ABSTRACT
This study aims to evaluate the effects of dietary concentrate: forage ratio (C:F) on the development of the gastrointestinal tract in Tibetan lamb. A total of 60 healthy two-month-old weaned black Tibetan sheep (approximately 10.50 kg of initial body weight) were assigned to three treatments and fed C:F of 70:30 (HC group), 50:50 (MC group) and 30:70 (LC group). Using the haematoxylin-eosin (HE) staining, morphological results showed that the increase of dietary roughage level from 30 to 70% exerts a positive effect on the development of the forestomach including rumen, reticulum and omasum, especially in papillae and muscle layer. Both submucosa thickness and muscle layer of abomasum are significantly increased in the LC group when compared with the HC group. Moreover, the mucosal thickness of the small intestine is higher in the LC group than that in the HC group, whereas crypt depth is higher in HC compared to the LC group. These data suggest that increased dietary forage levels promote gastrointestinal tract growth in black Tibetan sheep by changing in papilla, muscle layer and mucosal thickness, and concentrate can be supplemented up to 30% to fulfil the nutrient requirements without restriction on the development of the gastrointestinal tract.
1. Introduction
Young ruminants are vulnerable to several external environmental and nutritional factors (Lv et al. Citation2019). Previous studies have shown that early supplementary feeding maintains the normal development of the digestive system in fattening stages (Khiaosa-ard and Zebeli Citation2014).
Carbohydrates are mainly categorized into starch and neutral detergent fibre (NDF), the main structural and non-structural carbohydrate components, respectively (Khan et al. Citation2008). As opposed to roughages, concentrate feeds are provided to obtain high dry matter intake (DMI) and subsequent rapid volatile fatty acid (VFA) production, ensuring abundant protein for microbial growth in goats (Ma et al. Citation2021). However, NDF of diet is the pivotal index for the nutritional evaluation, since it promotes the morphological and functional development of the gastrointestinal tract and the organism’s health (Quigley et al. Citation2018). Feedlot lambs receiving 6% forage NDF have a higher average daily gain than those fed 9% forage NDF (Flores-Mar et al. Citation2017). Increasing the NDF diet from 9.9 to 15.8% significantly promotes the stabilization of the rumen environment and the nutrient absorption rate in veal calves (Suarez et al. Citation2006). Additionally, an increase in dietary NDF levels displays promising results in the muscle layer, mucosal area and papilla morphology of the intestinal tract, thereby maintaining intestinal tract development (Sun et al. Citation1994). However, feeding high-concentrate or restricted-roughage rations is found to decrease rumination time, rumen pH and microbial diversity by changing rumen fermentation, resulting in excessive keratinization of nipples and restriction of nutrient absorption (Xie et al. Citation2020).
The Tibetan sheep (Ovis aries) originate from the Tibet Plateau, with excellent adaptability to harsh conditions in high altitude areas, such as extremely cold winters, food shortages and drought seasons (Gui et al. Citation2021). In the process of evolution, the Tibetan sheep develop an efficient digestive system, which effectively converts plant structural carbohydrates into volatile fatty acids, resulting in ruminal microbial fermentation. It plays a pivotal role in the maintenance of the organismal metabolic process (Fan et al. Citation2021). The ongoing climate change has significantly reduced the available grassland, which restricts the traditional grazing management (Cao et al. Citation2021). Based on the economics principle, a strategy to eliminate the contradiction between economic development and ecological protection is essential in animal husbandry.
This study uses black Tibetan sheep living above an average altitude of 3500 m as the research objects to evaluate the effects of different ratios of concentrate to roughage diets (C:F) on their gastrointestinal tract morphology during the fattening period. The results can provide a theoretical basis for the formulation of feeding and management of black Tibetan sheep.
2. Materials and Methods
2.1. Experimental design
A total of 60 healthy two-month-old black Tibetan sheep (initial body weight of 10.45 kg ± 0.96) were selected from the Tibetan sheep breeding centre in Guinan County, Hainan Prefecture, Qinghai Province, China. Animals were randomly allocated into three groups (20 per group) and were fed with different total mixed rations of C:F of 30:70 (LC group), 50:50 (MC group) and 70:30 (HC group). Prior to the study, animals were allowed an eight-day adaptation period to familiarize themselves with the experimental diet, and the feeding experiment lasted for 120 days. Basal diets of sheep were formulated according to the requirement of the NRC (Citation2007). All diet samples were ground to allow passage through a 0.42-mm sieve (Zhejiang Shangyu Machinery, China) and were analyzed for dry matter (method 924.05; AOAC Citation1990), crude protein (method 988.05; AOAC Citation1990), ash (method 942.05; AOAC Citation1990) and ether extract (method 920.39; AOAC Citation1990). Neutral detergent fibre and acid detergent fibre were determined according to methods described by Van Soest et al. (Citation1991). The composition of each dietary treatment is presented in .
Table 1. Ingredient and analyzed composition (dry matter basis) of experimental diets formulated to contain varying C:F fed to black Tibetan sheep.
2.2. Histochemical analysis
At the end of the feeding experiment, five black Tibetan sheep were randomly selected from each group and were slaughtered according to the animal welfare procedures. About 1.0 cm2 samples from the ventral region were collected in rumen, reticulum, omasum and abomasum. Moreover, 8 cm of the small intestine was removed from the middle part of the duodenum, jejunum, and ileum. The chyme within the tissue was washed with normal saline and fixed in 4% paraformaldehyde solution (Ozbek et al. Citation2018).
2.3. Data analysis
The initial experimental data were preliminarily processed using MS EXCEL (2019 Edition), followed by one-way analysis of variance (ANOVA) using the Statistical Package for the Social Sciences (SPSS) v25.0 software. The Duncan’s post hoc comparative test was subsequently performed among groups. Statistical significance was set at P < .05 and P < .01.
3. Results
3.1. Effects of dietary C:F ratio on the morphological development of abdomen in black Tibetan sheep
The results of histochemical staining in the abdomen including rumen, reticulum, omasum and abomasum, are shown in and . For rumen, papillae width in the LC group is significantly higher than those in the HC group (P < .01). Compared to the HC group, the papillae length and muscle layer in the LC group have higher values (P < .05). Although there are no significant differences among groups for papillae length and papillae width of reticulum increased with the increase of diet concentrate ratio (P > .05). However, the muscle layer is lower (P < .05) for sheep fed the HC treatment than those fed the LC treatment. The papillae length (P < .01) and muscle layer (P < .05) in omasum are higher for sheep fed LC treatment than those fed HC treatment. Papillae width is not affected by the dietary C:F ratio (P > .05). Except for mucous membrane thickness, a significant difference between the HC and LC group is observed for submucosa thickness (P < .01) and muscle layer (P < .05) in the abomasum.
Figure 1. Morphological structure of abdomen in black Tibetan sheep (200× in rumen, reticulum and omasum, 400× in abomasum). LC, dietary 30C:70F. MC, dietary 50C:50F. HC, dietary 70C:30F.
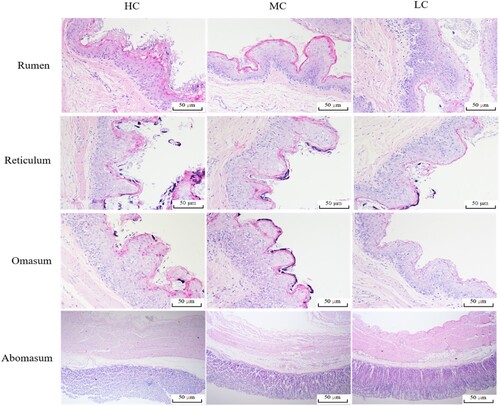
Table 2. Effects of diet C:F ratio on morphology of abdomen in black Tibetan sheep.
3.2. Effects of dietary C:F ratio on the morphological development of intestinal tract in black Tibetan sheep
The images of the intestinal tract are presented in and , respectively. For duodenum, muscle layer, mucosal thickness and villus height/crypt depth (V/C) decrease (P < .01) while crypt depth increases (P < .01) with an increase in dietary concentrate level. However, villus height and villus width do not differ among groups (P > .05). For jejunum, villus width (P < .05), mucosal thickness (P < .05) and V/C (P < .01) are significantly reduced as an effect of the increased dietary concentrate level. Both villus height and muscle layer are similar among the treatment groups (P > .05). For ileum, the villus height (P < .01), mucosal thickness (P < .05) and V/C (P < .01) of the LH group exhibit the highest, whereas the crypt depth of the LH group is significantly lower in the three groups (P < .01). However, no significant difference in muscle layer is observed for all groups (P > .05).
Figure 2. Morphological structure of small intestine in black Tibetan sheep (100×). LC, dietary 30C:70F. MC, dietary 50C:50F. HC, dietary 70C:30F.
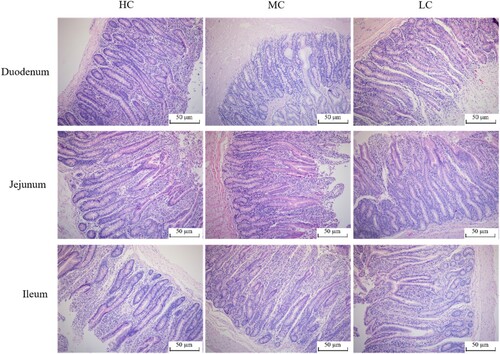
Table 3. Effects of diet C:F ratio on morphology of small intestine in black Tibetan Sheep.
4. Discussion
The rumen is a natural bioreactor for plant fibre degradation, where the structural carbohydrates are converted into a range of volatile fatty acids by rumen microbes (Mao et al. Citation2015). These fatty acids meet approximately 70% energy requirement for the host animal (Bergman Citation1990). Wang et al. (Citation2020) used dairy cows to evaluate the effects of two C:F (70:30 or 30:70) ratios on the microbial community and reported that rumen microbiota diversity and bacterial species richness increased quadratically with the decrease of C:F (Wang et al. Citation2020). Zhou et al. (Citation2018) evaluated the effects of nitrogen concentration (10.32, 19.49, 28.50 or 37.58) based on the different ratios of C:F diets and found that increasing nitrogen concentration increased linearly ruminal concentrations of ammonia, urea and amino acids in beef cattle. As the level of concentrate supplement in the Awassi lamb diet increased, the digestibility of crude protein ether extract and metabolizable energy increased, but the crude fibre, acid detergent fibre and cellulose intake decreased (Hassan and Mohammed Citation2014). Therefore, the optimal ratio of C:F diet and scientific management in ruminants could promote the development of the ruminal function, thus improving the production performance.
Previous studies have shown that concentrate dietary levels after calving affect the rate of increase of papilla surface area, suggesting that papilla morphology is modified by an increased level of fermentable organic matter intake (Dieho et al. Citation2016). Zitnan et al. (Citation1998) observed that the pH, acetate to propionate ratio and length of papillae in rumen were decreased in barley/soybean meal compared to alfalfa fed group in calves during the weaning period. Estevam et al. (Citation2020) reported that Nellore yearling bulls were adapted by 14 days in concentrate supplement through morphological changes and branching of rumen papilla. In this study, our data demonstrated that supplementation of C:F at 3:7 improved papillae and muscle in the ovine abdomen. The main reason was that adequate physical stimulation from dietary roughage induced gastric motility and improved the development of the muscular papillae and muscle.
During the morphological assessment of the intestinal tract in livestock, the villus height, crypt depth and muscle layer thickness of small intestine are important indicators (Sun et al. Citation2018) that reflect the ability of the gastrointestinal tract to digest and absorb nutrients (McCoard et al. Citation2020). The absorbing ability of nutrients is enhanced with the increase of villus height (Short and Derrickson Citation2020). The crypt depth correlates with the rate of multiplication of new cells is the place of regeneration of the intestinal epithelium (Boguslawska-Tryk et al. Citation2020). In addition, the intestinal mucosa sustains intestinal homeostasis by preventing the entrance of biological and chemical agents across the epithelium (Moreto and Perez-Bosque Citation2009). Via improving the morphological structure of the intestinal tract, appropriate strategies are critical for gaining and maintaining nutrition and development in livestock.
O2 consumption is higher in the colon mucosa of forage-fed compared with that of concentrate-fed lambs, suggesting a positive effect of forage diets on the thermogenesis of ruminants (Finegan et al. Citation2001). McLeod and Baldwin (Citation2000) found that jejunal epithelial mass and digestive tract weight increased for lambs fed 75% forage compared with 75% concentrate in sheep. In contrast, Wang et al. (Citation2021) showed that the increase in dietary concentrate feed level from 55% to 90% exerted a positive effect on carcass characteristics, meat quality and development of colonic villus in Liuyang Black goat. In this study, increased concentrate intake increases crypt depth, whereas mucosal thickness and the V/C ratio are higher for lambs fed 70% forage vs. 70% concentrate due to the absence of the sufficient plant structural carbohydrates in the diet. The roughage diet exhibits excellent water-absorption and water-retention abilities, which enhance satiety sense and intestinal peristalsis, thereby promoting the intestinal development (Loor et al. Citation2004).
5. Conclusion
In conclusion, we conclude that the digestive tract tissue morphology of black Tibetan sheep is affected by diets with different concentrate-to-forage ratios. Increased dietary roughage feed level exerts a positive effect on the papilla and muscle layer in the abdomen, and mucous in the small intestine, enhancing feed digestibility and health status associated with artificial rearing of lambs.
Acknowledgement
Taif University Researchers Supporting Project number (TURSP-2020/222), Taif University, Taif, Saudi Arabia.
Disclosure statement
No potential conflict of interest was reported by the author(s).
Correction Statement
This article has been republished with minor changes. These changes do not impact the academic content of the article.
Additional information
Funding
References
- [AOAC] Association of Official Analytical Chemists. 1990. Official method of analysis, Vol. 2. 15th ed. Washington (DC): AOAC.
- Bergman EN. 1990. Energy contributions of volatile fatty-acids from the gastrointestinal-tract in various species. Physiol Rev. 70:567–590.
- Boguslawska-Tryk M, Bogucka J, Dankowiakowska A, Walasik K. 2020. Small intestine morphology and ileal biogenic amines content in broiler chickens fed diets supplemented with lignocellulose. Livestock Sci. 241:104189.
- Cao JJ, Wei C, Adamowski JF, Biswas A, Li YM, Zhu GF, Liu CF, Feng Q. 2021. On China’s Qinghai-Tibetan Plateau, duration of grazing exclosure alters R:S ratio, root morphology and attending root biomass. Soil Tillage Res. 209:104969.
- Dieho K, Bannink A, Geurts IAL, Schonewille JT, Gort G, Dijkstra J. 2016. Morphological adaptation of rumen papillae during the dry period and early lactation as affected by rate of increase of concentrate allowance. J Dairy Sci. 99:2339–2352.
- Estevam DD, Pereira IC, Rigueiro ALN, Perdigao A, da Costa CF, Rizzieri RA, Pereira MCS, Martins CL, Millen DD, Arrigoni MDB. 2020. Feedlot performance and rumen morphometrics of Nellore cattle adapted to high-concentrate diets over periods of 6, 9, 14 and 21 days. Animal. 14:2298–2307.
- Fan QS, Cui XX, Wang ZF, Chang SH, Wanapat M, Yan TH, Hou FJ. 2021. Rumen microbiota of Tibetan Sheep (Ovis aries) adaptation to extremely cold season on the Qinghai-Tibetan Plateau. Front Vet Sci. 8:673822.
- Finegan EJ, Buchanan-Smith JG, Mcbride BW. 2001. The role of gut tissue in the energy metabolism of growing lambs fed forage or concentrate diets. Br J Nutr. 86:257–264.
- Flores-Mar J, Zinn RA, Salinas-Chavira J. 2017. Influence of forage NDF level and source in growing-finishing diets on growth performance of feedlot lambs. Acta Agri Scand A Anim Sci. 67:134–138.
- Gui LS, Raza SHA, Allam F, Zhou L, Hou SZ, Khan I, Kakar IU, Abd El-Aziz AH, Jia JL, Sun YG, Wang ZY. 2021. Altered milk yield and rumen microbial abundance in response to concentrate supplementation during the cold season in Tibetan sheep. Electron J Biotechnol. 53:80–86.
- Hassan SA, Mohammed SF. 2014. Effects of Saccharomyces cerevisiae supplementation on growth rate and nutrient digestibility in Awassi lambs fed diets with different roughage to concentrate ratios. Biotechnol Biochem Res. 2:37–43.
- Khan MA, Lee HJ, Lee WS, Kim HS, Kim SB, Park SB, Baek KS, Ha JK, Choi YJ. 2008. Starch source evaluation in calf starter: II. Ruminal parameters, rumen development, nutrient digestibilities, and nitrogen utilization in Holstein calves. J Dairy Sci. 91:1140–1149.
- Khiaosa-ard R, Zebeli Q. 2014. Cattle’s variation in rumen ecology and metabolism and its contributions to feed efficiency. Livestock Sci. 162:66–75.
- Loor JJ, Ueda K, Ferlay A, Chilliard Y, Doreau M. 2004. Biohydrogenation, duodenal flow, and intestinal digestibility of trans fatty acids and conjugated linoleic acids in response to dietary forage: concentrate ratio and linseed oil in dairy cows. J Dairy Sci. 87:2472–2485.
- Lv XK, Chai JM, Diao QY, Huang WQ, Zhuang YM, Zhang NF. 2019. The signature microbiota drive rumen function shifts in goat kids introduced to solid diet regimes. Microorganisms. 7:516.
- Ma Y, Wang C, Zhang H, Yu LH, Dong L, Gong DQ, Yao JH, Wang HR. 2021. Illumina sequencing and metabolomics analysis reveal thiamine modulation of ruminal microbiota and metabolome characteristics in goats fed a high-concentrate diet. Front Microbiol. 12:653283.
- Mao SY, Zhang ML, Liu JH, Zhu WY. 2015. Characterising the bacterial microbiota across the gastrointestinal tracts of dairy cattle: membership and potential function. Sci Rep. 5:16116.
- McCoard SA, Cristobal-Carballo O, Knol FW, Heiser A, Khan MA, Hennes N, Johnstone P, Lewis S, Stevens DR. 2020. Impact of early weaning on small intestine, metabolic, immune and endocrine system development, growth and body composition in artificially reared lambs. J Anim Sci. 98:1–11.
- McLeod KR, Baldwin RL. 2000. Effects of diet forage: concentrate ratio and metabolizable energy intake on visceral organ growth and in vitro oxidative capacity of gut tissues in sheep. J Anim Sci. 78:760–770.
- Moreto M, Perez-Bosque A. 2009. Dietary plasma proteins, the intestinal immune system, and the barrier functions of the intestinal mucosa. J Anim Sci. 87:E92–E100.
- [NRC] National Research Council. 2007. Nutrient requirements of small ruminants. Washington (DC): The National Academy Press.
- Ozbek M, Ergun E, Beyaz F, Ergun L, Yidirim N, Ozgenc O, Erhan F. 2018. Prenatal development and histochemical characteristics of gastrointestinal mucins in sheep fetuses. Microsc Res Tech. 81:630–648.
- Quigley JD, Hill TM, Dennis TS, Suarez-Mena FX, Schlotterbeck RL. 2018. Effects of feeding milk replacer at 2 rates with pelleted, low-starch or texturized, high-starch starters on calf performance and digestion. J Dairy Sci. 101:5937–5948.
- Short K, Derrickson EM. 2020. Compensatory changes in villus morphology of lactating Mus musculus in response to insufficient dietary protein. J Exp Biol. 223:1–5.
- Suarez BJ, Van Reenen CG, Beldman G, van Delen J, Dijkstra J, Gerrits WJJ. 2006. Effects of supplementing concentrates differing in carbohydrate composition in veal calf diets: I. Animal performance and rumen fermentation characteristics. J Dairy Sci. 89:4365–4375.
- Sun DM, Li HW, Mao SY, Zhu WY, Liu JH. 2018. Effects of different starch source of starter on small intestinal growth and endogenous GLP-2 secretion in preweaned lambs. J Anim Sci. 96:306–317.
- Sun W, Goetsch AL, Forster LA, Galloway DL, Lewis PK. 1994. Forage and splanchnic tissue mass in growing lambs – effects of dietary forage levels and source on splanchnic tissue mass in growing lambs. Br J Nutr. 71:141–151.
- Van Soest PJ, Robertson JB, Lewis BA. 1991. Methods for dietary fiber, neutral detergent fiber, and non-starch polysaccharides in relation to animal nutrition. J Dairy Sci. 74:3583–3597.
- Wang KJ, Peng XM, Lv FF, Zheng ML, Long DL, Mao HX, Si HB, Zhang PH. 2021. Microbiome-metabolites analysis reveals unhealthy alterations in the gut microbiota but improved meat quality with a high-rice diet challenge in a small ruminant model. Animals. 11:2306.
- Wang LJ, Li Y, Zhang YG, Wang LH. 2020. The effects of different concentrate-to-forage ratio diets on rumen bacterial microbiota and the structures of holstein cows during the feeding cycle. Animals. 10:957.
- Xie B, Huang WQ, Zhang CX, Diao QY, Cui K, Chai JM, Wang SQ, Lv XK, Zhang NF. 2020. Influences of starter NDF level on growth performance and rumen development in lambs fed isocaloric and isonitrogenous diets. J Anim Sci. 98:1–8.
- Zhou JW, Liu H, Zhong CL, Degen AA, Yang G, Zhang Y, Qian JL, Wang WW, Hao LZ, Qiu Q, et al. 2018. Apparent digestibility, rumen fermentation, digestive enzymes and urinary purine derivatives in yaks and Qaidam cattle offered forage-concentrate diets differing in nitrogen concentration. Livestock Sci. 208:14–21.
- Zitnan R, Voigt J, Schonhusen U, Wegner J, Kokardova M, Hagemeister H, Levkut M, Kuhla S, Sommer A. 1998. Influence of dietary concentrate to forage ratio on the development of rumen mucosa in calves. Arch Anim Nutr. 51:279–291.