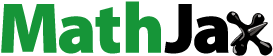
ABSTRACT
The availability of fossil shell flour(FSF) has increased its use as a natural feed additive in livestock diets. Therefore, identifying its optimum inclusion levels in livestock production is essential for animal productivity. This study investigated the effects of various fossil shell flour (FSF) inclusion levels on in vitro digestibility, relative feed values and rumen parameters of Dohne-Merino wethers. Twenty-four fistulated wethers, averagely 20 ± 1·5 kg body weight, were used in a complete randomized design. They were fed a basal diet without fossil shell flour (control, 0%) or with the addition of 2% FSF (T2), 4% FSF(T3) and 6% FSF (T4) of diet DM for 35 days. The results showed that increasing FSF levels had no effect on ruminal T0C or pH, but Ammonia-N increased (P < 0.01) with increasing FSF. The total molar concentrations of volatile fatty acids (VFA) decreased (P < 0.05) with increasing levels of FSF. IVTDDM, IVTDNDF and IVTDADF decreased to 4% FSF inclusion but tended to increase (P = 0.06) at 6% inclusion. Relative feed values of the diets tended to increase (P = 0.07) by adding fossil shell flour. In conclusion, adding FSF to the sheep diet had no adverse effects on the rumen parameters, though in vitro true digestibility could be reduced.
Introduction
Small ruminants, especially sheep, are reared and maintained in most sub-Saharan African countries on high roughage-based diets with limited access to concentrate supplements (Yang et al., Citation2018). This has led to reduced production performance, low meat quality, and subsequent economic losses to farmers and the nation. Therefore, improving feed utilization in small ruminant nutrition is one of the essential features that can improve farming profitability. One of the most popular strategies for improving feed utilization is the use of feed additives, including antibiotics, live yeast, enzymes (both fungal and bacterial sources), plant extracts, algae, bentonites, and fossil shell flour (Abdel-Aziz et al., Citation2015; Durmic et al., Citation2014; Valdes et al., Citation2015).
Similarly, Frater (Citation2014) reported that feed additives had been shown to stabilize rumen pH, reduce the risk of acidosis, increase dry matter intake (DMI), reduce methanogenesis, enhance rumen development, and improve meat quality. Manipulating the rumen microbial ecosystem to improve ruminants’ performance is essential for animal nutritionists. Thus, it is essential to identify feed additives that can modify rumen fermentation and efficiency of feed utilization while decreasing nitrogen excretion.
The removal of antibiotic growth-promoters as feed additives in ruminant nutrition in most advanced countries has led to the use of plant extracts, live yeast, enzymes or other organic additives to manipulate ruminal fermentation (Martin and Kadokawa, Citation2006; McGrath et al., Citation2018). These manipulations can promote feed efficiency and animal growth, and prevent, control, and treat diseases (Wang and Wang, Citation2016). The availability and cost implications of plant extracts, live yeast, enzymes, and other bioorganic feed additives have been challenging. However, using organic feed additives such as fossil shell flour in livestock diets is becoming popular recently. This is because it is assumed to leave no residue in the products of animals that consume it, is abundantly available, eco-friendly and is relatively cheap compared to inorganic feed additives (Ikusika et., 2019). Fossil shell flour (FSF) as feed additive increases feed intake, average daily weight gain, and overall performance of livestock because of its mineral richness (mainly silicon-oxide) (Mwanda et al.,2020). However, the effect of various FSF inclusion levels on the rumen environmental and fermentation parameters, in vitro true digestibility, and relative feed value is unknown. Therefore, this study investigated the effects of different inclusion levels of fossil shell flour on feed quality, in vitro true digestibility, and ruminal fermentation parameters in Dohne-Merino wethers. It was hypothesized that fossil shell flour in Dohne Merino wethers’ diets would improve feed quality, in vitro true digestibility, and ruminal fermentation parameters.
Materials and methods
Ethical approval
The University of Fort Hare approved the handling and use of animals (Ethically approved number – MPE041IKU01).
Study site description
The Experiment was conducted at the Small Ruminant Unit of the University of Fort Hare Teaching and Research Farm (Animal Section), Alice, Eastern Cape, South Africa. The research farm is located along Alice-Kings Williams's town, Alice, which lies at a longitude 26o50’ E and latitude of 32°46’ S. The annual rainfall is between 480-490 mm and a temperature range between 24.6 and 11.1 oC (average is 17.8 oC) at the altitude of 535 meters above sea level. The in vitro true digestibility using Daisy200 incubator was conducted at the Ruminant Nutritional Laboratory of the University of Zululand, Empangeni, KwaZulu Natal Province, South Africa.
Animals, experimental design, and management
Twenty-four single-stage rumen-cannulated Dohne-Merino wethers were randomly allotted into four different diets in a completely randomized design with six wethers per diet. The donor wethers were fed a basal diet without FSF addition 0% or FSF 2%, 4%, or 6% of diet DM. The wethers were fed the experimental diets 35 days before the rumen fluid collection. The ruminal fluid was collected from the cannulated wethers before morning feeding (7:30 am). Approximately 500 ml of rumen fluid were obtained from each fistulated wether, which allowed manually collected ruminal digesta to be strained through 4 layers of cheesecloth.
Experimental diets
The diets for the wethers consisted of concentrate and hay at a 40:60 ratio. The concentrate was made up of maize (8%), sunflower oil cake (10%), molasses (5%), wheat offal (15%), limestone (1.5%), salt 0.3%, and sheep mineral-vitamin premix (0.2%), whereas the hay consisted of 30% teff and 30% Lucerne. The ingredients for concentrate were purchased from Monti Feeds (pty) Ltd, East London, South Africa. In contrast, the teff and Lucerne were purchased from Umtiza Agricultural Products (Pty) Ltd, Kwantu shopping mall, Alice, South Africa. All ingredients were thoroughly milled and mixed evenly to form the basal diet. The feed was formulated to meet the used sheep's nutritional (energy and protein) requirements (NRC, Citation2007). The four dietary groups were: basal diet (0%); basal diet +2% FSF; basal diet +4% FSF and basal diet + 6% FSF. The wethers were fed at 8:00 h and 15:00 h at 4% of the body weight (on a dry matter (DM) basis). The food-grade Fossil shell flour was purchased from Eco-Earth (Pty) Ltd, Port Elizabeth, South Africa, which produces this product under a license by the Department of Agriculture, Forestry and Fisheries of South Africa. shows the feed ingredient and the chemical composition of the experimental diet, while shows the mineral content of the dietary fossil shell flour used in this present experiment.
Table 1. Proximate analysis of the experimental diets
Table 2. Mineral composition of Fossil shell flour (FSF).
Determination of in vitro True digestibility.
Rumen samples were collected from the twenty -four wethers through the fistulation. One-stage techniques for ruminal fistulation described by Ghazy (Citation2017) were used to collect ruminal fluid. The rumen liquor was collected from the sheep, transferred into a thermos flask pre-warmed to a 39 oC and transported immediately to the nutritional laboratory. The incubation procedure was carried out using the DAISY200 Incubator ANKOM Technology (2017) method. Each empty F57 bag (25 µm pore size) was weighed (W1) and recorded. Feed samples of 0.5 g each in triplicate (Coblentz et al., Citation2019) from each treatment were carefully put into the F57 bags and recorded as W2. Each bag was then heat-sealed, labelled, and evenly distributed on both sides of the Daisy200 incubator digestion jar with one sealed blank bag in all jars as a correction factor (C1). Then, the samples were pre-warmed at 39 oC with 1330 ml of buffer solution A (10.0 KH2PO4 + 0.5 MgSO4.7H2O +0.5 NaCl + 0.1 CaCl2.2H20 + 0.5 Urea), 270 ml of buffer solution B (15.0 Na2CO3 + 1.0 Na2S.9H2O) and 400 ml of four-layer cheesecloth filtered rumen fluid. This was poured into the digestion jar, purged with CO2 for 30 s, and placed into the Daisy incubator at 39 oC for 48 h. The Daisy incubator temperature was maintained at 39.5 oC ± 0.5. After incubation, the jars were removed from the incubator, the water drained, and the bags were thoroughly rinsed with cold tap water until the water was clean using minimal mechanical agitation. The bags were then dried at 60 oC until constant mass, as described by Paula et al. (Citation2017). These samples were further analyzed for fibre using the ANKOM200 Fiber Analyzer to determine the NDF using the procedure described by Van Soest et al. (Citation1991). In vitro NDF weight was recorded as W3. These values were used to calculate IVTDDM (Invitro true digestibility on a dry matter basis). This formula is taken from the Ankom200 method.
Where W1 = empty bag weight W2 = sample weight
W3 = final bag weight after in vitro and sequential ND treatment
C1 = Blank bag correction (final oven-dried weight/original blank bag weight).
Determination of relative feed values
Relative feed value estimates the digestibility of the dry forage matter and how much the sheep can consume based on its ‘satisfying’ capacity. This was developed in America by Hay Marketing Task Force. It is a key tool in forage marketing and forages quality education. Using the method or equation of Rohweder et al. (Citation1978), the relative feed value (RFV) of the diets was calculated as follows:
DMI = Dry matter intake (Live weight = LW %) = 120/ (NDF %); DMD = Dry matter digestibility (%) = 88.9 – (0.779×ADF %); RFV = Relative feed value = (DMD×DMI)/1.29.
Based on the Quality Grading Standard assigned by the Hay Marketing Task Force of the American Forage and Grassland Council, the RFV was assessed as roughages based on prime >151, 1 (premium) 151-125, 2 (good) 124- 103, 3 (fair) 102-87, 4 (poor) 86-75, 5(reject) < 75.
Determination of rumen fluid parameters (PH, temperature, and volatile fatty acid)
Rumen fluid was collected from twenty-four wethers through a fistula. The pH was immediately measured using a digital pH meter (Sartorius PT-10; Sartorius AG, Göttingen, Germany) and recorded each wether. Ammonia-N was determined from 5 mL of strained (through 4 layers of cheesecloth) rumen fluid pipetted with 1 ml of H2SO4 (20% Conc) for preservation before storing at −20 °C. It was analyzed using the phenol hypochlorite assay (Galyean, Citation2006).
To analyze ruminal VFA, 5 mL of strained rumen fluid-preserved with 1 mL of an acid solution containing 20% orthophosphoric acid and 0.5 formic acids (5% conc.) and stored at – 20°C was used. After thawing, rumen fluid samples were centrifuged (15,000 × g for 15 min at 4 °C), and the supernatant was used to quantify the VFA. The concentration of acetic acid, butyric acid, propionic acid, iso-butyric, valeric acid, and iso-valeric was measured by a gas chromatograph (UNICAM 4600; SB Analytical, Cambridge, UK), following the procedures described by Erwin et al., (Citation1961).
Statistical analysis
Analysis of variance by one-way experimental design within the groups was used to analyze each component's chemical composition, in vitro true digestibility, relative feed values, and rumen parameters (GLM of SAS, 2018). Duncan's multiple range test was done to compare the means, and differences were considered when P < 0.05.
Results
The effects of FSF's varying inclusion levels in diets of Dohne-merino rams on pH, temperature, ammonia-N, and VFA are presented in . Rumen pH increased slightly with FSF but was not different (P > 0.07) within the FSF inclusion levels. The NH3-N concentration was greater (P < 0.01) in 2% and 6% FSF than in 0% and 4%. With increased FSF inclusion levels, the molar total VFAs, acetic, butyric, and iso-butyric values decreased (P >0.05). The 4% FSF had lesser propionic value compared to 2%, 6%, and 0% FSF (P < 0.05). Molar iso-valeric acid in rumen fluid did not change with FSF inclusion (P > .05). The acetate to propionate ratio was highest at 4% FSF and lowest at 6% FSF.
Table 3. Effects of varying inclusion levels of FSF in diets on rumen parameters of Dohne-merino.
shows the nutrient composition of the four diets due to varying inclusion levels of FSF. The non-significant difference between crude protein components showed that FSF inclusion of up to 6% does not affect the nutrient content.
Table 4. Chemical composition of the diets with varying FSF inclusion levels.
shows the in vitro true digestibility of dry matter (IVTDDM) after adding three amounts of FSF to the diets, which consistently decreased (P < 0.05). IVTDOM %, IVTDNDF%, IVTDADF% and IVTDADL% all decreased at different levels of FSF supplementation compared to the control (0%).
Table 5. In vitro true digestibility of Dohne-merino diets with various levels of Fossil shell flour.
IVTDDM: in vitro true dry matter digestibility; IVTDOM: in vitro true organic matter digestibility IVTDNDF: in vitro true neutral detergent fibre digestibility; IVTDADF: in vitro true acid detergent fibre digestibility; IVTDADL: in vitro true acid detergent lignin digestibility.
The effects of including various FSF amounts into Dohne-merino wether diets on in vitro true digestibility and feed quality as defined by their RFV content are presented in . Relative feed value (RFV) and DMI tended to increase (P ≤0.05) as the amounts of FSF added to the diets increased ().
Table 6. Effects of FSF inclusion levels on diet quality.
Discussion
Mean ruminal pH and temperature values were within the normal physiological range of 6.1–6.8 in all experimental lambs, as observed by Van Soest (Citation1994). Imani Rad et al. (Citation2016) and McDonald et al. (Citation2011) reported that factors such as DMI, type of diet, addition or non- addition of an additive, hay to concentrate ratio, and the physical structure of the diets, digestibility, and feeding method affect rumen pH. In this study, these factors were not different among the dietary treatments; thus, there was no change (P ≥0.05) in the rams’ rumen pH. This means that the inclusion of FSF in the diets of Dohne-merino wethers maintained the ruminal pH and ruminal metabolism. This could be because FSF has a buffering capacity, which helps stabilize the rumen pH. The mean ammonia concentration for 2% and 6% FSF values obtained from this study were within the minimum concentration of 5 mg/dL required for maximal growth of microbes in animals’ rumen (Sinclair DA, Dawes IW, Citation1993). The concentration of ammonia-N was affected by the inclusion of FSF at 2% and 6% in the diets of Dohne -merino wethers, compared to the 0% level (P < 0.05). The reason could be that since FSF increased protein available in the rumen (McDonald et al., Citation2011), it follows that there would be degradation of excess crude protein in the rumen, leading to ammonia accumulation in the rumen. This will enhance rumen protozoa growth. This aligns with Pal et al. (Citation2010) study, which reported that when feed protein degradation is depressed in the rumen, protozoa growth is hindered because of a decrease in rumen ammonia concentration.
The main supply of energy to ruminants comes from the end products of fermentation by various microorganisms in the rumen, which is VFA (Imani-Rad et al., Citation2016; Van Soest, Citation1994). Hence, sheep performance and productivity can be enhanced through diets that promote higher production of VFA compared to lesser amounts (Penner, Citation2014). Though the amount of total molar VFA observed in this study was reducing as FSF inclusion levels increased, it still falls within the normal ranges of 60–150 mmol/L as reported by Imani-Rad et al. (Citation2016) and.
Martínez-Fernández et al. (Citation2014). Also, the lowering of total VFAs, acetic acid, butyric, and iso-butyric acid concentration as FSF increases were similar to Partanen and Jalava's (Citation2005) findings. The reduction in molar total VFAs and individuals in rumen fluid may be due to FSF's antimicrobial effects on rumen bacteria. There might be a change in the metabolic pathway through which microbes utilize substrates, reducing the typical VFA by-products (Baltran and Martins, Citation2015; Fernandez et al., Citation1998; Ikusika et al., Citation2019). However, the net reduction is minimal, as the VFA concentration was still within the normal VFA mM ratio.
The diet consisted of maize, sunflower oil cake, wheat bran, molasses, limestone, salt, and premix, while the hay was a combination of teff and Lucerne in equal proportion. Teff and Lucerne have been reported to be the right combination for sheep production, positively impacting performance (Kafilzadeh et al., Citation2012). The NDF, ADF, and ADL observed in this study are within the normal range reported by Gebremariam et al. (Citation2014) on teff and Lucerne. The diet CP% (14.7) was above the average CP% (∼7.5) for ruminant nutrition, as reported by Fitwi and Tadesse (Citation2013). The supplemented feed CP% varied from 15.52 compared to the 55 g/kg DM for teff and 118.0 g/kgDM for Lucerne reported by Ghasemi et al. (Citation2012). This could be due to the addition of concentrate to the diets. The major component of the concentrate, such as sunflower cake and maize, were richer in crude protein than teff and Lucerne. The similarity in the values of the various components shows uniformity in the basal diets, and the only source of variation can simply be FSF levels. However, adding FSF at different inclusion levels in this study did not affect the diets’ chemical composition.
Different feed additives to improve fibrous feeds’ digestibility in ruminants have been reported severally (Aboagye et al., Citation2015; Kondratovich et al., Citation2017; Salman et al., Citation2017). However, there was very little information on using fossil shell flour as an additive for improving rumen parameters and influencing true digestibility and relative feed values in sheep. Emeruwa (Citation2016) reported a significant difference in sheep supplemented with varying amounts of FSF and the control for in vitro organic matter digestibility. It was observed that treatment with 2% inclusion levels had the highest INVTDOM % (62.19%) among all the treatments. This was contrary to the present study's findings, which show that the control treatment had the highest INVTDOM % values. The difference could be attributed to the different feed ingredients used in the two experiments. Both concentrate and hay were used in this trial, while Emeruwa (Citation2016) used concentrate feed only.
The IVTDNDF% is an essential parameter for determining feed intake and quality (Oba and Allen, Citation2011; Salman et al., Citation2017). In this study, the values of IVTDNDF% tended to be higher in the 0% inclusion level than 2%, 4%, and 6% FSF. This means that digestibility decreased with increased FSF. This could be because of the antimicrobial properties of FSF that might have reduced the activities of the ruminal microorganism, such as Ruminococcus albus, Ruminococcus flavefaciens, and Butyrivibrio fibrisolvens that degrade fibrous feeds. The action mechanism was unknown but could be associated with FSF's ion exchange and absorption property, altering the cells’ electrolyte balance. This interruption can disturb ions’ trans-membrane movement and intracellular equilibrium, exhausting and causing some microorganisms’ death.
The observation in this study was similar to the reduction in NDF and ADF values obtained in a trial conducted by Anassori et al. (Citation2012). They investigated the effects of different feed additives on INVTDNDF% and INVTDADF %, and it was reported that Monessin and essential oil decreased NDF and ADF degradability in the Daisy200 digestion method. The reason is that Monessen has an inhibiting effect on gram-positive microorganisms, which include most of the cellulolytic bacteria, capable of hydrolyzing fibre, thereby reducing NDF degradability. This strongly backed FSF's antimicrobial potential, as Ikusika et al.(Citation2019) stated; hence the decrease in INVTD observed in this study.
The feed quality improved with an increase in FSF addition in the diets. All the treatments generally proved to have premium feed quality, as demonstrated by the relative feed value. There was no significant difference between them. The premium-rated feed values obtained in this study resulted from an increase in DMD and DMI values. The inclusion of FSF increases the feed intake of withers, dry matter intake, and dry matter digestibility. The mechanism explaining this was unclear but was associated with FSF richness in minerals such as Na, Ca, Mg, Cu, and Fe (Adebiyi et al., Citation2009).
Moreover, these minerals seem to increase the palatability of the diets (Desimone et al., Citation2013; Garmyn et al., Citation2011), hence, the high feed intake value. This agreed with the finding of Silva et al. (Citation2015) and Moyo et al. (Citation2019), who both reported that mineral supplementation has positive effects on feeding acceptance vis-a-vis the feed intake and the DMI. Similarly, Sahoo et al. (Citation2011) reported that adding mineral supplements to sheep's diets increases the animal's preference for such feed. The addition of FSF in the diets also increased INVTD%. Diets supplemented with FSF have higher INVTD numerical values than the non-supplemented diets (0%). Nevertheless, no significant difference was observed.
Conclusion
From this research work, it may be inferred that adding FSF at varying levels to wethers’ diets containing concentrate and hay (teff and Lucerne) improved feed intakes and feed values of the diets. Also, it has no adverse effects on ruminal parameters and in vitro true digestibility. Therefore, FSF can potentially enhance the production performance of Dohne Merino wethers. The FSF inclusion levels up to 4% could be further explored in vivo experiments.
Authors contribution
Conceptualization; Data curation; Formal analysis; Investigation; Methodology; Project administration; Resources; Software; Supervision, and writing of original draft were carried out by Olusegun Ikusika, while supervision and validation were done Conference T.Mpendulo
Acknowledgements
The authors acknowledged the efforts of some staff in the Department of Livestock and Pasture Science, University of Fort Hare, for their support.
Disclosure statement
No potential conflict of interest was reported by the author(s).
Data availability
Data will be available upon request.
Additional information
Funding
References
- Abdel-Aziz NA, Salem AZM, El-Adawy MM, Camacho LM, Kholif AE, Elghandour MMY, Borhami BE. 2015. Biological treatments as a mean to improve feed utilization in agriculture animals—An overview. J Integr Agric. 14:534–543.
- Aboagye IA, Lynch JP, Church JS, Baah J, Beauchemin KA. 2015. Digestibility and growth performance of sheep fed alfalfa hay treated with fibrolytic enzymes and a ferulic acid esterase producing bacterial additive. Anim Feed Sci Technol. 203:53–66.
- Adebiyi OA, Sokunbi OA, Ewuola EO. 2009. Performance evaluation and bone characteristics of growing cockerel Fed diets containing different levels of diatomaceous earth. Middle East J Sci Res. 4:36–39.
- Anassori E, Dalir-Naghadeh B, Pirmohammadi R, Taghizadeh A, Asri-Rezaei S, Farahmand-Azar S, Besharati M, Tahmoozi M. 2012. In vitro assessment of the digestibility of forage-based sheep diet, supplemented with raw garlic, garlic oil, and monensin. Vet Res Forum. 3:5–11.
- Beltran MA, Martins R. 2015. Original article diatomaceous earth inhibited the. Philipp J Vet Anim Sci. 41:135–140.
- Coblentz W, Akins M. 2019. Comparisons of fiber digestibility for triticale forages at two dierent sample sizes using the Ankom Daisy Incubator II System. In Proceedings of the ADSA Annual Meeting, Cincinnati, OH,USA, 23–26 June 2019. Abstract T69.
- Desimone JA, Beauchamp GK, Drewnowski A, Johnson GH. 2013. Sodium in the food supply: challenges and opportunities. Nutr Rev. 71:52–59.
- Durmic Z, Moate PJ, Eckard R, Revell DK, Williams R, Vercoe PE. 2014. In vitroscreening of selected feed additives, plant essential oils and plant extracts for rumen methane mitigation. J Sci Food Agric. 94:1191–1196.
- Emeruwa CH. 2016. Growth performance of west african dwarf sheep Fed diets supplemented with fossil shell flour. Nigeria: University of Ibadan.
- Erwin ES, Marco GJ. 1961. Volatile fatty acid analyses of blood and rumen fluid by Gas chromatography. J Dairy Sci. 44:1768–1771.
- Fernandez MI, Woodward BW, Stromberg BE. 1998. Effect of diatomaceous earth as an anthelmintic treatment on internal parasites and feedlot performance of beef steers. Anim Sci. 66:635–641.
- Fitwi M, Tadesse G. 2013. Effect of sesame cake supplementation on feed intake, body weight gain, feed conversion efficiency and carcass parameters in the ration of sheep fed on wheat bran and teff (eragrostis teff) straw. Momona Ethiop J Sci. 5:89.
- Frater, P., 2014. Feed additives in ruminant nutrition. Can feed additives reduce methane and improve performance in ruminants? Agriculture and Horticulture Development Board. www.beefandlamb.ahdb.org.uk (Accessed on 18/08/2018)
- Galyean L. 2006. Effects of an artificial sweetener on health, performance, and dietary preference of feedlot cattle1. J Anim Sci. 84:2491–2500.
- Garmyn AJ, Hilton GG, Mateescu RG, Morgan JB, Reecy JM, Tait Jr RG, Beitz DC, Duan Q, Schoonmaker JP, Mayes MS, et al. 2011. Estimation of relationships between mineral concentration and fatty acid composition of longissimus muscle and beef palatability traits1. J Anim Sci. 89:2849–2858.
- Gebremariam M, Zarnkow M, Becker T. 2014. Teff (Eragrostis tef) as a raw material for malting, brewing, and manufacturing of gluten-free foods and beverages: a review. J Food Sci Technol. 51:2881–2895.
- Ghazy, A. 2017. One-Stage Technique for Rumenal Fistulation in Rams. Alex J Vet Sci. 52(1):109. DOI:10.5455/ajvs.325696
- Ghasemi S, Naserian AA, Valizadeh R, Tahmasebi AM, Vakili AR, Behgar M. 2012. Effects of pistachio by-product in replacement of lucerne hay on microbial protein synthesis and fermentative parameters in the rumen of sheep. Anim Prod Sci. 52:1052.
- Ikusika OO, Mpendulo CT, Zindove TJ, Okoh AI. 2019. Fossil shell flour in livestock production: A review. Animals (Basel). 9:1–20.
- Imani Rad M, Rouzbehan Y, Rezaei J. 2016. Effect of dietary replacement of alfalfa with urea-treated almond hulls on intake, growth, digestibility, microbial nitrogen, nitrogen retention, ruminal fermentation, and blood parameters in fattening lambs. J Anim Sci. 94:349–358.
- Kafilzadeh F, Heidary N, Bahraminejad S. 2012. Variety effect on composition, the kinetics of fermentation, and in vitro digestibility of oat (avena sativa L.) straw and its neutral detergent fibre. S Afr J Anim Sci. 42:406–415.
- Kondratovich, L.B., Sarturi, J O., Ballou, M A., Sugg, D., Campanile, P R B., Reis, B Q., Melo, A-C, B., Pellarin, L A., Ovinge, L.A. 2017. 552 effects of fibrolytic enzyme on in-vitro true digestibility of by-products commonly fed to cattle. J Anim Sci 95: 270.
- Martin GB, Kadokawa H. 2006. “Clean, green and ethical” animal production. case study: reproductive efficiency in small ruminants. J Reprod Dev. 52:145–152.
- Martínez-Fernández G, Abecia L, Arco A, Cantalapiedra-Hijar G, Martín-García AI, Molina-Alcaide E, Kindermann M, Duval S, Yáñez-Ruiz DR. 2014. Effects of ethyl-3-nitrooxy propionate and 3-nitrooxypropanol on ruminal fermentation, microbial abundance, and methane emissions in sheep. J Dairy Sci. 97:3790–3799.
- McDonald, P., R.A. Edward, J.F.D. Greenhalgh, C.A. Morgan, L.A.S. 2011. Animal Nutrition, Animal Nut. ed. Prentice-Hall/Pearson Education Ltd, Harlow, UK.
- McGrath J, Duval SM, Tamassia LFM, Kindermann M, Stemmler RT, de Gouvea VN, Acedo TS, Immig I, Williams SN, Celi P. 2018. Nutritional strategies in ruminants: A lifetime approach. Res Vet Sci. 116:28–39.
- Moyo M, Adebayo RA, Nsahlai IV. 2019. Effects of diet and roughage quality, and period of the day on diurnal feeding behaviour patterns of sheep and goats under subtropical conditions. Asian-Australas J Anim Sci. 32:675–690.
- National Research Council (NRC). 2007. Nutrient requirement of small ruminant: sheep, goats, cervids and new world camelids. Washington, DC: National Academy of Science.
- Oba M, Allen M. 2011. The impact of improving NDF digestibility of corn silage on dairy cow performance. Ruminant Nutrition Symposia. 105–117.
- Pal DT, Gowda NKS, Prasad CS, Amarnath R, Bharadwaj U, Suresh Babu G, Sampath KT. 2010. Effect of copper- and zinc-methionine supplementation on bioavailability, mineral status, and tissue concentrations of copper and zinc in ewes. J Trace Elem Med Biol. 24:89–94.
- Partanen K, Jalava T. 2005. Effects of some organic acids and salts on microbial fermentation in the digestive tract of piglets estimated using an in vitro gas production technique. Agric Food Sci. 14:311–324.
- Paula EM, Monteiro HF, Silva, LG, Benedetti, PDB, Daniel JLP. 2017. Effects of replacing soybean meal with canola meal differing in rumen-undegradable protein content on ruminal fermentation and gas production kinetics. Int J Dairy Sci. 100(7):5281–5292
- Penner GB. 2014. There are mechanisms of volatile fatty acid absorption and metabolism and maintenance of a stable rumen environment. In: Proc. 25th Annu. Florida Rumin. Nutr. Symp., Feb. 4–5, Univ. Florida, Gainesville, FL. p. 92–106.
- Rohweder DA, Barnes RF, Jorgensen N. 1978. Proposed hay grading standards based on laboratory analyses for evaluating quality. J Anim Sci. 47:747–759.
- Sahoo A, Soren NM. 2011. Nutrition for wool production. WMC002384. 2(10):1–11.
- Salman M, Cetinkaya N, Selcuk Z, Genc B, Acici M. 2017. Effects of various inulin levels on in vitro digestibility of corn silage, perennial ryegrass (Lolium perenne L.), and common vetch (Vicia sativa L.)/oat (Avena sativa L.) hay. S Afr J Anim Sci. 47:723.
- Silva TPD, Marques CAT, Torreão JNC, Bezerra LR, Araújo MJ, Gottardi FP, Edvan RL, Oliveira RL. 2015. Ingestive behaviour of grazing ewes given two levels of concentrate. S Afr J Anim Sci. 45:180–187.
- Sinclair, D.A., Dawes, IW., 1993. Purification and characterization of the branched-chain alpha-ketoacid dehydrogenase complex from saccharomyces cerevisiae. Biochem Mol Biol Int 31: 911–922.
- Valdes KI, Salem AZM, Lopez S, Alonso MU, Rivero N, Elghandour MMY, Domínguez IA, Ronquillo MG, Kholif AE. 2015. Influence of exogenous enzymes in the presence of Salix babylonica extract on digestibility, microbial protein synthesis, and performance of lambs fed maize silage. J Agric Sci. 153:732–742.
- Van Soest PJ. 1994. Nutritional ecology of the ruminant., 2nd ed. Ithaca, NY, United States: Cornell Univ. Press.
- Van Soest PJ, Robertson JB, Lewis BA. 1991. Methods for dietary fibre, neutral detergent fibre, and non-starch carbohydrates in relation to animal nutrition. Int J Dairy Sci. 74:3583–3597.
- Wang SP, Wang WJ. 2016. Effects of dietary supplementation of Chinese herb medicine mixture on rumen fermentation, nutrient digestion, and blood profile in goats. S Afr J Anim Sci. 46:49–54.
- Yang, C., Gao, P., Hou, F., Yan, T., Chang, S., Chen, X., Wang, Z., 2018. Relationship between chemical composition of native forage and nutrient digestibility by Tibetan sheep on the qinghai–Tibetan plateau. J Anim Sci 96:1140–1149.