
Lipid quality indices, i.e. the atherogenic index (AI) and the thrombogenic index (TI) (Ulbricht and Southgate Citation1991), and hypocholesterolemic/hypercholesterolemic (h/H) (Fernández et al. Citation2007) indices were calculated on the basis of fatty acid analysis data as follows:
Statistical analyses
The statistical evaluation of the results was performed using descriptive statistics. Statistical analysis was performed using the statistical software package Statistica, version 7 (StatSoft Inc. STATISTICA Citation2006). The results are expressed as mean values and standard error (SE). Statistical data were analysed using standard methods of one-way analysis of variance (ANOVA). When ANOVA detected differences between the groups, the significance of differences between specific groups was determined with a Student’s t test. The differences were considered statistically significant at P < 0.05. The probability level of P < 0.05 was considered as statistically significant, whereas the differences of 0.05 < P < 0.099 were considered as a tendency to difference.
Results
Growth performance
The first period of the trial until 23 days of age showed that the addition of hempseed or camelina cakes to the duck diets had no effect on their body weight (). However, within the whole trial period, the addition of hempseed cake had a positive effect on the growth performance of ducks. HE group ducks gained the maximum weight, their feed intake was also highest, and the feed conversion ratio was better than that of C group (P < 0.05).
Table 3. Effect of dietary hempseed or camelina cake on growth performance of ducks.
Meanwhile, the addition of camelina cake had no significant influence on the body weight and feed conversion ratio of the CA group but influenced their feed intake which was lower in comparison with C and HE groups by 720 and 1100 g (P < 0.01), respectively. A tendency towards lower body weight in CA group ducks was also observed. The bodyweight of ducks fed camelina cake was 172 g lower (P = 0.054) than that of the C group.
Anatomical dissection of carcass
The use of different oilseed cakes had no effect on such carcass indicators as breast and leg muscles, heart and abdominal fat content but influenced the carcass yield which was highest for the HE group and by 2.06% (P < 0.05) higher compared with that of the C group ().
Table 4. Effect of dietary hempseed or camelina cake on carcass characteristics.
CA group ducks had the lowest carcass yield which was by 2.14% (P < 0.05) and 4.2% (P < 0.01) lower compared with C and HE groups, respectively.
Supplementation of the diet with hempseed cake resulted in lower weight of liver (−1.31%; −1.67% (P < 0.01)) and internal non-edible parts (−2.01% (P < 0.05); −5.25% (P < 0.01)) in comparison with C and CA groups, respectively.
Meanwhile, CA and C group ducks did not show any differences between liver weights as a percentage of carcass weight. Ducks given camelina cake had higher weight of non-edible parts (+3.24% (P < 0.05); +5.25% (P < 0.01)) and stomach (+0.82% (P = 0.054); +1.2% (P < 0.05)) as a percentage of carcass weight compared with the C and HE groups.
Liver fatty acid profile
In our study, saturated fatty acids (SFA) were prevalent in the liver fatty acid profile (). Neither dietary hempseed nor camelina cake had any effect on the total SFA as there were no significant differences in total SFA between the groups.
Table 5. Effect of dietary hempseed or camelina cake on liver saturated (SFA) and monounsaturated fatty acids (MUFA) profile (% of total fatty acids).
The highest total monounsaturated fatty acids (MUFA) content was found in the liver of C group ducks (). Meanwhile, the content of MUFA was the lowest in the liver of CA group ducks. It was 1.35 (P < 0.01) and 1.26 (P < 0.05) times lower than that of C and HE groups, respectively. Within MUFA, the content of oleic (C18:1n-9), hexadecenoic (C16:1n-9) and elaidic (C18:1n-9 trans) acids decreased, whereas that of eicosenoic (C20:1n-9) and erucic (C22:1n-9) acids increased in comparison with HE and C groups. It should be noted that the liver of the CA group contained 10 times (P < 0.01) higher content of erucic (C22:1n-9) acid in comparison with that of the C group.
Most significant changes in the PUFA profile were found in the liver of CA group ducks (). The liver of CA group ducks contained, respectively, 1.36, 2.47 and 2.35 times (P < 0.01) more total PUFA, total n-3 PUFA and total LC n-3 PUFA in comparison with that of the C group.
Table 6. Effect of dietary hempseed or camelina cake on liver polyunsaturated fatty acids (PUFA) profile (% of total fatty acids).
The content of individual LC n-3 PUFA such as ETE, EPA, docosapentaenoic (C22:5n-3; DPA) and DHA in the liver of CA group ducks was 2.3, 2.43, 1.92 and 2.49 (P < 0.01) times higher than that of C group ducks, respectively.
No significant total n-6 PUFA increase was detected in the liver of CA group ducks. However, the content of some individual n-6 PUFA, such as LA, eicosadienoic (C20:2n-6) and docosadienoic (C22:2n-6), was higher (P < 0.01) for CA group ducks than for the C group.
In our study, PUFA/SFA ratio for CA group ducks was 1.35 (P < 0.01) times higher and the ratios of n-6/n-3 PUFA and linoleic/α- linolenic acids were 2.04 and 2.45 (P < 0.01) times lower, respectively, in comparison with those of C group ducks.
The lowest content of total trans-fatty acids and thrombogenic index (TI) was found in the liver of CA group ducks, and they were 1.37 and 1.26 (P < 0.01) times lower, respectively, in comparison with that of the C group ducks.
No significant differences were found for the total SFA, MUFA and PUFA in the liver of ducks fed the diet with hempseed cake supplementation in comparison with the C group. However, linoleic/α-linolenic and hypercholesterolemic/hypocholesterolemic ratios for HE group ducks were, respectively, 1.35 and 1.24 (P < 0.01) times lower in comparison with those of C group ducks. A downward trend (P = 0.052) for the total trans-fatty acid content in the liver of HE group ducks was observed in comparison with C group ducks.
Discussion
Constantly increasing feed costs stimulate the search for underexploited plants which might become energy and protein sources in poultry feeding and reduce dependency on unsustainable conventional feeds such as cereal grains, corn and soybean. Camelina and hemp cultivation is more sustainable in comparison with rapeseed and soya, as it has a higher resistance to many diseases and pests, lower requirements for fertilizers, water and heat, better adaptation to growing under different climatic conditions (Bacenetti et al. Citation2017; Mohammed et al. Citation2017; Leclere et al. Citation2019).
Camelina and hempseed cakes used in poultry feeding could reduce farming costs and at the same time increase n-3 PUFA content in meat and other tissues. Our feeding trial has been conducted to determine the effects of dietary hempseed or camelina cakes on the liver lipid fatty acid profile and carcass characteristics of ducks. To the best of our knowledge, no similar studies with ducks have been carried out so far.
The addition of hempseed cake to the diet has improved body weight, feed intake, FCR, increased the carcass yield and lowered the weight of liver and internal non-edible parts of ducks. The reason for a higher carcass yield could be ascribed to the tetrahydrocannabinol present in hempseed that stimulates appetite and feed intake (Berry and Mechoulam Citation2002; Barth and Rinaldi-Carmonia Citation2005). Moreover, it could have also been influenced by a higher feed digestibility and nutrient availability due to a negligible content of antinutrients present in hempseed and cake (Matthäus Citation1997; Russo and Reggiani Citation2015; Mattila et al. Citation2018). A positive effect of hempseed on poultry growth performance was also reported by other researchers (Khan et al. Citation2010). However, other studies have shown that supplementation of the diets with hempseed cake (5–15%) or seed (5–20%) had no effect on the carcass yield of chickens (Khan et al. Citation2010; Stastník et al. Citation2019).
In our study, the addition of dietary camelina cake reduced the carcass yield and feed intake but had no effect on the FCR and body weight in comparison with the control group. However, there was a tendency towards the bodyweight decrease. The results from our study are in agreement with the findings of Ciurescu et al. (Citation2007), who indicated that 10% camelina seed in broiler diets reduced the carcass yield. Meanwhile, other studies indicated that supplementation of broiler chicken diets with 5–10% camelina seeds or 4–6% oil, or 10% cake had no negative effect on the carcass characteristics (Pekel et al., Citation2009 ; Pietras and Orczewska-Dudek Citation2013; Ciurescu et al. Citation2016; Orczewska-Dudek and Pietras Citation2019). Aziza et al. (Citation2010, Citation2014), Frame et al. (Citation2007) and Bulbul et al. (Citation2015) have also indicated that camelina meal inclusion in broiler, turkey hen and quail diets had no negative effect on the growth performance. Meanwhile, other authors indicate that camelina cake had a negative effect on the growth performance of poultry. Ryhänen et al. (Citation2007) indicated that the use of camelina expeller (5–10%) in broiler starter diets at days 1–14 reduced feed intake, body weight gain and impaired feed conversion ratio. Pekel et al. (Citation2015) who used 10% camelina meal in feed mixture have also reported lower feed intake and body weight but no differences for the feed conversion between the groups. Other authors have found that increasing levels of dietary camelina meal from 5 to 20% in turkey poultry (Frame et al. Citation2007) and from 3 to 15% in broiler chicken diets worsened the growth performance and reduced the apparent total tract digestibility of dry matter, nitrogen and energy (Acamovic et al. Citation1999; Thacker and Widyaratne Citation2012). Pekel et al. (Citation2015) have reported that camelina meal in broiler diets increased viscosity observed in jejunal digesta and, consequently, reduced utilization of energy and nitrogen.
Different research data could be explained by a wide variation of the content of glucosinolates from 13.6 to 36.6 μmol/g in camelina cake produced from different camelina genotypes in different environments (Matthäus and Zubr Citation2000; Thacker and Widyaratne Citation2012; Russo et al. Citation2014; Woyengo et al. Citation2017). Many authors indicate that glucosinolate content is the main reason for lower poultry growth parameters (Acamovic et al. Citation1999; Ryhänen et al. Citation2007; Thacker and Widyaratne Citation2012; Pekel et al. Citation2015). Toxic glucosinolate metabolites have to be detoxified by liver and kidney which might lead to the increase of their metabolic activity resulting in hyperplasia and hypertrophy (Tripathi and Mishra Citation2007). Our study indicated that there was no increase in the liver weight of ducks fed camelina cake in comparison with the C group and similar age Pekin ducks as indicated by Wild (Citation2019). Aziza et al. (Citation2010) have also found that 2.5–10% of dietary camelina meal had no influence on the liver weight of broiler chickens.
Cakes from oilseed crops used in our study had no influence on the liver SFA profile. Meanwhile, dietary camelina cake reduced the content of MUFA in the liver of ducks, especially because the content of the dominant within MUFA oleic acid (C18: 1n-9) was 1.41 times lower in comparison with the C group. This is in agreement with the findings of other authors who indicated linear decreases in MUFA in the liver and muscles of broiler chickens when the feed contained increasing levels of dietary camelina cake inclusions (Aziza et al. Citation2010; Nain et al. Citation2015). These results could be explained by 2.83 and 1.86 times higher n-3 PUFA content in camelina cake in comparison with rapeseed and hempseed cakes, respectively. Increased dietary n-3 PUFA could have caused an inhibition effect on the Δ9-desaturase enzyme, resulting in lower de novo synthesis of liver MUFA (Villaverde et al. Citation2006; Palmquist Citation2009).
The use of dietary camelina cake resulted in the major changes on liver n-3 PUFA profile as the total n-3 PUFA in the liver of CA group ducks was 2.47 times and LC n-3 PUFA 2.35 times higher in comparison with that of the C group. Moreover, significant changes in the content of individual fatty acids were found. The content of ALA and DHA increased, respectively, by 3.41 and 2.49 times in comparison with that of the C group. The results from the study showed more than twice the higher content of LC n-3 ETE and EPA in the liver of CA group ducks. As LC n-3 PUFA content in camelina cake accounted for less than 1%, the increase of liver LC n-3 PUFA cannot be explained by its amount in the feed. The reason for the change is the bioconversion of ALA into liver LC n-3 PUFA.
A similar effect of camelina meal on liver n-3 PUFA in broiler chicken was found by Aziza et al. (Citation2010, Citation2014) and Nain et al. (Citation2015). However, it should be noted that the accumulation of n-3 PUFA in the liver of broilers with 10% camelina cake was more intensive than that of ducks with 15–20% camelina cake. In our study, liver DHA increased 2.49 times, while Aziza et al. (Citation2010, Citation2014) reported 3.31 and Nain et al. (Citation2015) 3.05 times increase, respectively. The differences could be explained by the peculiarities of chicken and duck species (Palmquist Citation2009). Studies indicate that chicken and duck fatty acid elongase 2 (ELOVL2) and ELOVL5 differ by activity and substrate specificity. However, duck and chicken abilities to convert dietary ALA to DHA are comparable (Gregory and James Citation2014).
The liver of CA ducks contained more erucic (C22:1n-9) fatty acid, but its content remained well within regulatory limits allowed for human nutrition (Ryhänen et al. Citation2007; Nain et al. Citation2015).
Dietary camelina cake for ducks had no significant influence on the total n-6 PUFA increase. Meanwhile, the increase of some individual n-6 PUFA such as LA, eicosadienoic (C20:2n-6) and docosadienoic (C22:2n-6) was significant. Similar results were reported by other authors in the trials with camelina cake for broilers (Aziza et al. Citation2010; Nain et al. Citation2015).
The use of camelina cake also resulted in lower n-6/n-3 PUFA and linoleic/α-linolenic ratios and lower thrombogenic index of the duck liver. The n-6/n-3 PUFA ratio was 3.38 and more than twice lower in comparison with that of the C group. The n-6/n-3 ratio determined in our study with CA group ducks meet the requirements for healthy food, as it is lower than 4 (Wood and Enser Citation1997; Gregory and James Citation2014).
The richest and the most often used n-3 PUFA sources are found in fish oil and flaxseed oil. However, our study showed that camelina cake could also be compared with the above sources as the content of liver LC n-3 PUFA increased up to 6.61%, while Chen et al. (Citation2017) indicated up to 7.07% and 4.27% LC n-3 PUFA increase when feeding 2% fish oil or flaxseed oil, respectively. However, fish oil and flaxseed oil increased the liver DHA content more effectively, up to 6.48% and 4.11%, (Chen et al. Citation2017) respectively, against 3.44% in our study. Moreover, the content of other LC n-3 PUFAs, such as EPA and DPA, in the liver of CA ducks was also comparatively high, while the liver of ducks fed 2% fish oil or 2% flaxseed oil had no DPA and the amount of EPA was from 3.21 to 11.25 times lower (Chen et al. Citation2017).
Our study showed that LC n-3 PUFA accumulation in the liver of the CA group was 5.29 and 7.60 times, respectively, higher in comparison with that in breast and leg muscles of the ducks treated in our previous investigation.
Hempseed cake inclusion in the duck diet did not result in any significant changes in the liver n-3 PUFA profile compared with that in the C group. The amount of total PUFA in hempseed cake was 2.52 and 1.50 times higher than in rapeseed and camelina cakes, respectively, but the percentage of n-6 PUFA was even 4 times higher than that of n-3 PUFA. A higher amount of n-6 PUFA inhibited ALA bioconversion to LC n-3 PUFA (Wood et al. Citation2008). LA, a precursor of n-6 PUFA, competed with ALA for the same elongation–desaturation enzymes necessary for the synthesis of both n-3 and n-6 LC fatty acids (Holman Citation1998; Wood et al. Citation2008). The liver of HE group ducks contained a lower elaidic (C18:1n-9trans) fatty acid and lower linoleic/α-linolenic ratio. A negative effect of hempseed cake was found with a lower hypocholesterolemic /hypercholesterolemic ratio.
The results of our study support the notion that one of in vivo PUFA metabolism-regulating factors is dietary fatty acid composition (Wang et al. Citation2005; Jing et al. Citation2013, Citation2017). Jing et al. (Citation2013) found that the expression of FADS1, FADS2, ELOVL2 and ELOVL5 genes in the liver of broiler chickens was higher when the linoleic/α-linolenic ratio in the diet was lower. Our study showed that the inclusion of dietary camelina cake with the lowest linoleic/α-linolenic ratio of 0.78 resulted in the highest n-3 PUFA accumulation in the liver of ducks. In the meanwhile, the above ratio for the hempseed cake was 3.75 and no n-3 PUFA increase was found in the liver of HE group ducks.
The nutritional advice for LC n-3 PUFA amounts to 250 mg daily (EFSA Citation2009). Consumption of 100 g CA group liver supplied 96.4% LC n-3 while consumption of the same amount of C or HE group liver supplied only 39% of the required amount.
The amounts of EPA + DHA obtained in our study were sufficient to label the liver as ‘high in omega-3 fatty acids’ according to European Commission Nutrition Claims (EU Regulation Citation2010).
The above results might arouse consumer interest, and consequently, higher liver consumption aimed with valuable n-3 LC PUFA, the average daily intake values of which are suboptimal in many countries (De Smet and Vossen Citation2016).
Conclusion
Dietary inclusion of camelina cake (15–20%) reduced the carcass yield and feed intake of ducks. However, significant changes in the composition of lipid fatty acids were found, namely, a significant decrease of liver MUFA and an increase of liver n-3 PUFA. The content of ALA was 3.41 times higher and that of LC n-3 PUFA, such as EPA and DHA, was 2.43 and 2.49 times higher in comparison with that of the control group ducks fed rapeseed cake. The use of camelina cake in the feed resulted in significantly lower n-6/n-3 and linoleic/α-linolenic ratios, total trans-fatty acid amount, thrombogenic index and higher PUFA/SFA ratio.
Camelina cake supplementation of duck diets allows producing liver of higher nutritional value and is more beneficial for human health that can be used for further food production development.
The inclusion of dietary hempseed cake resulted in higher carcass yield and improved the growth performance of ducks compared with dietary rapeseed and camelina cakes. However, hempseed cake has not increased the proportion of liver n-3 PUFA.
Disclosure statement
No potential conflict of interest was reported by the author(s ).
References
- Acamovic T, Gilbert K, Lamb K, Walker KC. 1999. Nutritive value of Camelina sativa meal for poultry. Br Poult Sci. 40:S27–S41.
- Ajuyah AO, Hardin RT, Sim JS. 1993. Studies on canola seed in Turkey grower diet: effects on ώ-3 fatty acid composition of breast meat, breast skin and selected organs. Can J Anim Sci. 73:177–181.
- AOAC. 1990. Official methods of analysis. Washington, DC: AOAC.
- Association of Poultry Processors and Poultry Trade in the EU Countries ASBL. 2019. AVEC annual report. Accessed 15 April 2021. https://www.avec-poultry.eu/wp-content/uploads/2019/10/05494-AVEC-annual-report-2019.pdf.
- Aziza AE, Awadin WF, Quezada N, Cherian G. 2014. Gastrointestinal morphology, fatty acid profile, and production performance of broiler chicken fed camelina meal or fish oil. Eur J Lipid Sci Technol. 116:1727–1733.
- Aziza AE, Quezada N, Cherian G. 2010. Feeding Camelina sativa meal to meat-type chickens: effect on production performance and tissue fatty acid composition. J App Poult Res. 19:157–168.
- Bacenetti J, Restuccia A, Schillaci G, Failla S. 2017. Biodiesel production from unconventional oilseed crops (Linum usitatissimum L. and Camelina sativa L.) in Mediterranean conditions: environmental sustainability assessment. Renew Energy. 112:444–456.
- Barth F, Rinaldi-Carmonia M. 2005. Cannabinoids in appetite and obesity. In: Mechoulam R, editor. Cannabinoids as therapeutics. Basel: Birkhäuserv Verlag; p. 219.
- Berry EM, Mechoulam R. 2002. Tetrahydrocannabinol and endocannabinoids in feeding and appetite. Pharmacol Ther. 95:185–190.
- Buckiuniene V, Gruzauskas R, Kliseviciute V, Raceviciute-Stupeliene A, Svirmickas G, Bliznikas S, Miezeliene A, Alencikiene G, Grashorn MA. 2016. Effect of organic and inorganic iron on iron content, fatty acid profile, content of malondialdehyde, texture and sensory properties of broiler meat. Europ Poult Sci. 80:1–14.
- Bulbul T, Rahman A, Ozdemir V. 2015. Effect of false flax meal on certain growth, serum and meat parameters of Japanese quails. J Anim Plant Sci. 25(5):1245–1250.
- Callaway JC. 2004. Hempseed as a nutritional resource: an overview. Euphytica. 140:65–72.
- Chen W, Zhao R, Yan BX, Zhang JS, Huang YQ, Wang ZX, Guo YM. 2014. Effects of the replacement of corn oil with linseed oil on fatty acid composition and the expression of lipogenic genes in broiler chickens. Czech J Anim Sci. 59:353–364.
- Chen X, Du X, Shen J, Lu L, Wang W. 2017. Effect of various dietary fats on fatty acid profile in duck liver: efficient conversion of short-chain to long-chain omega-3 fatty acids. Exp Biol Med. 242:80–87.
- Ciurescu G, Hebean V, Tamas V, Burcea D. 2007. Use of dietary Camelina (Camelina sativa) seeds during the finishing period: effects on broiler performance and on the organoleptic traits of broiler meat. Anim Sci Biotechnol. 40(1):410–417.
- Ciurescu G, Ropota M, Toncea I, Habeanu M. 2016. Camelina (Camelina sativa L. Crantz variety) oil and seeds as n-3 fatty acids rich products in broiler diets and its effects on performance, meat fatty acid composition, immune tissue weights, and plasma metabolic profile. J Agr Sci Tech 18(2):315–326.
- Da Porto C, Decorti D, Tubaro F. 2012. Fatty acid composition and oxidation stability of hemp (Cannabis sativa L.) seed oil extracted by supercritical carbon dioxide. Ind Crops Prod. 36:401–404.
- Della Rocca G, Di Salvo A. 2020. Hemp in veterinary medicine: from feed to drug. Front Vet Sci. 7:387.
- De Smet S, Vossen E. 2016. Meat: the balance between nutrition and health. A review. Meat Sci. 120:145–156.
- EU Regulation. 2010. No 116/2010 of 9 February 2010 amending regulation No1924/2006 of the European parliament and of the council with regard to the list of nutrition claims. Off J Eur Union L. 37:16–18.
- European Food Safety Authority (EFSA). 2009. Labelling reference intake values for n-3 and n-6 polyunsaturated fatty acids. EFSA Journal. 1176:1–11.
- European Parliament and the Council. 2010. Directive 2010/63/EU. The protection of animals used for scientific purposes. Official Journal of the European Union L 276/78.
- FAO. 2018. Livestock primary, production quantity, duck meat. Accessed 7 April 2021. http://www.fao.org/faostat/en/#data/QL.
- Fernández M, Ordonez JA, Cambero I, Santos C, Pin C, de la Hoz L. 2007. Fatty acid compositions of selected varieties of Spanish ham related to their nutritional implications. Food Chem. 101:107–112.
- Folch J, Lees M, Sloane-Stanley GH. 1957. A simple method for the isolation and purification of total lipids from animal tissues. J Biol Chem. 226(1):497–509.
- Frame DD, Palmer M, Peterso B. 2007. Use of Camelina sativa in the diets of young turkeys. J Appl Poult Res. 16(3):381–386.
- González-Esquerra R, Leeson S. 2001. Alternatives for enrichment of eggs and chicken meat with omega-3 fatty acids. Can J Anim Sci. 81:295–305.
- Gregory MK, James MJ. 2014. Functional characterization of the duck and Turkey fatty acyl elongase enzymes ELOVL5 and ELOVL2. J Nutr. 144:1234–1239.
- Holman RT. 1998. The slow discovery of the importance of ώ3 essential fatty acids in human health. J Nutr. 128(2):427S–433S.
- Jing M, Gakhar N, Gibson RA, House JD. 2013. Dietary and ontogenic regulation of fatty acid desaturase and elongase expression in broiler chickens. Prostaglandins Leukot Essen Fatty Acids. 89:107–113.
- Jing M, Zhao S, House JD. 2017. Performance and tissue fatty acid profile of broiler chickens and laying hens fed hemp oil and HempOmegaTM. Poult Sci. 96:1809–1819.
- Juodka R, Juska R, Juskiene V, Leikus R, Stankeviciene D, Nainiene R. 2018. The effect of feeding with hemp and Camelina cakes on the fatty acid profile of duck muscles. Arch Anim Breed. 61:293–303.
- Khan RU, Durrani FR, Chand N, Anwar H. 2010. Influence of feed supplementation with Cannabis sativa on quality of broiler carcass. Pak Vet J. 30:34–38.
- Kicińska A, Glichowska P, Mamak M. 2019. Micro- and macroelement contents in the liver of farm and wild animals and the health risks involved in liver consumption. Environ Monit Assess. 191:132.
- Konieczka P, Czauderna M, Smulikowska S. 2017. The enrichment of chicken meat with omega-3 fatty acids by dietary fish oil or its mixture with rapeseed or flaxseed – effect of feeding duration: dietary fish oil, flaxseed, and rapeseed and n-3 enriched broiler meat. Anim Feed Sci Technol. 223:42–52.
- Leclere M, Jueffroy M-H, Butier A, Chatain C, Loyce C. 2019. Controlling weeds in Camelina with innovative herbicide-free crop management routes across various environments. Ind Crops Prod. 140:111605.
- López-Ferrer S, Baucells MD, Barroeta AC, Galobart J, Grashorn MA. 2001a. n-3 enrichment of chicken meat. 2. Use of precursors of long-chain polyunsaturated fatty acids: linseed oil. Poult Sci 80:753–761.
- López-Ferrer S, Baucells MD, Barroeta AC, Grashorn MA. 2001b. n-3 enrichment of chicken meat 1. Use of very long-chain fatty acids in chicken diets and their influence on meat quality: fish oil. Poult Sci. 80:741–752.
- Lukashenko VS, Lisenko MA, Stoliar TA. 1984. Methodological recommendation of anatomic carcass dissection and organoleptic evaluation of poultry. VASCHNIL, Moscow (in Russian).
- Matthäus B. 1997. Antinutritive compounds in different oilseeds. Lipid Fett. 99:170–174.
- Matthäus B, Zubr J. 2000. Variability of specific components in Camelina sativa oilseed cakes. Ind Crops Prod. 12:9–18.
- Mattila PH, Pihlava JM, Hellström J, Nurmi M, Eurola M, Mäkinen S, Jalava T, Pihlanto A. 2018. Content of phytochemicals and antinutritional factors in commercial protein-rich plant products. FQS. 2(4):213–219.
- Mierlita D. 2018. Effects of diets containing hemp seeds or hemp cake on fatty acid composition and oxidative stability of sheep milk. S Afr J Anim Sci. 48:504–515.
- Mohammed YA, Chen C, Afshar RK. 2017. Nutrient requirements of Camelina for biodiesel feedstock in Central Montana. Agron J. 109(1):309–316.
- Nain S, Oryschak MA, Betti M, Beltranena E. 2015. Camelina sativa cake for broilers: effects of increasing dietary inclusion from 0 to 24% on tissue fatty acid proportions at 14, 28, and 42 d of age. Poult Sci 94:1247–1258.
- Orczewska-Dudek S, Pietras M. 2019. The effect of dietary Camelina sativa oil or cake in the diets of broiler chickens on growth performance, fatty acid profile, and sensory quality of meat. Animals. 9:734.
- Palmquist DL. 2009. Omega-3 fatty acids in metabolism, health, and nutrition and for modified animal product foods. Prof Anim Sci. 25(3):207–249.
- Pekel AY, Kim JL, Chapple C, Adeola O. 2015. Nutritional characteristics of camelina meal for 3 week-old broiler chickens. Poult Sci. 94:371–378.
- Pekel AY, Patterson PH, Hulet RM, Acar N, Cravener TL, Dowler DB, Hunter JM. 2009. Dietary camelina meal versus flaxseed with and without supplemental copper for broiler chickens: Live performance and processing yield. Poultry Science. 88(11):2392–2398. https://doi.org/10.3382/ps.2009-00051
- Pietras MP, Orczewska-Dudek S. 2013. The effect of dietary camelina sativa oil on quality of broiler chicken meat. Ann Anim Sci. 13:869–882.
- Poureslami R, Raes K, Turchini GM, Huyghebaert G, De Smet S. 2010. Effect of diet, sex and age on fatty acid metabolism in broiler chickens: n-3 and n-6 PUFA. Br J Nutr. 104:189–197.
- Russo R, Galasso I, Reggiani R. 2014. Variability in glucosinolate content among camelina species. Am J Plant Sci. 5:294–298.
- Russo R, Reggiani R. 2015. Evaluation of protein concentration, amino acid profile and antinutritional compounds in hempseed meal from dioecious and monoecious varieties. Am J Plant Sci. 6:14–22.
- Ryhänen EL, Pertilä S, Tupasela T, Valaja J, Eriksson C, Larkka K. 2007. Effect of Camelina sativa expeller cake on performance and meat quality of broilers. J Sci Food Agric. 87:1489–1494.
- Rymer C, Gibbs RA, Givens DI. 2010. Comparison of algal and fish sources on the oxidative stability of poultry meat and its enrichment with omega-3 polyunsaturated fatty acids. Poult Sci. 89:150–159.
- Scans CG, Christensen KD. 2020. Poultry Science. Long Grove, IL: Waveland Press.
- Seimas of the Republic of Lithuania. 2012. The provisions of the Republic of Lithuania (2012-10-03) for animal welfare and handling. Law No. XI-2271, No. 108-2728, State News, No. 122-6126, Vilnius, Lithuania (in Lithuanian).
- Sirri F, Minelli G, Iaffaldano N, Tallarico N, Franchini A. 2003. Oxidative stability and quality traits of n-3 PUFA enriched chicken meat. Ital J Anim Sci. 2(Suppl. 1):450–452.
- Skřivan M, Englmaierová M, Taubner T, Skrivanová E. 2020. Effects of dietary hempseed and flaxseed on growth performance, meat fatty acid compositions, liver tocopherol concentration and bone strength of cockerels. Animals. 10:458.
- Soriano J. 2010. Chemical composition and nutritional content of raw poultry meat. In: Guerrero-Legarreta I., Hui Y.H., editor. Handbook of poultry science and technology, volume 1: primary processing. Hoboken, NJ: John Wiley & Sons Inc; p. 467–489.
- Stastník O, Juzl M, Karasek F, Fernandova D, Mrkvicova E, Pavlata L, Nedomova S, Vyhnanek T, Trojan V, Dolezal P. 2019. The effect of hempseed expellers on selected quality indicators of broiler chicken’s meat. Acta Vet Brno. 88:121–128.
- State Food and Veterinary Service. 2012. Sub-statutory act by the State Food and Veterinary Service of Lithuanian Republic regarding the confirmation of the requirements for keeping, maintenance and use of animals intended for science and education purposes, State News, No. 130-6595, Vilnius, Lithuania (in Lithuanian).
- StatSoft Inc. 2006. STATISTICA (data analysis software system), Version 7.0. Tulsa: StatSoft Inc.
- Thacker P, Widyaratne G. 2012. Effects of expeller pressed camelina meal and/or canola meal on digestibility, performance and fatty acid composition of broiler chickens fed wheat-soybean meal-based diets. Anim Nutr. 66:402–415.
- Toldra F, Mora L, Reig M. 2016. New insights into meat by-product utilization. Meat Sci. 120:54–59.
- Tripathi MK, Mishra AS. 2007. Glucosinolates in animal nutrition: a review. Anim Feed Sci Technol. 132:1–27.
- Ulbricht TLV, Southgate DAT. 1991. Coronary heart disease: seven dietary factors. Lancet. 338:985–992.
- Villaverde C, Baucells MD, Cortinas L, Barroeta AC. 2006. Effects of dietary concentration and degree of polyunsaturation of dietary fat on endogenous synthesis and deposition of fatty acids in chickens. Br Poult Sci. 47:173–179.
- Wang Y, Botolin D, Christian B, Busik J, Xu J, Jump DB. 2005. Tissue-specific, nutritional, and developmental regulation of rat fatty acid elongases. J Lipid Res. 46:706–715.
- Wild C. 2019. Performance of current Peking duck breeds. Lohmann Inform. 53(2):28–32.
- Wood JD, Enser M. 1997. Factors influencing fatty acids in meat and the role of antioxidants in improving meat quality. Br J Nutr. 78:49–60.
- Wood JD, Enser M, Fisher AV, Nute GR, Sheard PR, Richardson RI, Hughes SI, Whittington FM. 2008. Fat deposition, fatty acid composition and meat quality: a review. Meat Sci. 78(4):343–358.
- Woyengo TA, Beltranena E, Zijlstra RT. 2017. Effect of anti-nutritional factors of oilseed co-product on feed intake of pigs and poultry. Anim Feed Sci Technol. 233:76–86.
- Wu L, Guo X, Fang Y. 2012. Effect of diet dilution ratio at early age on growth performance, carcass characteristics and hepatic lipogenesis of pekin ducks. Rev Bras Cienc Avic. 14:43–49.