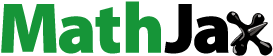
ABSTRACT
Egg production is seasonally regulated by the photoperiod in geese. To evaluate the effect of the natural photoperiod on egg production, 30 males and 120 females of 270-day-old Wanxi white geese and Yangzhou geese were selected and divided into two groups according to the breed. Both groups were raised under the natural photoperiod. Compared with the Yangzhou goose, the Wanxi white goose had a lower laying rate and higher expression levels of follicle-stimulating hormone receptor and anti-Müllerian hormone receptor 2 (AMHR2) in the ovary at the peak-laying period. Compared with the early-laying period, both breeds had lower serum uric acid and triacylglycerol levels, and higher serum cholesterol and high-density lipoprotein cholesterol levels at the peak-laying period. The level of the serum luteinizing hormone (LH) at the pre-laying period was higher than that of the other egg-laying periods in the Yangzhou goose. In conclusion, the photoperiod affected egg production in geese, which was accompanied by changes in serum biochemical parameters and serum reproductive hormone levels. The concentration of the serum LH might be associated with the maturation of ovary and oviducts. In the Wanxi white goose, higher expression of AMHR2 might cause a lower sensitivity of follicles to the level of the FSH.
1. Introduction
Goose is an important economic waterfowl that could provide meat and feather (Shi et al. Citation2008). However, the seasonality of breeding and the low reproductive efficiency hinders the development of the goose industry (Shi et al. Citation2007). Therefore, strategies of improving the reproductive performance is an urgent task in the field of goose research.
The photoperiod is the predominant factor controlling the seasonality of poultry reproductive activity (Shi et al. Citation2007). The photoperiod signals act on photoreceptors located in the retina and the brain (Mobarkey et al. Citation2010), which directly affect the secretion of the gonadotrophin-releasing hormone (GnRH) from the hypothalamus. The GnRH promotes the secretion of gonadotropins (luteinizing hormone, LH; follicle-stimulating hormone, FSH) and prolactin (PRL) from the pituitary gland (Sharp and Blache Citation2003). Furthermore, the FSH promotes gonadal maturation and follicular selection in females, whereas anti-Müllerian hormone (AMH) inhibited follicular maturation through reducing the sensitivity of follicles to the level of the FSH (Ocón-Grove et al. Citation2012). Bone morphogenetic protein 6 (BMP6) promoted follicular maturation through an increase in the expression of follicle-stimulating hormone receptor (FSHR), and thus in gonadotropin-induced progesterone (P4) production (Al-Musawi et al. Citation2007; Ocón-Grove et al. Citation2012). Cholesterol is converted to P4 by the up-regulation of steroidogenic acute regulatory protein (STAR), cytochrome P450 family 11 subfamily A Member 1 (CYP11A1) and 3β-hydroxysteroid dehydrogenase (HSD3B1) (Johnson and Lee Citation2016). These findings indicated that the reproductive activity was controlled by specific hormones which were strongly regulated by the photoperiod.
Geese exhibited seasonal breeding that could be categorized into three types, namely short-day breeding, long-day breeding and partial long-day breeding, depending on the latitude of their habitats (Shi et al. Citation2008; Zhu et al. Citation2010). Geese showed partial long-day breeding usually located at 30°–40° north latitude, the egg production begins in late autumn or early winter, ends in late spring or early summer and generally reaches peak when the day length begins to extend (Shi et al. Citation2008). Both the Yangzhou goose and Wanxi white goose belong to the partial long-day breeding type. It is reported that the annual egg production of the Yangzhou goose is about 80 eggs per year, whereas for the Wanxi white goose it is about 20 to 25 eggs per year (Shi et al. Citation2008; Xia et al. Citation2018).
To overcome the seasonal effect on the egg production, the artificial photoperiod control has been widely used for regulating the egg production in goose. The Yangzhou goose, the first artificially selected four-line breed, showed relatively a high egg production performance through the photoperiod regulation. As an indigenous breed, the Wanxi white goose provides both a high quantity and quality. However, the Wanxi white goose is still reared under natural reproduction systems without any light programme application. Therefore, the photoperiod response might be different in the two breeds. Whether the discrepancy in the egg production performance is caused by different responses to the photoperiod in the two breeds is still unknown. In this study, egg production was recorded in the two breeds under the natural photoperiod regulation. At the same time, serum biochemical parameters, inflammatory factor levels, reproductive hormone concentration and ovary hormone-regulated gene expression levels were recorded to predict the effect of the photoperiod on the egg production.
2. Materials and methods
2.1. Animals and experimental treatments
A flock of 270-day-old Wanxi white geese and Yangzhou geese (n = 150, 1♂: 4♀ per breed) with similar body weight within the breed was raised at the Bengbu Huaxin Poultry Industry Co., Ltd (Bengbu, Anhui, China; east longitude 117.38° and north latitude 32.29°). Geese were assigned into two groups according to the breed, and the two groups were raised separately under the same environmental conditions and natural photoperiod. Both groups were raised in sheds with free access to an outdoor concrete playground with a water pool. All geese were fed ad libitum with mixed feed of 15% crude protein () during the daytime, and had free access to drinking water provided via drinking troughs. The whole experimental period lasted from 1 October 2018 to 6 June 2019. The laying rate was recorded by week.
Table 1. Ingredient and nutrient composition of the experimental diets.
2.2. Sample collection and analytical determination
Blood samples were collected at each egg-laying period (pre-laying, early-laying, peak-laying and falling-laying periods). The blood collection time of the Wanxi white goose was 7th week (12 November 2018), 17th week (21 January 2019), 21th week (18 February 2019) and 29th week (15 April 2019). The blood collection time of the Yangzhou goose was 6th week (5 November 2018), 13th week (24 December 2018), 21th week (18 February 2019) and 31th week (29 April 2019). Ten female geese were randomly selected from each group and about 4 mL of blood was collected from the brachial vein. The blood was collected into anticoagulant-free vacuum tubes. After the blood sample was coagulated, it was centrifuged at 4000 rpm, 4°C for 12 min, and serum samples were collected and preserved under −20°C until the determination of serum reproductive hormone concentration, serum biochemical parameters and serum inflammatory factors level. At the peak-laying period, six female geese were randomly selected from each group for slaughter. Ovaries were collected and immediately frozen in liquid nitrogen and stored under −80°C for RNA extraction.
The concentration levels of the serum PRL, P4, E2, FSH, LH and tumour necrosis factor-α (TNF-α) were measured by Radioimmunoassay (Beijing Northern Biotechnology Institute, Beijing, China). The contents of serum glucose (GLU), uric acid (UA), total protein (TP), albumin (ALB), globulin (GLB), cholesterol (CHOL), triacylglycerol (TG), high-density lipoprotein cholesterol (HDL-C) and low-density lipoprotein cholesterol (LDL-C) were determined using commercial kits (Nanjing Jiancheng Institute of Bioengineering, Nanjing, China) and their corresponding procedures. The concentrations of serum interleukin 1 (IL-1), interleukin 6 (IL-6) and interleukin 10 (IL-10) were measured by a commercial ELISA kits (R&D Systems, Beijing, China).
2.3. Gene expression analysis
Real-time quantitative PCR was performed to quantify genes FSHR, BMP6, AMHR2, STAR, CYP11A1 and HSD3B1 mRNA expression in ovary. Gene 18S rRNA was set as an internal control. Gene-specific primers were designed by using Primer Premier 5.0 and synthesized commercially by Qingke biological co. LTD (Beijing, China). Primer sequences are listed in .
Table 2. Primers for RT-qPCR.
Total RNA was extracted from the ovarian tissue by using Trizol (Yeasen Biotech Co., Ltd, Shanghai, China) according to the instructions of the manufacturer. The RNA quality was evaluated via gel electrophoresis. RNA was reverse transcribed to synthesize the first strand cDNA using HifairⅡ 1st Strand cDNA Synthesis Kit (Yeasen Biotech Co., Ltd, Shanghai, China) following the manufacturer's instructions. PCRs were carried out in a 20 μL reaction volume containing 10 μL 2 × SYBR Green Fast q-PCR Mix with Low Rox (Abclonal Biotechnology Co., Ltd, Wuhan, China) and 0.4 μL relevant primer. The gene relative expression levels were calculated by using the 2−ΔΔCT method (Livak and Schmittgen Citation2001). The gene relative expression levels in ovarian tissues of the Yangzhou goose were defined as 1.0.
2.4. Statistical analysis
All data were analyzed by the SPSS 26.0 software. The egg-laying rate, serum biochemical parameters, serum inflammatory factor levels, serum hormone concentrations and ovary gene expression levels between the two groups were analyzed by T-test. Serum biochemical parameters, serum inflammatory factor levels and serum hormone levels at different egg-laying periods were analyzed by one-way ANOVA. The relationships between the natural photoperiod and the egg-laying rate, the natural photoperiod and the serum reproductive hormone were analyzed by regression analysis. The data were considered having a significant difference at p < 0.05. Results were expressed as mean ± SEM.
3. Results
3.1. Natural photoperiod controlled laying rate of the two breeds
The laying rates of the Wanxi white goose and Yangzhou goose were affected by the photoperiod, showing a seasonal egg production character (). The daylight length on 1 October 2018 (1st week) was 11.86 h, and it continuously decreased to 9.98 h at the 13th week (21 December 2018). The daylight length was then continuously increased to 14.20 h on 3 June 2019 (36th week, ).
Figure 1. The natural photoperiod and the laying rate of the Wanxi white goose and Yangzhou goose at Bengbu County. W, Wanxi white goose; Y, Yangzhou goose. Note: The first week was from 1 October 2018 to 7 October 2018.
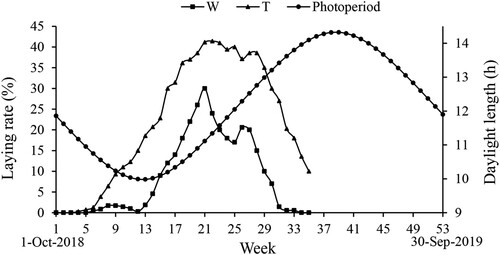
The Yangzhou goose and Wanxi white goose started laying on the 4th week (22 October 2018) and the 6th week (5 November 2018), respectively. The laying rate maintained at a low level of 0.28–1.71% till the 12th week (17 December 2018) in the Wanxi white goose, while increased rapidly and reached to a maximum of 30.00% at the 21st week (18 February 2019), and then dropped to 17.00% at the 25th week (18 March 2019). The laying rate reached to second peak of 20.57% in the 26th week (25 March 2019) in the Wanxi white goose, and gradually decreased to 0.57% at the 32nd week (6 May 2019). In the Yangzhou goose, the egg-laying rate was only 0.29% at the 4th week, and gradually reached to 30.00% at the 16th week (14 January 2019), then maintained above 30.00% from the 16th to the 30th week (22 April 2019) with highest level of 41.43%. The Wanxi white goose exhibited lower egg-laying rate than the Yangzhou goose at both peak- and falling-laying periods (P < 0.05, ). No significant difference was observed during the early-laying period between the two breeds (P > 0.05, ).
Table 3. Laying rates in different egg-laying periods in the Wanxi white goose and Yangzhou goose.
3.2. Egg-laying curves based on the natural photoperiod
The egg-laying rate increased with the shortening of the daytime before the winter solstice in the Yangzhou goose (P < 0.01, (A)). When the daylight length was less than 12.05 h, the laying rate increased and maintained at a peak level (P < 0.01, (B)). When the daylight length was 12.05 h–14.10 h, the laying rate gradually decreased till it ceased (P < 0.01, (B)).
Figure 2 .#Regression analysis between laying rate and photoperiod. A: Regression analysis between laying rate and photoperiod before winter solstice in the Yangzhou goose; B: Regression analysis between laying rate and photoperiod of the Wanxi white goose and Yangzhou goose after winter solstice. W, Wanxi white goose; Y, Yangzhou goose.
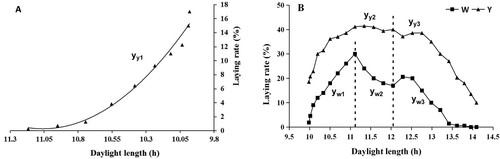
Regression equation between laying rate (y) and photoperiod (x) after the winter solstice in the Wanxi white goose:
(1) | yw1 = −9.1665×2 + 216.15x – 1240.6, 9.983 ≤ x < 11.113, R2 = 0.9855, P < 0.01 yw2 = 16.202×2 – 388.9x + 2350.9, 11.113 ≤ x < 12.048, R2 = 0.9985, p < 0.01 yw3 = −4.0771×2 + 92.553x – 503.13, 12.048 ≤ x < 13.798, R2 = 0.9105, p < 0.01 |
Regression equation between laying rate (y) and photoperiod (x) in the Yangzhou goose ((2): before the winter solstice; (3) after the winter solstice):
(2) | yy1 = 12.896×2 – 285.24x + 1577.6, 9.981 ≤ x < 11.168, R2 = 0.9817, P < 0.01 yy2 = −11.432×2 + 260.27x – 1440, 9.981 ≤ x < 12.048, R2 = 0.959, P < 0.01 | ||||
(3) | yy3 = −8.1675×2 + 198.87x – 1171.1, 12.048 ≤ x < 14.095, R2 = 0.987, P < 0.01 |
The laying rate increased with the extension of the photoperiod and reached peak (30%) when the daylight length was 11.11 h after the winter solstice in the Wanxi white goose (P < 0.01, (B)). When the daylight length was 11.11 h – 12.05 h, the laying rate showed a little decrease (P < 0.01, (B)). When the daylight length was 12.05 h – 13.80 h, the laying rate first increased, then decreased and finally ceased (P < 0.01, (B)).
3.3. Serum biochemical parameters of the two breeds at different laying periods
Compared with the Yangzhou goose, the Wanxi white goose had lower serum GLU and higher serum HDL-C levels at the pre-laying period, higher serum TP and ALB levels at the early-laying period (P < 0.05, ), and it had lower serum GLU, TP, ALB, GLB, CHOL, HDL-C, LDL-C levels and higher serum UA level at the peak-laying period (P < 0.05), while its serum TP, GLB, CHOL, and HDL-C levels were lower at the falling-laying period (P < 0.05).
Table 4. Serum biochemical parameters in different egg-laying periods in the Wanxi white goose and Yangzhou goose.
The serum GLU level at the pre-laying period was lower than that of the other three periods in the Wanxi white goose (P < 0.05), and it was lower than that of the falling-laying period in the Yangzhou goose (P < 0.05). The serum TP, ALB and GLB levels at the early-laying period were higher than that of the other three periods (P < 0.05), and the levels at the pre-laying period were higher than the peak-laying period in the Wanxi white goose (P < 0.05). The serum UA level at the early-laying period was higher than that of the peak-laying period (P < 0.05), and it was lower than the falling-laying period in both breeds (P < 0.05). The serum CHOL, HDL-C and LDL-C concentrations at the falling-laying period were higher than that of pre- and early-laying periods in the two breeds (P < 0.05). The serum HDL-C and LDL-C concentrations in the Wanxi white goose and the CHOL concentration in the Yangzhou goose at the falling-laying period were higher than that of the peak-laying period (P < 0.05). The serum TG level at the pre-laying period was higher than that of peak- and falling-laying periods, and it was lower than the early-laying period in the two breeds (P < 0.05, ).
3.4. Serum inflammatory factors of the two breeds at different laying periods
The serum TNF-α level of the Wanxi white goose was lower than that of the Yangzhou goose at the falling-laying period (P < 0.05, ). The serum IL-10 level of the Wanxi white goose was higher than that of the Yangzhou goose at pre- and peak-laying periods (P < 0.05). The serum IL-6 level at the pre-laying period was higher than that of the falling-laying period in the Yangzhou goose (P < 0.05). The serum IL-10 level at the falling-laying period was lower than that of the other three periods in the Wanxi white goose (P < 0.05).
Table 5. Serum inflammatory factor level in different egg-laying periods in the Wanxi white goose and Yangzhou goose
3.5. Serum reproductive hormone concentrations of the two breeds at different laying periods
The daylight lengths during Wanxi white goose blood collection in the pre-, early-, peak- and falling-laying periods were 10.55 h, 10.34 h, 11.11 h and 12.99 h, respectively ().
Table 6. Serum reproductive hormone concentration in different egg-laying periods in the Wanxi white goose and Yangzhou goose.
The serum PRL concentration in the Wanxi white goose was significantly higher than that of the Yangzhou goose at the early- and peak-laying periods (P < 0.05, ). However, no significant difference was detected at the pre- and falling-laying periods between the two groups (P > 0.05). Serum PRL at the pre- and early-laying periods were significantly higher than that of the peak-laying period in the Yangzhou goose (P < 0.05). Serum PRL at the pre- and early-laying periods were higher than that of the falling-laying period in the Wanxi white goose (P < 0.05).
The serum LH showed no significant difference between the Wanxi white goose and Yangzhou goose at the whole egg-laying period (P > 0.05). The serum LH at the pre-laying period was higher than that of the other three periods in the Yangzhou goose (P < 0.05). The serum LH at early-laying was higher than that of the peak-laying period in the Wanxi white goose (P < 0.05). No significant difference was observed in the FSH and P4 among periods and between breeds (P > 0.05).
The serum E2 concentration and E2/P4 in the Yangzhou goose was significantly higher than that of the Wanxi white goose at the peak- and falling-laying periods (P < 0.05), while no significant difference was observed at the pre- and early-laying periods in the two groups (P > 0.05). Serum E2 level and E2/P4 at the peak-laying period was higher than that of the pre-laying period in the Wanxi white goose (P < 0.05). In the Yangzhou goose, serum E2 concentration and E2/P4 at pre- and early-laying periods was lower than that of the peak- and falling-laying periods (P < 0.05), and E2/P4 at the early-laying period was higher than that of the pre-laying period (P < 0.05).
3.6. Gene expression levels in ovary tissues
Compared with the Yangzhou goose, the Wanxi white goose had a higher expression level of FSHR and AMHR2 in ovary at the peak-laying period (P < 0.05, ). There were no significant differences in the expression levels of BMP6, STAR, CYP11A1 and HSD3B1 between the two breeds (P > 0.05).
Figure 3. Gene relative expression levels of ovary tissues in the Wanxi white goose and Yangzhou goose at peak-laying period. W, Wanxi white goose; Y, Yangzhou goose; Values marked with different letters on the bars were considered significant different (P < 0.05). FSHR, follicle-stimulating hormone receptor; BMP6, bone morphogenetic protein 6; AMHR2, anti-Müllerian hormone receptor 2; STAR, steroidogenic acute regulatory protein; CYP11A1, cytochrome P450 family 11 subfamily A Member 1; HSD3B1, 3β-hydroxysteroid dehydrogenase.
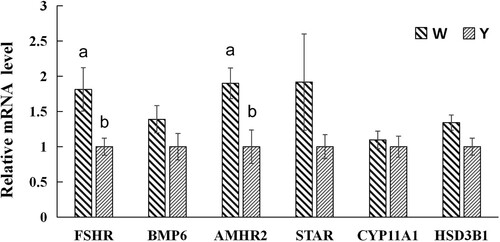
4. Discussion
As partial long-day breeding geese, the reproductive activities begin in late autumn when the day is shortening and ends in late spring or early summer. Egg production generally reaches the peak after the day length begins to extend. However, with the continuous increase of the daylight length, the goose appeared to be photo-passivated and the egg-laying rate began to decrease. In this study, egg laying was monitored in the Wanxi white goose and Yangzhou goose. The Yangzhou goose generally lays eggs from November to June (Yang et al. Citation2017). The Israeli goose lays eggs from October to June (Wang et al. Citation2005). The Magang goose generally lays eggs from July to March (Huang et al. Citation2008). It is reported that seasonal reproduction of geese might be related to latitude (Wang et al. Citation2005), and the seasonality enables the goose to adapt to local environmental conditions, allowed it achieve maximum reproductive performance and survival (Yang et al. Citation2017). It is generally considered that egg production is related to the photoperiod regulation in goose. The different laying rates in the Wanxi white goose and Yangzhou goose could be possibly attributed to the different sensitivity to photoperiod between the two breeds. Therefore, the sensitivity of the Wanxi white goose to the photoperiod can be adjusted through domestication and other methods to increase the egg production. In this study, the regression analysis of the egg-laying rate and photoperiod of the Wanxi white goose and Yangzhou goose was suggested based on previous photoperiod regulation theories (Dawson Citation2015). The regression analysis not only illustrated the biological significances of natural photoperiod that affect egg reproduction cycles of goose, but also can be used to provide mathematical parameters for the future development of artificial light programmes.
Serum biochemical parameters usually reflect the health and metabolism status of poultry, and can be affected by many factors, including genetic factors and serum hormone levels (Manterys et al. Citation2016; Rehman et al. Citation2017). The difference in serum biochemical levels between the Wanxi white goose and Yangzhou goose in this experiment might be caused by genetic factors. Poultry serum biochemical parameters play an important role in maintaining egg composition (Hussein et al. Citation2018). Serum GLU, TP, ALB, GLB, CHOL, HDL-C, LDL-C levels of the Yangzhou goose were higher than that of the Wanxi white goose at the peak-laying period, which was associated with the higher egg-laying rate of the Yangzhou goose during this period.
Glucose is the most important energy source for animals and can be converted into lipids (Hosoda et al. Citation2006; Hussein et al. Citation2018). The level of serum GLU level at the laying period was higher than that of the pre-laying period, which may be regulated by E2 to meet the nutritional needs during laying. The serum ALB, TP and UA levels reflect the protein metabolism of poultry (Pavlik et al. Citation2007; Li et al. Citation2019). The major cholesterol transporters are HDL (α-2-globulin portion) and LDL (β-globulin portion), which carry major serum TP. The serum TP level at the falling-laying period was lower than that at the early-laying period, which might be caused by the increase of serum HDL-C and LDL-C concentrations. Serum UA is the metabolite of protein in poultry. During the peak-laying period, the goose had sufficient energy intake and high egg production. The formation of eggs required the increase of protein synthesis, which led to the decrease of serum TP, ALB, GLB and UA levels. Most egg yolk lipids are composed of phospholipids, CHOL, TG, HDL-C and LDL-C. Under the regulation of reproductive hormones, the lipids such as CHOL, TG, HDL-C and LDL-C in the serum were deposited in egg yolk (Ito et al. Citation2003). There was an association between some serum lipids and egg production. When serum TG level decreased, lipid metabolism slowed down and production performance decreased. During the falling-laying period, due to the decrease in egg production, the fat deposits and the serum CHOL, HDL-C and LDL-C levels increase. It can be stated that under the regulation of the photoperiod, the levels of serum reproductive hormones change, and the geese exhibit seasonal egg laying, accompanied by changes in serum biochemical parameters.
TNF-α, IL-1 and IL-6 are the main pro-inflammatory factors, and IL-10 is an important anti-inflammatory factor in animals. These inflammatory factors play an important role in the process of body injury. IL-1, IL-6 and TNF-α promote the differentiation of lymphocytes through a synergistic effect, thereby causing inflammatory damage to the organisms (Granger and Remick Citation2005). The results of this study showed that the level of serum anti-inflammatory factors in the Yangzhou goose was lower than that of the Wanxi white goose at the pre- and peak-laying periods, and the level of serum pro-inflammatory factors in the Yangzhou goose was higher than that of the Wanxi white goose at the falling-laying period. The stronger inflammatory response of the Yangzhou goose might be related to its higher egg production. The inflammation response of the Yangzhou goose was stronger during the pre-laying period than that of the falling-period, while that of the Wanxi white goose was opposite. The different inflammatory response between the two breeds may be related to their different living habits.
The GnRH in the hypothalamus regulates the secretion of the LH and FSH, which regulates the reproductive seasonality of goose together with PRL and P4 (Sharp and Blache Citation2003). Moreover, PRL plays an important role in maintaining broodiness in goose (Chen et al. Citation2020). The higher PRL and lower E2 might explain the stronger broodiness in the Wanxi white goose and resulted in a lower egg-laying rate. In female, the FSH promotes gonadal maturation and follicular selection and regulates P4 secretion by granulosa cells in the pre-hierarchical follicle. FSH-mediated signaling pathways, including steroid biosynthesis and oxidative phosphorylation, could promote the proliferation of granulosa cells (Du et al. Citation2018), which increased the growth and ovulation of follicle together with P4. There was no difference in the concentration of the FSH and P4 in the laying period between the two breeds, suggested that egg production was not only regulated by the FSH and P4 stimulation.
Studies had shown that long-light photoperiod increased PRL and E2 (Gumulka and Rozenboim Citation2015; Chang et al. Citation2016). Sharp (Citation2002) found that the peak of PRL secretion was highly consistent with the onset of gonadal regression. In order to maintain egg laying, PRL was down-regulated in goose during the peak- and falling-laying stage. Serum PRL was higher in autumn when the day gradually shortened in the Wanxi white goose and Yangzhou goose. PRL was then rapidly decreased with the maturation of gonads under the regulation of the photoperiod. The lowest plasma E2/P4 is detected during the peak-laying period in female quails (Elnaga and Abd-Elhady Citation2009). However, this study had the highest E2/P4 levels during the peak-laying period in both groups. During egg laying, serum E2 rose to a relatively high level (Zhao et al. Citation2017), this might cause a peak E2/P4 observed in this study. It seems that the goose during the pre-laying period could have been stimulated by the short-light photoperiod and began to lay. This might be caused by the elevated serum E2 concentration during the laying period. During the elongated day time, serum E2 is maintained in a high level to maintain an elevated egg-laying rate in the two breeds, which inversely caused decreased serum LH. The high level of the LH at the pre-laying period might suggest it as important hormone for stimulation of ovary and oviduct maturation before egg laying.
After the initiation of primary follicle, granulosa cells began to express FSHR and gradually gained reactivity to FSH. Within 10–15 h after ovulation, E2 was increasingly secreted, while activin and inhibin were transiently secreted in ovary (Davis et al. Citation2001; Lovell et al. Citation2003). Activin and E2 then enhanced the proliferation of granulosa cells and mediated the expression of FSHR and LHR (Davis et al. Citation2001; Lovell et al. Citation2003). Therefore, different expression level of FSHR among individuals within the same period might be a result of different ovulation time. While the higher expression of FSHR in the Wanxi white goose was possibly affected by the time interval between blood collection and ovulation. Johnson et al. (Citation2009) studied the expression of the AMH in two strains of hens differing in ovulatory efficiency, and found that the AMH expression was greater in broiler breeder hens as compared with laying hens. AMH participation in the signaling pathway might be a key factor for inhibition of ovulation in poultry breeds. The increased expression of AMHR2 could reduce the sensitivity of follicle to FSH. The higher expression of AMHR2 might be the main reason that caused the lower egg production in the Wanxi white goose. In vivo, BMP6 induced the expression of AMH and FSHR which regulate the development and growth rate of follicle through paracrine and/or autocrine (Ocón-Grove et al. Citation2012). BMP6 could increase the expression of FSHR (Durlinger et al. Citation2001; Al-Musawi et al. Citation2007). In this study, no difference in the expression of BMP6 suggested that the expression of FSHR was not only regulated by BMP6. STAR, CYP11A1 and HSD3B1 could positively regulate the synthesis of P4 (Johnson and Lee Citation2016). There's no differences in the expression of STAR, CYP11A1 and HSD3B1, which is highly coincident with the serum P4 level between the two breeds.
5. Conclusions
In summary, the photoperiod affected egg production in geese, which was accompanied by changes in serum biochemical parameters and serum reproductive hormone levels. The different egg production between the Wanxi white goose and Yangzhou goose might be because of the different sensitivities to the photoperiod in the two breeds. The Yangzhou goose with a higher laying production has a strong inflammatory response. In the Wanxi white goose, a higher expression of AMHR might cause a lower sensitivity of follicles to the FSH.
Disclosure statement
No potential conflict of interest was reported by the author(s).
Additional information
Funding
References
- Al-Musawi SL, Gladwell RT, Knight PG. 2007. Bone morphogenetic protein-6 enhances gonadotrophin-dependent progesterone and inhibin secretion and expression of mRNA transcripts encoding gonadotrophin receptors and inhibin/activin subunits in chicken granulosa cells. Reproduction. 134:293–306. Epub 2007/07/31.
- Chang SC, Chiang HI, Lin MJ, Jea YS, Chen LR, Fan YK, Lee TT. 2016. Effects of short light regimes and lower dietary protein content on the reproductive performance of White Roman geese in an environment-controlled house. Anim Reprod Sci. 170:141–148. Epub 2016/05/24.
- Chen XC, Zhang YW, Ma WF, Wang ZB. 2020. Effects of Ligustrum lucidum on egg production, egg quality, and caecal microbiota of hens during the late laying period. Ital J Anim Sci. 14(19):687–696.
- Davis AJ, Brooks CF, Johnson PA. 2001. Activin A and gonadotropin regulation of follicle-stimulating hormone and luteinizing hormone receptor messenger RNA in avian granulosa cells. Biol Reprod. 65:1352–1358.
- Dawson A. 2015. Annual gonadal cycles in birds: modeling the effects of photoperiod on seasonal changes in GnRH-1 secretion. Front Neuroendocrin. 37:52–64.
- Du L, Gu T, Zhang Y, Huang ZY, Wu N, Zhao W, Chang G, Xu Q, Chen G. 2018. Transcriptome profiling to identify key mediators of granulosa cell proliferation upon FSH stimulation in the goose (Anser cygnoides). Brit Poultry Sci. 59:416–421.
- Durlinger ALL, Gruijters MJG, Kramer P, Karels B, Kumar TR, Matzuk MM, Rose UM, de Jong FH, Uilenbroek JTJ, Grootegoed JA, et al. 2001. Anti-Mullerian hormone attenuates the effects of FSH on follicle development in the mouse ovary. Endocrinology 142:4891–4899.
- Elnaga SA, Abd-Elhady AM. 2009. Exogenous estradiol: productive and reproductive performance and physiological profile of Japanese quail hens. Int J Poult Sci. 8:634–641.
- Granger J, Remick D. 2005. Acute pancreatitis: models, markers, and mediators. Shock. 24(Suppl 1):45–51. Epub 2005/12/24.
- Gumulka M, Rozenboim I. 2015. Effect of breeding stage and photoperiod on gonadal and serotonergic axes in domestic ganders. Theriogenology. 84:1332–1341.
- Hosoda K, Kuramoto K, Eruden B, Nishida T, Shioya S. 2006. The effects of three herbs as feed supplements on blood metabolites, hormones, antioxidant activity, IgG concentration, and ruminal fermentation in Holstein steers. Asian Austral J Anim. 19:35–41.
- Huang YM, Shi ZD, Liu Z, Liu Y, Li XW. 2008. Endocrine regulations of reproductive seasonality, follicular development and incubation in Magang geese. Animal Reproduction Science. 3(104):344–358.
- Hussein AS, Ayoub MA, Elhwetiy AY, Ghurair JA, Sulaiman M, Habib HM. 2018. Effect of dietary inclusion of sugar syrup on production performance, egg quality and blood biochemical parameters in laying hens. Anim Nutr. 4:59–64. Epub 2018/09/01.
- Ito Y, Kihara M, Nakamura E, Yonezawa S, Yoshizaki N. 2003. Vitellogenin transport and yolk formation in the quail ovary. Zoolog Sci. 20:717–726. Epub 2003/07/02.
- Johnson AL, Lee J. 2016. Granulosa cell responsiveness to follicle stimulating hormone during early growth of hen ovarian follicles. Poult Sci. 95:108–114. Epub 2015/11/18.
- Johnson PA, Kent TR, Urick ME, Trevino LS, Giles JR. 2009. Expression of anti-Mullerian hormone in hens selected for different ovulation rates. Reproduction 137:857–863. Epub 2009/02/20.
- Li F, Shan MX, Gao X, Yang Y, Yang X, Zhang YY, Hu JW, Shan AS, Cheng BJ. 2019. Effects of nutrition restriction of fat- and lean-line broiler breeder hens during the laying period on offspring performance, blood biochemical parameters, and hormone levels. Domest Anim Endocrin. 68:73–82.
- Livak KJ, Schmittgen TD. 2001. Analysis of relative gene expression data using real-time quantitative PCR and the 2(T)(-Delta Delta C) method. Methods 25:402–408.
- Lovell TM, Gladwell RT, Groome NP, Knight PG. 2003. Ovarian follicle development in the laying hen is accompanied by divergent changes in inhibin A, inhibin B, activin A and follistatin production in granulosa and theca layers. J Endocrinol. 177:45–55.
- Manterys A, Franczyk-Zarow M, Czyzynska-Cichon I, Drahun A, Kus E, Szymczyk B, Kostogrys RB. 2016. Haematological parameters, serum lipid profile, liver function and fatty acid profile of broiler chickens fed on diets supplemented with pomegranate seed oil and linseed oil. Brit Poultry Sci. 57:771–779.
- Mobarkey N, Avital N, Heiblum R, Rozenboim I. 2010. The role of retinal and extra-retinal photostimulation in reproductive activity in broiler breeder hens. Domest Anim Endocrin. 38:235–243.
- Ocón-Grove OM, Poole DH, Johnson AL. 2012. Bone morphogenetic protein 6 promotes FSH receptor and anti-Müllerian hormone mRNA expression in granulosa cells from hen prehierarchal follicles. Reproduction 143:825–833. Epub 2012/04/13.
- Pavlik A, Pokludova M, Zapletal D, Jelinek P. 2007. Effects of housing systems on biochemical indicators of blood plasma in laying hens. Acta Vet Brno. 76:339–347.
- Rehman MS, Mahmud A, Mehmood S, Pasha TN, Hussain J, Khan MT. 2017. Blood biochemistry and immune response in aseel chicken under free range, semi-intensive, and confinement rearing systems. Poult Sci. 1(96):226–233. Epub 2016/09/04.
- Sharp PJ. 2002. The role of prolactin in the photoperiodic control of avian gonadotrophin secretion. J Physiol-London. 543:6s–6s.
- Sharp PJ, Blache D. 2003. A neuroendocrine model for prolactin as the key mediator of seasonal breeding in birds under long- and short-day photoperiods. Can J Physiol Pharmacol. 81:350–358. Epub 2003/05/29.
- Shi ZD, Huang YM, Liu Z, Liu Y, Li XW, Proudman JA, Yu RC. 2007. Seasonal and photoperiodic regulation of secretion of hormones associated with reproduction in Magang goose ganders. Domest Anim Endocrin. 32:190–200.
- Shi ZD, Tian YB, Wu W, Wang ZY. 2008. Controlling reproductive seasonality in the geese: a review. World Poultry Sci J. 64:343–355.
- Wang CM, Kao JY, Lee SR, Chen LR. 2005. Effects of artificial supplemental light on the reproductive season of geese kept in open houses. Brit Poultry Sci. 46:728–732.
- Xia M, Wei W, Jiang Z, He D, Li Z, Yu S, Wang Q, Liu H, Chen J. 2018. A functional mutation in KIAA1462 promoter decreases glucocorticoid receptor affinity and affects Egg-laying performance in Yangzhou geese. Int J Mol Sci. 21(19):1531. Epub 2018/06/09.
- Yang HM, Wang Y, Wang ZY, Wang XX. 2017. Seasonal and photoperiodic regulation of reproductive hormones and related genes in Yangzhou geese. Poultry Science. 96:486–490.
- Zhao XZ, Gao GL, Wang HW, Li Q, Zhang KS, Zhong H, Wang QG. 2017. Effect of photoperiod on serum hormone concentrations during the annual reproductive cycle in geese. Genet Mol Res. 22:16. Epub 2017/03/25.
- Zhu WQ, Chen KW, Li HF, Song WT, Xu WJ, Shu JT, Han W. 2010. Two maternal origins of the Chinese domestic grey goose. J Anim Vet Adv. 9:2674–2678.