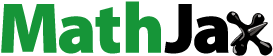
ABSTRACT
A study was conducted in Adola Reedde to identify major grass species and evaluate their chemical composition, in vitro digestibility, and dry matter yield. Sixty key informants taken from the sampled kebele of three agro-climates were interviewed to identify common grasses in their vernacular name. Relative feed value and dry matter digestibility were computed using neutral detergent fibre and acid detergent fibre contents. Spearman's rank correlation was used to examine relationships between laboratory results and farmers' perception of grass quality. Fifteen grass species were identified and ranked by farmers according to the species' preferences for cattle. Crude protein values ranged from 56.5 g/kg DM for lowland to 113 g/kg DM for highland agro-climate. overall mean of neutral detergent fibre was 662 g/kg DM, and in vitro dry matter digestibility and relative feed value were 446.5 g/kg DM and 60.97%, respectively. Total dry matter yield and dry matter of individual grass species were significantly (P < 0.001) higher in highland than in low land agro-climate. Dry matter yield across agro-climate ranged from 92.47 ± 0.04 g m−2-119.41 ± 0.07 g m−2 for highland and lowland agro-climate, respectively. Generally, study highlights the potential of herbaceous species to support livestock production if the grassland is properly rehabilitated and managed.
Introduction
In Sub-Saharan Africa, ruminant livestock commonly relies on feed resources viz: natural grasses, forbs with some browse shrubs and trees (Abusuwar and Ahmed Citation2010). In Ethiopia, the livestock population is 60.39 million cattle, 31.30 million sheep, 32.74 million goats, 56.06 million poultry, 2.01 million horses, 8.85 million donkeys, 0.46 million mules, 1.42 million camels and 5.92 million hive-bee colonies (CSA Citation2017/Citation18). The total production capacity for the country is about 3.32 billion litres of cow milk, 327.64 million litres of camel milk, 66.22 million kilograms of honey and 136.76 million eggs (CSA Citation2017/Citation18).
Even though Ethiopia has an enormous and substantial number of farm animals, their productivity and contribution to its economy are pretty low (Adugna Citation2008). This disparity arises from limited feed resources and the low quality of the existing range of land resources which hinders anticipated livestock productivity (Solomon and Teferi Citation2010). The significant reason for low milk production in Ethiopia is linked to the insufficiency of livestock feeds, especially during the dry season (Zewdie Citation2010). The low nutritive value leads to high livestock death, longer calving interval and substantial weight losses, particularly during the dry season (December to May) in most central Ethiopia (Adugna Citation2007; Shenkute et al. Citation2012). Furthermore, the degradation of rangelands due to the disproportionate use of communal grazing lands of undulated topography in the highlands and erratic rainfall in semi-arid areas have further declined the availability of rangeland resources (Abule Citation2003; Solomon and Teferi Citation2010).
The shortage of animal feed in quantity and quality is worse in arid, semi-arid and tropical regions because of scarce and erratic rainfall that hinder the growth of natural pasture species, resulting in low biomass yield. Thus, livestock in such regions has the challenge of low-quality feed resources for most parts of the year (Robles et al. Citation2008; Boufennara et al. Citation2012).
The productivity of range animals is directly related to the quantity and nutritive quality of the available forage (Hussain and Durrani Citation2009). Sheep is the most appropriate species of livestock for utilization of open sparse vegetation and can convert the course roughages into valuable products meant for human use.
Adugna et al. (Citation2012) classified feed resources into the natural pasture, crop residues, improved pasture and forage and agro-industrial by-products, of which pasture and crop residues contribute the most significant part. Natural pastures encompass naturally occurring grasses, legumes, herbs, shrubs and tree foliage (Adugna Citation2008). The productivity of natural pastures is gradually decreasing because of the rapid increase in human population pressure, expansion of arable land farming for food crops and shrinkage of grazing land areas, resulting in a shortage of livestock feed (Adugna Citation2007). Continuous overstocking also declines the potential of valuable species and favours replacement by less nutritious and unpalatable species (Hassen Citation2006). Even though indigenous plant species are dominant and still contribute as an indispensable source of feed for ruminant livestock across the agro-climatic regions in Ethiopia, the anticipated production potential and quality of natural grazing lands have been decreasing over time and could not support optimal livestock production. This arises from overgrazing of the natural grazing lands due to poor grazing land management (Alemayehu Citation2003; Getnet Citation2003; Wondatir and Yoseph Citation2014). Several studies have evaluated the chemical composition of native grasslands and reported that the potential and nutritional contents of indigenous plant species differin all seasons agro-climates (Angassa and Oba Citation2010; Geleti et al. Citation2012; Keba et al. Citation2013). Natural grazing lands are heterogeneous (Eaton et al. Citation2011). Its chemical constituents differ with environmental factors such as altitude, rainfall, soil type, cropping intensity, grazing land management and variation in the genetic characteristics inherent to specific individual plant species (Alemayehu Citation2003; Robles et al. Citation2008; Teka et al. Citation2012). These factors also affect forage yield, intake and digestibility and animal grazing behaviour (Solomon and Teferi Citation2010).
The feeding value of animal feed determines the optimal herbivore body size and the relative success of livestock. Hence, evaluating the nutritional value of animal feed is imperative in livestock feeding because effective livestock production is linked tothe quantity of nutrients in the feed (Schut et al. Citation2010). The study area, particularly the Adola Reedde district, has huge potential and great diversity of local grass species. However, detailed studies have not yet been done concerning the assessment, identification and determination of the nutritional composition of local grass species. Therefore, the current study was designed with the objectives of identifying major grass species evaluating their chemical composition, in vitro digestibility, and dry matter yield under varying agro-climate of the Adola Reedde district.
Materials and methods
Description of the study area
The study was conducted in the Adola Reedde district, Guji zone of Oromia National Regional State, located about 475 km south of Addis Ababa. The area is located between 5° 44'10″ N–6o12’ 38″ N latitude and 38o 45'10″ E longitude – 39°12’ 37″ E. The district shares boundaries with the Girja district in the Northeast direction, Anna Sorra in the Northwest direction, Oddo Skakiso in the Southern direction and Wadara in the Southeast direction. It has a total area of about 1401km2. The district has 28 rural and two urban kebele, and it is characterized by dega (highland), woina dega (midland) and kola (lowland). The percentage of the coverage of each agro-climate zone of the district is highland at 33%, midland at 47% and lowland at 20%. The major soil of the Adola Reedde district is nitisols (red basaltic soil), and the soil is dominantly brown (Guji Zone Citation2014). Moreover, it has a land surface with an elevation ranging from 1 500 m in the Southern part to over 2 000 m in the Northwestern part. The farming system in the Adola Reedde is traditional, with oxen and yoke, and labour as major means of production during land preparation, planting and harvesting, as well as post-harvest processes. However, semi-pastoral economic activity is also practised as a means of livelihood by some residents (Aschalew Citation2014). Rainfall and temperature of the district are estimated to be 900–1700mm on average and 12oC–28°C. The geographical location of the study area is presented in .
Selection of the study site and sampling methods
The study district was selected from the Guji zone because of its huge potential and great diversity of local grass species, large total area with different agro-ecologies and massive livestock (Guji Zone Citation2014). A multi-stage sampling technique was used to select the agro-climate. The rural kebele (the smallest administrative structure in Ethiopia) was stratified into agro-climates, namely highland (dega), midland (woina dega) and lowland (kola) and the kebele within strata were selected purposively based on the availability, potential and accessibility of indigenous grass species. Accordingly, highland (Meleka and Sekaro), mid-altitude (Gunacho and Bilu), and lowland (Bechera and Chemibe) kebele were selected as representatives of the agro-climates.
Twenty key informants were selected from each agro-climate, giving 60 key informants across the three agro-climates. Common grasses were identified in their vernacular name, and ranking was then done based on information obtained from key informants regarding their relative abundance in the area and their consumption preference by livestock. For fresh herbage biomass yield estimation, three 50-m-long transects were established at three slopes of each kebele, and the average of its yield was used to represent each agro-climate. Then a metal frame quadrant of 0.5 m×0.5 m with short legs welded to its corners was placed at every 10 m starting from one end, making five samples per transect; thus, fifteen 0.25 m2 quadrant samples per each kebele and thirty 0.25 m2 quadrant samples per each agro-climate. The transect positions within each kebele were then selected to represent the upper, medium and lower positions to determine the dry matter yield of different slopes across three agro-climates in the Adola Reedde district. Herbaceous vegetation within the quadrants was manually clipped at the height of 5 cm above the ground (Allen et al. Citation2011), sorted into different species, and their fresh weight was recorded using a sensitive balance. Then, each fresh grass species was chopped into 2–5 cm and dried in an oven at 65 °C for 48 h. Finally, the dry matter (DM) yield of each grass species at each agro-climate was calculated by multiplying the percentage dry weight of each grass species by the fresh weight of the respective grass species in each quadrant per 0.25 m2 area. Herbaceous species were sampled in the mid- September for three consecutive days.
A sampling of local grass species for identification
For grasses whose scientific names were not correctly identified in the field, specimens were collected in duplicate, pressed between white plain papers, labelled, dried and transported to the National Herbarium of Addis Ababa University for identification and naming. These grass species were identified following the guideline provided in the Flora of Ethiopia (Hedberg and Edwards Citation1989, Citation1995).
Preparation of grasses for chemical analysis
The samples of common grass species were bulked together and thoroughly mixed, and then one composite sample was maintained per agro-climate. The air-dried samples were dried at 65 °C for 72 h in an oven and ground in a ‘Willey mill’, which was manufactured under the name Thomas Scientific and registered as the trademark of Arthur H.Thomas Company’ to pass through a 1 mm sieve. Following partial drying in an oven, all grass samples from each agro-climate and 45(15 × 3) from the three agro-climate were subjected to chemical analysis after equilibration to room temperature for 24 h.
Chemical analysis
Proximate analyses and detergent fibre analysis procedures were followed to determine the chemical composition of the grass samples, as outlined below. As the grass was harvested, a wet two-step drying procedure was employed. The grass samples were first dried at 65 °C for 72 h in a forced-draft oven to obtain partial dry matter (Sanson and Kercher Citation1996). The partially dried samples were ground in, and then about 2 g of grass sample was weighed in a clean and dried porcelain crucible and dried in an oven at 105 °C for 24 h. Dry matter (DM%) was calculated using the following formula: Dry matter (DM%) = []×100. Ash was determined according to the procedure of AOAC (Citation1990). Briefly, two grams of ground grass sample was added to a clean, dry crucible in an oven at 100 °C for 2 h and ignited in a muffle furnace at 550 °C for 3 h and weighed after cooling in a desiccator.
Nitrogen content was analyzed according to the procedures described by AOAC (1990). Nitrogen was analyzed by the Kjeldahl technique, and crude protein (CP) content was calculated as nitrogen (N) × 6.25. Neutral detergent fibre (NDF), acid detergent fibre (ADF), and acid detergent lignin (ADL) were determined following the method of Van Soest et al. (Citation1991).
In vitro dry matter digestibility
In vitro dry matter digestibility (IVDMD) of each grass species was determined by the method of Tilley and Terry (Citation1963), as modified by Van Soest and Robertson (Citation1985). A sample analysis was done at the Holeta Agricultural Research Centre animal nutrition laboratory. The samples, dried at 650C to constant weight, were ground to pass through a 1 mm sieve. About 0.5 g of the sample was incubated in 125 ml Erlenmeyer flasks containing rumen fluid-medium mixture. Then, it was incubated for 48 h at 39 °C for microbial digestion. For enzyme digestion, duplicate samples were then incubated for 48 h with acid pepsin solution. Empty samples containing only buffered rumen fluid were also incubated in duplicates for adjustment. The sample residues were dried at 105 °C for 24 h. The rumen fluid was obtained from three rumen fistulated (Boran × Friesian) cross-bred steers kept on a maintenance diet. Dry matter loss was calculated as the difference between the dry matter weight of the sample at the start of the incubation and the weight of residue dry matter remaining at the end of the incubation period, as stated by Tilley and Terry (Citation1963). All measured concentrations were expressed on a dry matter basis. In vitro dry matter (IVDM) was calculated as dry sample weight−(residue- blank)/dry sample weight × 100.
The sample was ashed to estimate in vitro organic matter digestibility (IVOMD). The ME content was estimated using the equation: ME (MJ kg−1 DM) = 0.15*IVOMD (Beever and Mould Citation2000).
Determination of relative feed value
The relative feed value (RFV) was calculated according to Stalling (Citation2005) using the following procedure:
where 1.29 = the expected digestible dry matter intake as % of body weight;
DMD = 83.58−0.824 x ADF% + 2.626 N% (Oddy et al. Citation1983);
DMI = (Sanson and Kercher Citation1996).
Perception analysis
Perceptions of key informants were taken during the field sample collection. A community-level group discussion was held at each agro-climate, and the key informants were selected based on the individual’s experience in livestock keeping and vegetation. The selected respondents participated in the ranking of the same common grass species used for laboratory analysis. The cumulative match of key informant perception of ordering a particular grass species across the study location was divided by the total number of respondents (i.e. 60) and multiplied by 100 to obtain the percentage value for that particular species. All the species were ranked in a descending order based on their percentage value concerning other species under examination. The perception value of individual species was then correlated with the results of the chemical composition of that particular species using Spearman’s rank correlation (Fowler and Cohen Citation1996).
Statistical analysis
Agro-climate and species were considered independent variables. Grass species’ nutritive values and their dry matter yield were considered response variables. General Linear Model procedure of statistical analysis system (SAS) version 9.1 (SAS Citation2008) was used to compute statistical analysis. The experimental design was completely randomized and analyzed as a two-factor experiment (agro-ecology and species). LSD was used to determine mean differences at P≤0.05.
The model used was
where Y is the response variable,
μ is the overall mean,
Ai is the grass species effect,
Bj is the agro-climate effect,
(AB)ij is the interaction between species and agro-climate and
eijk is the random residual error assumed to be normally and independently distributed.
Results
Major grass species
The farmers identified the grass species according to their vernacular name. Fifteen major grass species were found in all agro-climates (lowland, midland and highland). The grass species and their local and botanical names are presented in .
Table 1. The major available grass species identified in the study area.
Chemical composition and IVDMD of grass species
The mean crude protein (CP) values of 15 grass species for the highland and midland agro-climate of the study area were 78 g/kg DM and 73 g/kg DM, respectively, but reduced to a mean value of 66 g/kg DM at lowland agro-climate with an overall mean value of 72.3 g/kg DM. The highest crude protein (CP) was recorded for C. dactylon (L.) Pers (113.0 g/kg DM) at highland agro-climate and the lowest was recorded in H. contortus (L.) Roem. & Schult (56.5 g/kg DM) at lowland agro-climate. The highest dry matter (DM) was recorded for the H. contortus (L.) Roem. & Schult (95.7%) at lowland agro-climate and the lowest was recorded in C. dactylon (L.) Pers (88.7%) at highland agro-climate. All the species recorded higher values for DM, ADF, NDF, ADL, and ash at lowland than in highland and midland agro-climate (). The mean value ash content was significantly higher in the lowland agro-climate than in highland and midland agro-climates and ranged from (132-91.5 g/kg DM), (113.5-87 g/kg DM), and (120.5−92 g/kg DM), respectively. The highest ash content was observed in the H. contortus (L.) Roem. & Schult (132 g/kg DM) followed by C. aucheri (131 g/kg DM) in the lowland, whereas the lowest ash content (87 g/kg DM) was observed in C. dactylon (L.)Pers followed by D. ternata (A. Rich.) Stapf (99 g/kg DM) in highland agro-ecology.
Table 2. Chemical composition of grass species across three agro-climate of the study area.
The highest neutral detergent fibre (NDF) (701.4 g/kg DM), acid detergent fibre (ADF) (492.5 g/kg DM) and acid detergent lignin (ADL) (142 g/kg DM) content were recorded for H. contortus (L.) Roem. & Schult (L.) Roem. & Schul t at lowland Agro-climate and the corresponding lowest NDF (586 g/kg DM), ADF (397.5 g/kg DM), and ADL (90.5 g/kg DM) content were recorded for C. dactylon (L.) Pers in highland agro-climate. The overall mean values of in vitro dry matter digestibility (IVDMD) and metabolizable energy (ME) were 446.5 g/kg DM and 66.9 (MJ), respectively. The highest in vitro dry matter digestibility (IVDMD) (556 g/kg DM) and ME (8.34MJ kg−1 DM) values were observed in the highland agro-climate for C. dactylon (L.)Pers, whereas H. contortus (L.) Roem. & Schult exhibited the lowest in vitro dry matter digestibility (IVDMD) (403 g/kg DM) and metabolizable energy (ME) (6.05MJ kg−1 DM) in lowland agro-climate. Of grass species, the highest relative feed value (RFV) was recorded in C. dactylon (L.)Pers (73.16%) in highland agro-climate, whereas H. contortus (L.) Roem. & Schult (53.88%), and C. aucheri (59.72%) exhibited the lowest relative feed value (RFV) in lowland agro-climate.
Frequency of grass species occurrence
Based on the relative frequencies of each grass species across all agro-climates, three grass species, E. papposa, C. ciliaris, and C. aucheri, with respective percentage values of 62.98, 58.85 and 54.64 were dominant and had high frequencies of >50%. Eleven other species were of intermediate frequency (20−25%), while the remaining two grass species, S. arundinaceum (Desv.) Stapf and S. barbata (Lam.) Kunth, had frequencies below 20%.
Dry matter yield of grass species
Dry weights of individual species in the three agro-climates are presented in . In the highland agro-climate, C. ciliaris, H. contortus (L.) Roem. & Schult, H. hirta (L.) Stapf and C. aucheri were the dominant grass species recorded in higher proportions than others (). In all agro-climates, species such as B. insculpta, C. aucheri, C. dactylon (L.)Pers.), E. papposa, T. triandra, C. ciliaris, H. contortus (L.) Roem. & Schult, and H. hirta (L.)Stapf were commonly recorded. The highes dry matter (DM) yield (119.41 ± 0.07 g m−2) was recorded for highland agro-climate and the lowest dry matter (DM) yield (92.47 ± 0.04 g m−2) was recorded for lowland agro-climate. The effect of intra-location transect position was not significantly different (P > 0.05) for dry matter (DM) yield ().
Table 3. Dry matter yield of herbaceous species by their dry weight across three agro-climates of the Adola Reedde district.
Table 4. Dry matter yield (g m−2) (Mean ± SE) as sampled from different slopes across three agro-climates in the Adola Reedde district.
The rank of grass species according to the perception of key informants
The ranking of the common grass species according to the perception of key informants is presented in . The key informants of the three agro-climates identified common grass species and ranked them according to relative abundance, preferences, and palatability of grass species by grazing animals. Of the 60 key informants, more than 85% mentioned C. ciliaris, C. dactylon (L.)Pers.), S. pyramidalis P.Beauv, H. contortus (L.) Roem. & Schult and C. aucheri were the dominant grasses out of the 15 identified grass species. According to key informants, S. festivus, L. hexandra Sw, T. triandra, B. insculpta, and E. papposa were perceived as species of low preference and palatability, while the remaining grass species were perceived as moderately preferred and palatable species in the study area.
Table 5. Rank of herbaceous species according to Adola Reedde pastoralists’ perception.
Correlation between ranks of key informants’ perceptions and the nutritional value of grass species across three agro-climates
The correlation coefficient between the key informant’s perception ranking and the nutritive value of the common grass species is presented in . There was inconsistency in key informants’ perception of agro-climates. The ranking value of individual grass species based on the perception of key informants was positively correlated with the laboratory results of dry matter (DM), crude protein (CP), relative feed value (RFV), metabolizable energy (ME), in vitro dry matter digestibility (IVDMD), and ash of grass species from highland and mid-altitude agro-climate, but negatively correlated with neutral detergent fibre (NDF), acid detergent fibre (ADF) and acid detergent lignin (ADL). The laboratory results of neutral detergent fibre (NDF), acid detergent fibre (ADF) and acid detergent lignin (ADL) and ash were positively correlated with key informants’ ranking values at lowland agro-climate but negatively correlated with dry matter (DM), crude protein (CP), relative feed value (RFV, metabolizable energy (ME), and in vitro dry matter digestibility (IVDMD).
Table 6. The correlation coefficient between farmers perception’s and the nutritional value of grass species.
The correlation between the different parameters of the nutritional value of the grass species is given in . The results indicated that CP was positively correlated with RFV at (r = 0.935, p < 0.001), ME at (r = 0.961, p < 0.001), and IVDMD at (r = 0.961, p < 0.001) respectively, while negatively correlated with NDF at (r = −0.951, p < 0.001), ADF at (r = −0.922, p < 0.001), ADL at (r = −0.852, p < 0.001), DM at (r = −0.196, p < 0.001), and Ash at (r = −0.924; P < 0.05). There was also a positive correlation between structural constituents of herbaceous plants viz, NDF, ADF, and ADL.
Table 7. The correlation between different parameters of the nutritional value of the grass species across three agro-climates.
Discussion
Chemical composition of grass species and their correlation with the perception ranking of key informants across three agro-climates
All the grass species recorded higher values of crude protein (CP) at highland than midland and lowland agro-climate, while the reverse was true for neutral detergent fibre (NDF), acid detergent fibre (ADF) and acid detergent lignin (ADL). The probable reason for the highest crude protein (CP) content in the highland agro-climate may be attributed to the relatively mid-stage maturity of grass species at harvest compared to the lowland agro-climate, which maintains the structural constituents of herbaceous plants. On the other hand, grass species of lowland agro-climate had an early stage of maturity, which is attributed to the high development of fibre constituents at the same harvesting time. The variation in chemical constituents may also be associated with differences in temperature, precipitation and soil characteristics (Asmare et al. Citation2017), where the growth of plant community and quality were affected markedly by temperature and soil moisture conditions. Improper rangeland management may also result in severe deterioration in the nutritive values of herbaceous plants, land degradation, reduced biodiversity, and gradual replacement of local grasses by undesirable species (Alemayehu Citation2004; Amaha Citation2006; Abate Citation2007; Asrat et al. Citation2015).
Variation in nutritional qualities of different grass species exhibited in the current study could be due to the inherent nature of each species, morphological and anatomical differences among grass species within the same agro-climate. Species and agro-ecological variation are common factors that affect the nutritional value of native forage species (Desalew Citation2008; Subhalakshmi et al. Citation2011; Taklu et al. Citation2010; Asrat et al. Citation2015). Teka et al. (Citation2012) reported that variation in the nutritional value of herbaceous species might also be attributed to the potential of the area in terms of variation in soil characteristics and temperature situations that arise from agro-ecological differences. Soil nutrient status, grazing pressure, management aspects, production location, and spatial distributions of grasses also affect the quality of common herbaceous species (Vander Westhuizen et al. Citation2005; Henkin et al. Citation2011; Tessema et al. Citation2011; Keba et al. Citation2013).
The reported mean value of crude protein (CP) was generally within the recommended range (60–80 g/kg DM), considered satisfactory for maintenance requirements of domestic herbivores (Esmaeli and Ebrahimi Citation2003; Hussain and Durrani Citation2009; Keba et al. Citation2013), but lower than the critical limit of 100.6 g/kg DM proposed by Minson (Citation1990). McDonald et al. (Citation2002) and Sampaio et al. (Citation2009) also recommended that the minimum required level of crude protein (CP) content of grass species for optimal rumen functioning and microbial activity is 70–80 g/kg DM. The crude protein (CP) content of grass is compared to that reported by Gelaye (Citation2015), which indicated a mean value of 79.8 g/kg DM crude protein (CP) content of grass species during the wet and dry seasons, respectively, in the rangeland of Gambella. The result of crude protein (CP) is also in line with the finding of Mlay et al. (Citation2006) that recommended the crude protein (CP) content of tropical grass species is in the range of 40–150.1 g/kg DM.
The mean value of dry matter (DM) content reported in this study is in line with the report of Gelaye (Citation2015), which indicated the mean value of dry matter (DM) content of grass species of Gambella rangelands to be 93.19% during the wet season and decreased to 89.65% during the dry season. The result of the highest mean value of dry matter (DM) exhibited in lowland agro-climate is in line with the finding of other studies (Alemu et al. Citation2007; Asmare et al. Citation2017) for other types of grasses. The highest mean value of dry matter (DM) in lowland agro-ecologies might be attributed to an advanced growth and development of grasses and decreased moisture content compared to highland and midland agro-climates.
The mean neutral detergent fibre (NDF) value of 662 g/kg DM in this study was beyond a threshold level (600 g/kg DM) that declines voluntary feed intake, prolonged rumination time, and decreases the efficiency of conversion of metabolizable energy to net energy. This result is in line with the results reported by Geleti et al. (Citation2012), who noted a mean neutral detergent fibre (NDF) value of 760 g/kg DM for grass species that exceeds the threshold level and they exhibit the lowest voluntary feed intake. The higher mean value of neutral detergent fibre (NDF), acid detergent fibre (ADF) and acid detergent lignin (ADL) contents of grass species in the study area are attributed to the higher percentage of structural carbohydrates and a higher proportion of stems. The reported mean value of ash in this study is in line with Mlay et al. (Citation2006), which ranges from 65 to 140 g/kg DM. The highest ash in the lowland agro-climate may be attributed to the soil type due to the accumulation of topsoil by flooding and erratic rainfall down the lower gradient.
The average in vitro dry matter digestibility (IVDMD) value (426.7–467.9 g/kg DM) recorded in this study was comparable with those reported by Geleti et al. (Citation2012) and lower than the values reported by Gemiyo et al. (Citation2013). This difference could result from wide variations in topography, elevation, rainfall distribution, soil fertility, management conditions and probable variations in the maturity stage of grass species. The highest metabolizable energy (ME) values at the highland agro-climate attribute to the highest in vitro dry matter digestibility (IVDMD) at the highland agro-climate than midland and lowland agro-climates. As cited by Geleti et al. (Citation2012), Leng (Citation1990) and Adugna and Said (Citation1994) indicated that forage with respective crude protein (CP), and digestibility values lower than 80 and 550 g/kg DM are categorized under low-quality forages. As a result, they exhibit low intake, digestibility and poor utilization of dry feed matter (DM). Therefore, in terms of crude protein (CP) and digestibility values, grass species studied in the current study fall under low-quality forages.
The reported overall average value of relative feed value (RFV) (60.97) is in agreement with the report of Keba et al. (Citation2013). However, this was apparently below the threshold level of 100 according to the report of Dunham (Citation1998) and below 151 reported by other researchers (Redfearn and Zhang Citation2011). On the other hand, the present result was higher than the reports of Ørskov (Citation2000), who suggest a relative feed value (RFV) of 30 can provide sufficient energy for maintenance. The highest relative feed value (RFV) in the highland agro-climate may be attributed to the lowest recorded value of neutral detergent fibre (NDF), acid detergent fibre (ADF) and the highest recorded value of crude protein in highland agro-ecology than midland and lowland agro-ecologies.
Dry matter yield of grass species
The high total dry matter (DM) yield and dry matter (DM) of individual grass in highland than lowland in the current study are linked to the impact of farming. In the highland agro-climate, there is a mixed farming (crop-livestock) system that minimizes the pressure of grazing compared to the lowland agro-climate, where farmers own several animals as a source of income and food that increases the pressure of grazing. Grazers owned in lowland agro-climate could also affect plant community structure, primary production, growth, and turnover of its roots, microbes, and animals that control the decomposition of dead organic matter in the soil (Frank and McNaughton Citation1993; Frank et al. Citation2002; Hellström et al. Citation2003; Sankaran and Augustine Citation2004; Patra Citation2005). The pressure of grazing at lowland agro-climate could also result in removing plant shoot tissue (defoliation) (Frank and McNaughton Citation1993). Defoliation affects plant growth and carbon allocation (Briske et al. Citation1996; Ferraro and Oesterheld Citation2002) and reduces the input of aboveground litter to the soil and the amount of coarse organic matter in the soil (Burke et al. Citation1999).
The low dry matter (DM) yield of grass species in the lowland compared to highland agro-ecology corresponds to the reports of Abate (Citation2007) and Asrat et al. (Citation2015), who suggested that rangelands in deprived conditions had low pasture production with unpalatable pastures than those rangelands under good conditions. The low dry matter yield of herbaceous plant species in lowland agro-climate can be generally attributed to poor management practices and soil fertility, inadequate and erratic rainfall, recurrent drought and high grazing pressure. The present findings are in line with previous studies (Dalle et al. Citation2006; Shenkute et al. Citation2012; Rasool et al. Citation2013; Asrat et al. Citation2015). This result is also in line with the report of Oba et al. (Citation2000) and Keba et al. (Citation2013), who indicated that the decline in biomass of grass species arises from the cumulative effect of grazing pressure, which reduces the available biomass for the process of decomposition in the soil and aggravates the encroachment of bush in grazing land by reducing soil organic matter content. Variations in dry matter (DM) production across species may also be attributed to variation in growth rate, growth habit and reduced biodiversity of rangeland, which are mediated through the genotype and phenotypic differences (Opiyo Citation2007; Mganga Citation2009; Ogillo Citation2010). Scarce and erratic rainfall also limit the growth of natural pasture species and dry matter yield in rangeland areas (Lenz and Facell Citation2006; Robles et al. Citation2008; Boufennara et al. Citation2012).
Grass species quality as perceived by the farmers
In the present study, although interviewed key informants lack knowledge related to physiological functions and anatomical characteristics of livestock, they have developed the ability to identify common grass and rank them according to their preferences and palatability because of the long experiences acquired through the years. This result is in line with the finding of Bothma (Citation2010), who suggested that feed selection by livestock is primarily influenced by two factors: palatability and preference for feeds.
The correlation of the ranking value of farmer’s perceptions with laboratory analysis results of grass species at highland and midland agro-climate indicated that farmers are familiar with grass species quality based on preferences and palatability of grass species by a particular class of livestock. However, there was some variation among farmers in lowland agro-climate. This inconsistency in farmer perception might result from heterogeneity in species composition, topographical difference, land-use patterns and soil characteristics of varying agro-climates of the study area. The correlation result was not in agreement with Keba et al. (Citation2013), who suggested a strong correlation between species ranking value and nutritional value of grass species based on pastoral perception in semi-arid rangelands of Ethiopia. Indigenous knowledge refers to the traditional or local acquaintance existing within and accustomed to the specific conditions of indigenous people in particular geographic areas (Leonti et al. Citation2003). Disagreement and inconsistency in the present study might result from variation in the perception of different communities on particular species of their particular area.
Conclusion
Crude protein values were higher at highland than midland and lowland agro-ecology for all grass species. The crude protein content of herbaceous species was adequate for the maintenance requirements of most domestic herbivores at high land agro-climate but considerably declined towards lowland agro-climate. The structural carbohydrate (neutral detergent fibre, acid detergent fibre, acid detergent lignin) and dry matter (DM) in the sampled herbaceous species were higher in lowland agro-climate, with critical implications for forage quality and the sustainability of livestock production. Overall, the low accumulation of crude protein and increased structural fibres were a clue to the poor quality of forage. The nutritional quality of herbaceous forage is more affected by the stage of maturity, which results from agro-ecological differences, than any other factor that was pronounced in lowland agro-climate. Grass quality attributes generally indicate that the vegetation is poor in providing forage biomass capable of meeting livestock nutritional quality requirements.
The correlation of ranking value with laboratory analysis results was inconsistent across agro-climate except in highland and midland agro-climates. This might result from heterogeneity in species composition, spatial variation in topography, land-use patterns, soil characteristics and variation in the perception of different communities in particular species of their particular area. Dry matter yield of grass species implied that the status of grasslands in the lowland agro-climate was in poor condition compared to midland and highland agro-climate due to relatively low rainfall, high temperature, and grazing pressure. Generally, the results of this study highlight the potential of the herbaceous species to support ruminant livestock production, particularly in semi-arid areas where prevailing climate change/variability and global warming impact forage species availability if the rangeland/grassland is properly rehabilitated and managed.
Recommendation
Our findings indicated a need for protein and energy supplementation, rangeland improvement for in situ conservation of key forage species by use of holistic planned grazing and enclosure. Our study also indicated a need for documentation and integration of local knowledge of farmers into rangeland resources management to understand the prevailing land-use impacts, develop new insights into improving existing scientific research, designing proper research and development policies for improvement, sustainable utilization, and conservation of natural resources and in turn alleviating the problem of food and feed shortage/scarcity.
Acknowledgements
We would like to express our gratitude and appreciation to all key informants for their supports during vernacular naming and ranking of common grasses as per their indigenous perception.
Disclosure statement
No potential conflict of interest was reported by the author(s ).
References
- Abate T. 2007. Traditional utilization practices and condition assessment of then rangelands in Rayitu district of Bale zone, Ethiopia [dissertion]. Dire Dewa, Ethiopia: Haramaya University. 129.
- Abule E. 2003. Rangeland evaluation in relation to pastoralists’ perceptions in the Middle Awash Valley of Ethiopia [dissertion]. Bloemfontein, South Africa: University of the Free State.
- Abusuwar AO, Ahmed EO. 2010. Seasonal variability in nutritive value of ruminant diets under open grazing system in the semi-arid rangeland of Sudan (South Darfur State). Agric Biol J North Am. 1(3):243–249.
- Adugna T, Said AN. 1994. Assessment of feed resources in Welayita Sodo. J Agric Sci. 14:69–87.
- Adugna T, Yami A, Dawit A. 2012. Livestock feed resources in Ethiopia: Challenges, opportunities and the need for transformation. Ethiopia Animal Feed Industry Association, Addis Ababa, Ethiopia.
- Adugna T. 2007. Feed resources for producing export quality meat and livestock in Ethiopia. Ethiopia Sanitary and Phytosanitary Standards and Livestock and Meat Marketing project (SPS-LMM). 77.
- Adugna T. 2008. Feed resources and feeding management: A manual for feedlot operators and development workers. PS-LMM Program, Addis Ababa.
- Alemayehu M. 2003. International Livestock Development Project (ILDP). Livestock Feed Resources Survey. North Gonder, Ethiopia. 75.
- Alemayehu M. 2004. Rangelands biodiversity: concepts, approaches and the way forward. Addis Ababa, Ethiopia. 109.
- Alemu B, Solomon M, Prasad NK. 2007. Effects of varying seed proportions and harvesting stages on biological compatibility and forage yield of oats (Avena sativa L. and vetch (Vicia villosa R.) mixtures. Livestock Res Rural Dev. 19:1–9.
- Allen VG, Batello C, Berretta EJ, Hodgson J, Kothmann M, Li X, McIvor J, Milne J, Morris C, Peeters A, Sanderson M. 2011. An international terminology for grazing lands and grazing animals. Grass Forage Sci. 66:2–28.
- Amaha K. 2006. Characterization of rangeland resources and dynamics of the pastoral production system in the Somali region of eastern Ethiopia. PhD Thesis. Free State University,Bloemfontein, South Africa. 249.
- Angassa A, Oba G. 2010. Effect of grazing pressure, age of enclosures and seasonality on bush cover dynamics and vegetation composition in Southern Ethiopia. J Arid Environ. 74:111–120.
- AOAC (Association of official Analytical Chemists). 1990. Official Methods of Analysis. (K. Helrich, Ed.) (15th ed., Vol. 1). Arlington, Virginia, USA.
- Aschalew S. 2014. Smallholder farmers adaptation strategies to climate change in Ethiopia: evidence from Adola Rede Woreda, Oromia Region. J Econ Sustainable Dev. 5(7):166–168.
- Asmare B, Demeke S, Tolemariam T, Tegegne F, Haile A, Wamatu J. 2017. Effects of altitude and harvesting dates on morphological characteristics, yield and nutritive value of Desho grass (Pennisetum pedicellatum Trin.) in Ethiopia. J Agric Nat Res. 51:148–153.
- Asrat M, Angassa A, Abebe A. 2015. The effects of area enclosures on rangeland condition, Herbaceous biomass and nutritional quality in Southeast Ethiopia. School of Animal and Range Sciences, College of Agriculture, Hawassa University. Sci, Technol Arts Res J. 4(2):79–88.
- Association of official Analytical Chemists. 1990. Official Methods of Analysis. (K. Helrich, Ed.) (15th ed., Vol. 1). Arlington, Virginia, USA.
- Beever DE, Mould FL. 2000. Forage evaluation for efficient ruminant livestock production. In: Givens DI, Owen E, Axford RFE, Omed HM, editor. Forage Evaluation in Ruminant Nutrition. Wallingford: CAB International; p. 15–43.
- Bothma JP. 2010. Game ranch management. J.L. van Schaik Publishers, Pretoria.
- Boufennara S, Lopez S, Bousseboua H, Bodas R, Bouazza L. 2012. Chemical composition and digestibility of some browse plant species collected from Algerian arid rangelands. Spanish J Agric Res. 10(1):88–98.
- Briske DD, Boutton TW, Wang Z. 1996. Contribution of flexible allocation priorities to herbivory tolerance in C4 perennial grasses: an evaluation with C labeling. Oecologia. 105:151–159.
- Burke IC, Lauenroth WK, Riggle R, Brannen P, Madigan B, Beard S. 1999. Spatial variability of soil properties in the shortgrass steppe: the relative importance of topography, grazing, microsite, and plant species in controlling spatial patterns. Ecosystems. 2:422–438.
- Central Statistical Agency. 2017/18. Federal democratic republic of Ethiopia, agricultural sample survey: report on Livestock and Livestock characteristics (Private peasant holdings). Addis Ababa, Ethiopia. 2:9–23.
- Dalle G, Maass BL, Isselstein J. 2006. Rangeland condition and trend in the semi- arid Borana lowlands, Southern Oromia, Ethiopia. Afr J Range Forage Sci. 23:49–58.
- Desalew T. 2008. Assessment of feed resources and rangeland condition in Metema district on North Gonder Zone, Ethiopia [dissertion]. Dire Dewa, Ethiopia: Haramaya University. 161.
- Dunham JR. 1998. Relative feed value measures forage quality. Forage Facts, # 41.
- Eaton DP, Santos SA, Santos MCAA, Lima JB, Keuroghlian, A. 2011. Rotational grazing of native pasturelands in the Pantanal: an effective conservation tool. Tropical Conservation Science. 4 (1): 39-52.
- Esmaeli N, Ebrahimi A. 2003. Necessity of determining animal unit requirement based on the quality of forage. Iranian Journal of Natural Resources. 55:579–596.
- Ferraro DO, Oesterheld M. 2002. Effect of defoliation on grass growth. A quantitative review. Oikos. 98:125–133.
- Fowler J, Cohen L. 1996. Practical statistics for field biology. Chichester: John Wiley and Sons.
- Frank DA, Kuns MM, Guido DR. 2002. Consumer control of grassland plant production. Ecology. 83:602–606.
- Frank DA, McNaughton SJ. 1993. Evidence for the promotion of aboveground grassland production by native large herbivores in Yellowstone national park. Oecologia. 96:157–161.
- Gelaye KT. 2015. Seasonal dynamics in botanical composition, nutritive value of vegetation and soil nutrient status of Gambella rangelands, South Western Ethiopia [dissertion]. Debre Zeit, Ethiopia: Addis Ababa University, College of Veterinary Medicine and Agriculture, Department of Animal Production Studies.
- Geleti D, Mekonnen H, Mengistu A, Tolera A. 2012. Herbage yield, species diversity and quality of native grazing land vegetation under sub humid climatic conditions of Western Ethiopia. J Agric Res Dev. 2(4):96–100.
- Gemiyo D, Hassen A, Kocho T, Birhanu T, Bassa Z, Jimma A. 2013. Chemical composition and digestibility of major feed resources in mixed farming system of Southern Ethiopia. World Appl Sci J. 26(2):267–275.
- Getnet A. 2003. Feed resource development and utilization: possible options and recommendations under Ethiopian condition. training handout prepared for agricultural Subject Matter Specialists, holeta Agricultural Research Centre, Holeta, Ethiopia. 1–37.
- Guji Zone. 2014. Socio economic profile of Guji Zone.
- Hassen A. 2006. Assessment and Utilization Practices of Feed Resources in Basona Worana Woreda of North Showa [dissertion]. Haramaya, Ethiopia: Haramaya University.
- Hedberg I, Edwards S. 1989. Flora of Ethiopia. The National Herbarium, Addis Ababa, Ethiopia. 3: 660.
- Hedberg I, Edwards S. 1995. Flora of Ethiopia and Eritrea, Addis Ababa, Ethiopia. 7.
- Hellström K, Huhta AP, Rautio P, Tuomi J, Oksanen J, Laine K. 2003. Use of sheep grazing in the restoration of semi-natural meadows in northern Finland. Appl Veg Sci. 6:45–52.
- Henkin N, Ungar ED, Dvash L, Perevolotsky A, Yehuda Y, Sternbergs M, Voet H, Landau SY. 2011. Effects of cattle grazing on herbage quality in a herbaceous Mediterranean rangeland. Grass Forage Sci. 66:516–525.
- Hussain F, Durrani MJ. 2009. Seasonal availability, palatability and animal preferences of forage plants in Harboi arid range land, Kalat, Pakistan. Pak J Bot. 41(2):539–554.
- Keba HT, Madakadze IC, Angassa A, Hassen A. 2013. Nutritive value of grasses in semi-arid rangelands of Ethiopia: local experience based herbage preference evaluation versus laboratory analysis. Faculty of natural and Agricultural sciences, Department of plant production and soil science, University of Pretoria, P. Bag X20 Hatfield, Pretoria, 0028, South Africa. Asian-Australas J Anim Sci. 26(3):366–377.
- Leng RA. 1990. Factors affecting the utilization of poor quality forage by ruminants particularly under tropical condition. Nutr Res Rev. 3:277–303.
- Lenz TI, Facell IJM. 2006. Correlations between environmental factors, the biomass of exotic annual grasses and the frequency of native perennial grasses. Aust J Bot. 54:655–667.
- Leonti M, Sticher O, Heinrich M. 2003. Antiquity of medicinal plant usage in two Macro- Mayan ethnic groups (México). J Ethno Pharmacol. 88:119–124.
- McDonald P, Edwards RA, Greenhalgh JFD, Morgan CA. 2002. Animal Nutrition, sixth edition. Ashford Colour Press Ltd, Gosport.693.
- Mganga KZ. 2009. Impact of grass reseeding technology on rehabilitation of degraded rangelands: A case study of Kibwezi district, Kenya [dissertion]. Nairobi, Kenya: University of Nairobi.
- Minson DJ. 1990. Forage in ruminant nutrition. (Ed. T. J. Conha). Academic Press, Inc., Javanorich Publishers, USA. 60–263.
- Mlay PS, Pereka A, Phiri EC, Balthazary S, Igusti J, Hvelplund T, Weisbjerg MR, Madsen J. 2006. Feed value of selected tropical grasses, legumes and concentrates. Vet Arh. 76(1):53–63.
- Oba G, Post E, Syvertsen PO, Stenseth NC. 2000. Bush cover and range condition assessments in relation to landscape and grazing in southern Ethiopia. Land Scape Ecol. 15:535–546.
- Oddy VU, Roberts GE, Low SG. 1983. Prediction of in-vivo dry matter digestibility from fiber and nitrogen content of feed. Common Wealth Agriculture Bureax, Australia. 295–298.
- Ogillo BP. 2010. Evaluating performance of range grasses under different micro catchments and financial returns from reseeding in Southern Kenya [dissertion]. Nairobi, Kenya: University of Nairobi.
- Opiyo FO. 2007. Land treatment effects on morphometric characteristics of three grass species and economic returns from reseeding in Kitui District, Kenya [dissertion]. Nairobi, Kenya: University of Nairobi.
- Ørskov ER. 2000. The in situ technique for the estimation of forage degradability in ruminants. In: Givens DI, Owen E, Axford RFE, Omed HM, editor. Forage Evaluation in ruminant nutrition. Wallingford: CABI International; p. 175–188.
- Patra AK, et al. 2005. Effects of grazing on microbial functional groups involved in soil N dynamics. Ecol Monogr. 75:65–80.
- Rasool F, Khan ZH, Ishaque M, Hussain Z, Khalid KM, Saleem MS, Farooq M, Bashai M. 2013. Assessment of nutritional status in selected indigenous and exotic rangeland grasses. World Appl Sci J. 21(5):795–801. ISSN 1818-4952.
- Redfearn D, Zhang H. 2011. Forage Quality interpretations, Oklahoma cooperative extension service, PSS 2117. Relative feed value and quality index. In: Proceeding of Florida Ruminant Nutrition Symposium, University of Florida, Gainesville. 16–31.
- Robles AB, Ruiz-Mirazo J, Ramos ME, González-Rebollar JL. 2008. Role of livestock grazing in sustainable use, naturalness promotion in naturalization of marginal ecosystems of southeastern Spain (Andalusia). In: Rigueiro-Rodríguez A, McAdam J and Mosquera-Losada MR (Eds) Agroforestry in Europe, Current status and future prospects. Advances in Agroforestry 6: 211–231.
- Sampaio CB, Detmann E, Paulino MF, Filho SCV, DE Souza, MA, Lazzari NI, Rodrigues Paulino PV, DE Queiroz AC. 2009. Intake and digestibility in cattle fed low-quality tropical forage and supplemented with nitrogenous compounds. Trop Anim Health Prod. 42:1471–1479.
- Sankaran M, Augustine DJ. 2004. Large herbivores suppress decomposer abundance in a semiarid grazing ecosystem. Ecology. 85:1052–1061.
- Sanson DW, Kercher CJ. 1996. Validation of equations used to estimate relative feed value of alfalfa Hay. The Prof Anim Scientist. 12(3):162–166. doi:10.15232/S1080-7446(15)32512-2.
- SAS Institute. 2008. Statistical analysis software. Cary, NC: SAS Institute Inc.
- Schut A, Gherardi S, Wood D. 2010. Empirical models to quantify the nutritive characteristics of annual pastures in south-west Western Australia. Crop and Pasture Sci. 61:32–43.
- Shenkute B, Abubeker H, Assefa T, Amen N, Ebro A. 2012. Identification and nutritive value of potential of fodder trees and shrubs in the mid rift valley of Ethiopia. J Anim Plant Sci. 22:1126–1132.
- Solomon M, Teferi A. 2010. Chemical composition, in vitro dry matter digestibility and in sacco degradability of selected browse species used as animal feeds under semi-arid conditions in 12. Goering HK and PJ Van Soest, 1970. Forage Fiber Northern Ethiopia, Agroforest Syst. 80:173–184.
- Stalling CC. 2005. Test available for measuring forage quality. Virginia Cooperative Extension Dairy Guideline 404-124. Available from: https://vtechworks.lib.vt.edu/bitstream/handle/10919/48380/404-124_pdf.pdf.
- Subhalakshmi B, Bhuyan R, Sama DN, Sharma KK, Bora A. 2011. Effect of variety and stag of harvest on yield, chemical composition and in vitro digestibility of hybrid Napier (Pennisetum purpureum x P. americanum). Indian J Anim Nutr. 28:418–420.
- Taklu B, Negesse T, Ayana A. 2010. Effect of farming system on floristic composition, yield and nutritional content of forages at the natural pasture of Assosa Zone. Trop Subtropical Agroecosyst. 12:583–592.
- Teka H, Madakadze IC, Ayana A, Hassen A. 2012. Effect of seasonal variation on the nutritional quality of key herbaceous species in semi-arid areas of Borana, Ethiopia. Indian J Anim Nutr. 29(4):324–332.
- Tessema ZK, de Boer WF, Baars RMT, Prins HHT. 2011. Changes in soil nutrients, vegetation structure and herbaceous biomass in response to grazing in a semi-arid savanna of Ethiopia. Journal of Arid Environment. 75:662–670.
- Tilley JMA, Terry RA. 1963. A two stage technique for in vitro digestion of forage crops. J Br Grassland Soc. 18:104–111.
- Vander Westhuizen HC, Snyman HA, Fouchè HJ. 2005. A degradation gradient for the assessment of rangeland condition of a semi-arid sour veld in Southern Africa. Afr J Range Forage Sci. 22:47–58.
- Van Soest PJ, Robertson JB. 1985. Analysis of Forages and Fibrous Feeds. Laboratory Manual for Animal Science 613, Cornell University, Ithaca, New York. 202.
- Van Soest PJ, Robertson JB, Lewis B. 1991. Methods for dietary fiber, neutral detergent fiber, and non-starch polysaccharides in relation to animal nutrition. J Dairy Sci. 74:3583–3597.
- Wondatir Z, Yoseph M. 2014. Feed resources availability and livestock production in the central rift valley of Ethiopia. Int J Livestock Prod. 5(2):30–36.
- Zewdie W. 2010. Livestock production systems in relation with feed availability in the Highlands and Central Rift valley of Ethiopia. MSc thesis. Haramaya University, Haramaya, Ethiopia.