ABSTRACT
Mastitis is one of the most impacting diseases in dairy farming. Conventional treatment of mastitis using antibiotics is costly and has led to the emergence of antimicrobial resistance against most of the commonly used antibacterial agents. Research has begun to focus on molecules with antimicrobial potentials structurally different from conventional antibiotics We compared the antibacterial activity in vitro of ozonized sunflower oil (OSO) with different peroxide concentrations (150, 300, and 600 PI) against E. coli in goat, cow and sheep milk. It was found that the antibacterial effect, after 72 h, was more important for the goat's milk with OSO 150 (p < 0.001) and OSO 300 (p < 0.001). However, the effect was greater for cow's milk, when OSO 600 was used (p < 0.001). In the case of sheep's milk, it was observed that the antimicrobial effect was only significant with the use of OSO 600, however, this decrease in the concentration of E. coli (p < 0.001) remained practically unchanged from 24 h to 72 h of incubation. In conclusion, ozonated sunflower oil offers many therapeutic possibilities that would reduce the use of antibiotics for the prevention or treatments of mastitis, and its antimicrobial effect is greater with cow's milk and less with sheep's milk.
1. Introduction
Mastitis is one of the most impacting diseases in dairy farming. Their main cause is infections by bacteria and other microorganisms (Islas-Rodrìguez et al. Citation2009; Lakew et al. Citation2019) in addition to injuries and traumas in the udders (Megersa et al. Citation2010; Getaneh and Gebremedhin Citation2017). It is an important endemic disease that can affect all lactating animals (Ariffin et al. Citation2019). The most frequently found etiological agents in cows, goats and sheep suffering from infectious mastitis are staphylococci (S. aureus), streptococci (S. dysgalactiae, S. uberis) and coliform (Escherichia coli) (Tomasinsig et al. Citation2010; Ariffin et al. Citation2019; Giagu et al. Citation2022). It is estimated that approximately 10% of clinical coliform mastitis cause the death of animals (Hogan and Larry Smith Citation2003). The specie isolated in 80% of coliform cases is Escherichia coli (E. coli) (Bradley and Green Citation2001; Alekish et al. Citation2018). This microorganism usually infects the mammary glands during the dry period, progressing to inflammation and symptoms during early lactation (Balemi et al. Citation2021).
Controlling these infections is a long-sought ambition actually (Jingar et al. Citation2017). Conventional treatment of mastitis using antibiotics is costly and has led to the emergence of antimicrobial resistance (AMR) against most of the commonly used antibacterial agents (Bergonier et al. Citation2003; Jingar et al. Citation2017). The antibacterial sensitivity test results in different studies involving dairy cows, goats and sheep indicate an alarmingly increasing incidence of AMR among S. aureus and E. coli isolates against most commonly used antibacterial agents in many parts of the world (Vishnupriya et al. Citation2014; Jahan et al. Citation2015; Ismail Citation2017; Alekish et al. Citation2018) Therefore, not only AMR is regarded as a serious threat to global public health and food security, but it also increases animal suffering and production losses (Shinozuka et al. Citation2009; Alekish et al. Citation2018; de Prado-Taranilla et al. Citation2020). Therefore, research has begun to focus on drying off treatment protocols, vaccination and on molecules with antimicrobial and antiseptic potentials that are structurally different from conventional antibiotics (Whist et al. Citation2006; Lim et al. Citation2007; Sunder et al. Citation2013; Alekish et al. Citation2018; de Prado-Taranilla et al. Citation2020; Pollock et al. Citation2021; Rainard et al. Citation2022; Taga et al. Citation2012; H. Wang et al. Citation2022). One such molecule is ozone, which is the third most potent oxidant agent after fluor and persulfate and known for its antiseptic and anti-inflammatory properties. This molecule leads to the production of reactive oxygen species and the formation of hydrogen peroxide and lipid peroxidation products that are responsible for bacterial lysis and cell death (Grandi et al. Citation2022). Over the last few years, several therapeutic protocols using ozone have been developed to treat infections in human and animals. Thus, in the veterinary sector, ozone has been used due to its anti-inflammatory and antiseptic properties (Remondino and Valdenassi Citation2018). For example, it has been used in the prevention of epidemics from parasites in the poultry industry (da Soares et al. Citation2018), in urogenital tract infections (Koseman et al. Citation2019), in the purification of warehouses for animal feed (Conte et al. Citation2020; Kannan et al. Citation2021) in the disinfection of clinical equipment (Córdoba-Lanús et al. Citation2022; Torres-Mata et al. Citation2022); and in the prevention and treatment of mastitis also (Ogata and Nagahata Citation2000; Duričić et al. Citation2015). In these protocols, ozone is used in the following three different forms: gaseous ozone, ozonated water, and ozonated oil (Önyay et al. Citation2015; Fitzpatrick et al. Citation2018; Koseman et al. Citation2019; Grandi et al. Citation2022; Ramirez-Peña et al. Citation2022).
Ozonated vegetable oils are obtained after the oxidation generated by ozone to fatty acids and other substances present in vegetable oils. During this ozonation reaction, lipoperoxides, hydroperoxides, peroxides, ozonides, aldehydes and ketones are produced (Díaz et al. Citation2005) which, due to their high oxidant activity, affects polyunsaturated acids in the biological membranes of bacteria, molds, fungi, and viruses, while also oxidizing nucleic acids (Ayala et al. Citation2014; Torres-Mata et al. Citation2022; X. Wang et al. Citation2022) causing the destruction of the pathogenic agent. In recent years, ozonized sunflower oil (OSO) is one of the most used pharmaceutical presentations of ozone, which according to experimental and clinical studies, provides a large number of therapeutic effects against certain pathological agents including E. coli both in human and in veterinary medicine (Ginel et al. Citation2021; Grandi et al. Citation2022; Sechi et al. Citation2001; Zerillo et al. Citation2022).
Although there are several studies in which ozone therapy has been used for the treatment of mastitis (Ogata and Nagahata Citation2000; Ohtsuka et al. Citation2006; Shinozuka et al. Citation2009; Duričić et al. Citation2015; Koseman et al. Citation2019) some of them do not contain microbiological analysis; do not mention the concentration of ozone or time of the ozone treatment (Afonso et al. Citation2022). Taking into account that lipid oxidation products may exert antimicrobial and anti-inflammatory properties (Ogata and Nagahata Citation2000; Zamora Rodríguez et al. Citation2007; Skalska et al. Citation2009; Koseman et al. Citation2019) the aim of this study was to compare the antibacterial activity of OSO against E. coli on the three most common milk of animal origin in our geographical and socio-economic environment (goat, cow and sheep), evaluating in vitro, different peroxide concentrations and treatment times.
2. Materials and methods
2.1. Experimental design
Briefly in each type of milk (goat, G; cow, C; and sheep, S), the procedure was identical and consisted of evaluating the antimicrobial capacity against E. coli, of each concentration of OSO (PI 150, PI 300, PI 600) at different incubation times (0, 8, 12, 24, 48 and 72 h) at 37°C. Thus, each milk sample (990 μl) was inoculated with 1 μl of the E. coli EBETAN-1 suspension, as described in the corresponding section on material and methods. In addition, 10 μl of OSO was added to each sample, of the corresponding concentration according to the study group.
There is not much scientific bibliography regarding this matter, and aspects such as microbial analysis, dosage, volumes, or excipients of ozonized products, nor the action times, are usually not well explained or standardized. Thus, and calculating a volume of milk in the udder after milking of 200 mL for sheep and goats and about 400 mL for cows (Davis and Reinemann Citation2001; Cording et al. Citation2013; Meyer et al. Citation2022), we simulate the application of an intramammary cannula inside the udder of a cow, goat and sheep with a proportionally similar volume (1–2%) to that is used routinely in commercial presentations of intramammary cannulas for the treatment of mastitis in dairy cattle (Johns et al. Citation2001; Gonzalo et al. Citation2009; Bradley et al. Citation2010), avoiding the effect of dilution of the bacterial load that could be caused with larger volumes of product. Next, we decided to assess the effectiveness against a unique microorganism such as E. coli, which has been referred as one of the most common pathogens in mastitis in dairy cattle (Alekish et al. Citation2018; Ariffin et al. Citation2019; H. Wang et al. Citation2022). Finally, we focus on the study of antibacterial efficacy in the first 72 h.
As a summary, in each type of milk, the different concentrations of OSO were evaluated at six different times and each times was evaluated with eight samples. In summary, the total number of samples in the study was 576 (192 samples/ type of milk).
summarizes the experimental design.
Figure 1. Illustration of the experimental design used in this study and timeline. Goat, cow and sheep milk (G, C and S groups respectively) inoculated with E. coli EBETAN-1 HUGCDN. In each subgroup (n = 12), resulting from the combination of types of milk (G, C and S) with different types of ozonated sunflower oil (OSO150, OSO 300 and OSO 600) or not- ozonated sunflower oil (SO) the colony-forming units of E. coli EBETAN-1 HUGCDN per mL of milk (CFU/mL), at 0, 8, 12, 24, 48 and 72H incubation at 37°C were calculated.
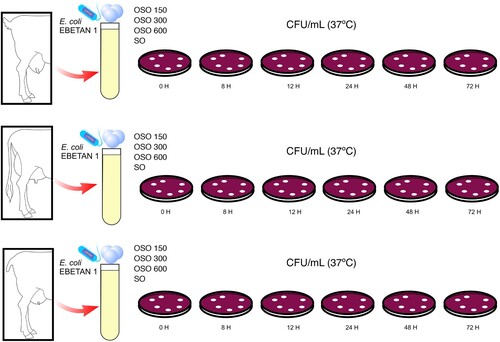
2.2. Preparation of milk
Goat and sheep milk were obtained from local farms. After milking they were kept at 4°C until use, while a commercial brand (Asturiana®) was used for the cow's milk group. At the beginning of each test, the milk was pasteurized in a water bath, guaranteeing 75°C, in the centre of the sample, for 15 min.
2.3. Preparation of ozonated sunflower oil (OSO)
Standardization of the preparation of OSO was carried out according to peroxide index (PI), which indicates the quantity of peroxide (active oxygen) available per kilogram of OSO (mmol/kg).
Ozonized sunflower oil (OSO) was commercially provided by Laboratorio Pérez del Toro (Gran Canaria, Spain) produced using a portable medical ozone generator (Ozonobaric P®, Sedecal, Madrid, Spain), and medical grade O2, obtaining three different concentrations of O3/sunflower oil: 150, 300 and 600 PI, (OSO 150, OSO 300 and OSO 600) equivalent to 150, 300 and 600 mmol/mL, respectively. Room temperature (22.0–23.7°C) and relative humidity (53–65%) were controlled by the air conditioning system of the Hospital de Gran Canaria Dr. Negrín. OSO was kept refrigerated at 4°C, and protected from light until used within 30 days of preparation. Non-ozonated sunflower oil (SO) was used as a control.
2.4. E. coli inocula preparation
The E. coli strain used in this study was isolated from intestinal microbiota of healthy Sprague Dawley rats from the animal facility of the Hospital Universitario de Gran Canaria, Dr. Negrín. The MALDI-TOF MS (Vitek®MS, Biomerieux) technique (Bizzini and Greub Citation2010) was used for the strain identification. The strain was designated as E. coli EBETAN-1 HUGCDN (Gutiérrez-Falcón et al. Citation2021). E. coli strain cultures were stored at −80°C with 20% glycerol (v/v) addition in Brain Heart Infusion broth (PanReac-AppliChem). Fresh cultures were made prior the assays, and the strain was aerobically incubated in Trypticase Soy broth (Becton Dickinson) medium for 18 h at 37°C with shaking (120 rpm). Before the challenge, the bacteria were centrifuged at 2500×g for 10 min and washed three times with sterile 0.9% saline solution. The bacterial concentration was measured with a spectrometer at 600 nm. Sterile 0.9% saline solution was used to adjust the suspension to the desired bacterial concentration (5 × 108 CFU/mL).
2.5. E. coli counts in milk
To determine the survival capability of E. coli EBETAN-1 HUGCDN in milk, the number of colony-forming units per mL of milk (CFU/mL) was measured. Milk samples were inoculated with the amount described in the experimental design section and the corresponding amount of OSO was added, according to the experimental group. To examine bacterial loads, serial tenfold dilutions were made in 0.9% sterile saline and plated on McConkey Agar (Beckton Dickinson). After incubation at 37°C for 0, 8, 12 24, 48 and 72 h, we calculated total viable counts of original samples (CFU/mL milk). Colonies were separated and isolated 2–3 times. We identified bacterial species using colony morphology and Gram stain. MALDI-TOF MS (Vitek®MS, Biomerieux) technique (Bizzini and Greub Citation2010)was used for advanced identification.
2.6. Statistical analysis
Analyses were done using Statistical Package R Core Team 2022 version 4.2 (R Foundation for Statistical Computing, Vienna, Austria). The Kolmogorov–Smirnov test has been used to check the normality of the data. Quantitative variables are expressed as medians and 25th and 75th percentiles. To calculate the differences between incubation times and types of milk with OSO, the linear model of repeated measures with interaction has been used. Data are expressed as median. All tests were two-tailed and statistical significance was considered at p < 0.05.
3. Results
The number of colony-forming units of E. coli EBETAN-1 HUGCDN per mL of milk, according to type of animal species, type of OSO and incubation time are shown in .
Table 1. Minimum (Min), maximum (Max), 25th, 50th and 75th percentiles (P25, P50 and P75, respectively) of the number of colony-forming units of E. coli EBETAN-1 HUGCDN per mL of milk (CFU/mL) during 0, 8, 12, 24, 48 and 72 h of incubation (0H, 8H, 12H, 24H, 48H and 72H respectively).
3.1. Antimicrobial effect depending on the type of OSO
The concentrations of colony-forming units over time, depending on the type of OSO used, are shown in . The first result that we can highlight is that the concentration of E. coli EBETAN-1 HUGCDN continues to increase in cow's milk, reaches the plateau of the curve in sheep's milk, and decreases in goat's milk after 72 h of incubation at 37°C.
Figure 2. E. coli EBETAN-1 HUGCDN concentration (log10 CFU/mL) in cow's milk, goat's milk and sheep's milk containing non-ozonated sunflower oil (SO) during the first 72 h of incubation at 37°C. Data shown as median. Significant difference ***(p < 0.001).
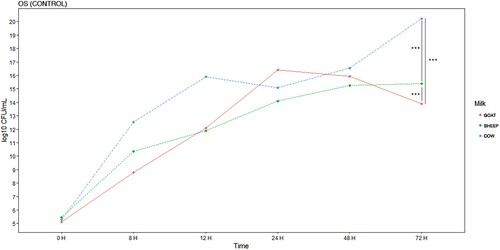
Figure 3. E. coli EBETAN-1 HUGCDN concentration (log10 CFU/mL) in cow's milk, goat's milk and sheep's milk containing ozonated sunflower oil 150 PI (OSO 150) during the first 72 h of incubation at 37°C. Data shown as median. Significant difference **(0.001 > p < 0.01) and ***(p < 0.001).
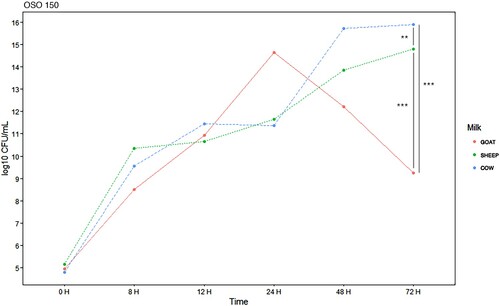
Figure 4. E. coli EBETAN-1 HUGCDN concentration (log10 CFU/mL) in cow's milk, goat's milk and sheep's milk containing ozonated sunflower oil 300 PI (OSO 300) during the first 72 h of incubation at 37°C. Data shown as median. Significant difference **(0.001 > p < 0.01) and ***(p < 0.001).
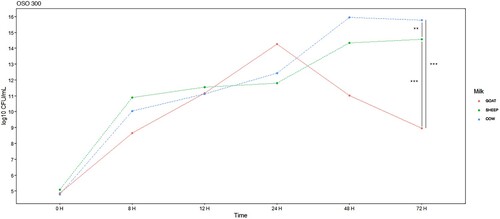
Figure 5. E. coli EBETAN-1 HUGCDN concentration (log10 CFU/mL) in cow's milk, goat's milk and sheep's milk containing ozonated sunflower oil 600 PI (OSO 600) during the first 72 h of incubation at 37°C. Data shown as median. Significant difference ***(p < 0.001).
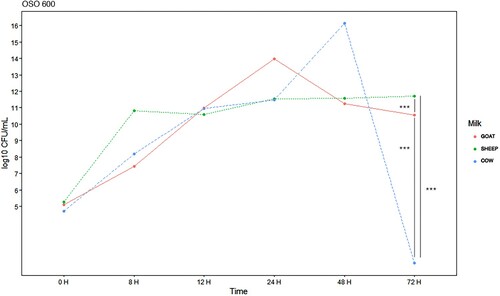
Comparing with the normal evolution of the bacterial load in the milk samples treated with SO, it was found that the antibacterial effect, on the studied strain, after 72 h of incubation, is more important for the goat milk with OSO 150 (14.3 vs 9.08 log10 CFU/mL, p < 0.001) and OSO 300 (14.3 vs 9 log10 CFU/mL, p < 0.001). However, the effect was greater for cow's milk, when OSO 600 was used (20.67 vs 2.3 log10 CFU/mL, p < 0.001).
3.2. Antimicrobial effect depending on the animal origin of the milk
The concentrations of colony-forming units over time, depending on the type of milk used, are shown in . When we analysed the values in cow's milk, it was observed that the greatest difference appeared at 72 h, when using OSO 600, the bacterial population significantly decreased to concentration below 3 log10 CFU/mL (p < 0.05), while the groups with OSO 150 and OSO 300, maintained similar values (15.76 and 15.75 log10 CFU/mL respectively).
Figure 6. E. coli EBETAN-1 HUGCDN reduction (log10 CFU/mL) of cow's milk containing non-ozonated sunflower oil (SO), and ozonated sunflower oil with three different concentrations of peroxides (OSO 150, OSO 300 and OSO 600) during the first 72 h of incubation at 37°C. Data shown as median. Significant difference *(p < 0.05), and **(0.001 > p < 0.01).
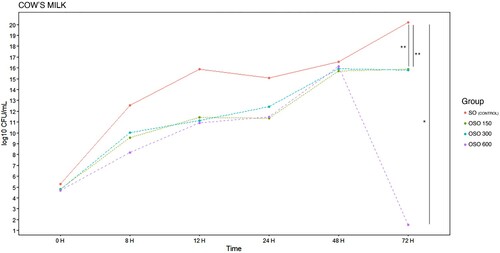
Figure 7. E. coli EBETAN-1 HUGCDN reduction (log10 CFU/mL) of goat's milk containing non-ozonated sunflower oil (SO), and ozonated sunflower oil with three different concentrations of peroxides (OSO 150, OSO 300 and OSO 600) during the first 72 h of incubation at 37°C. Data shown as median. Significant difference **(0.001 > p < 0.01).
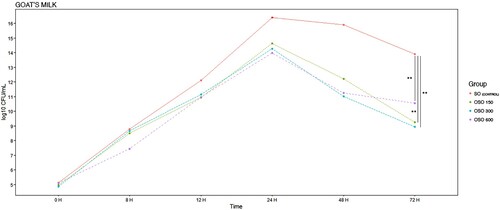
Figure 8. E. coli EBETAN-1 HUGCDN reduction (log10 CFU/mL) of sheep's milk containing non-ozonated sunflower oil (SO), and ozonated sunflower oil with three different concentrations of peroxides (OSO 150, OSO 300 and OSO 600) during the first 72 h of incubation at 37°C. Data shown as median. Significant difference **(0.001 > p < 0.01).
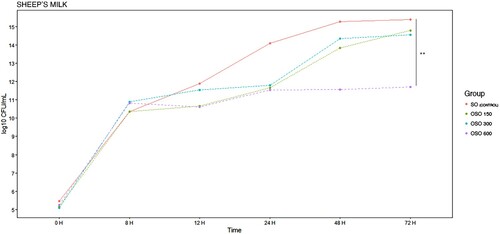
In the case of goat milk, decreases in bacterial concentration began to be noted after 24 h, regardless of the type of OSO used. We observed the greatest effect at 72 h with the use of OSO 150 or OSO 300 in a very similar way.
Finally, in the case of sheep's milk, it was observed that the antimicrobial effect was only significant with the use of OSO 600, however, this decrease in the concentration of E. coli EBETAN-1 HUGCDN (0.001 > p < 0.01) remained practically unchanged from 24 h to 72 h of milk incubation (11.62–11.74 log10 CFU/mL).
4. Discussion
In general, when the antimicrobial capacity of the different concentrations of OSO on the E. coli strain used was evaluated, we could to observe a clear germicidal effect dependent on the concentration of OSO and on the time of action. Several authors have demonstrated the efficacy of ozone's oxidative potential on bacteria or fungi, such as Staphylococcus aureus (Epelle et al. Citation2022), Klebsiella pneumoniae (Piletić et al. Citation2022), E. coli and Pseudomonas aeruginosa (Baghal Asghari et al. Citation2021), Aspergillus fumigatus, and Candida albicans (Epelle et al. Citation2022). In addition, other authors have indicated that Gram-negative bacteria, such as E. coli, are generally more sensitive to ozone than Gram-positive bacteria due to the lower amount of peptidoglycan in their cell walls and its protective role against peroxidation of the cell membrane caused by reactive oxygen species (Rangel et al. Citation2022).
To the best of our knowledge, no studies in the literature investigate the time required for action of ozone or ozone-containing preparations to achieve an optimal antimicrobial effect in milk. There are studies in cows, in which it is shown that the intramammary application of foam at two different moments of the lactation period, offers better results on the concentration of E. coli, and somatic cell counting than if it is applied only once (Koseman et al. Citation2019). These results partially agree with ours, since the best antimicrobial effects were obtained with the longer OSO action times. However, we must be careful in our conclusions since the ozone-containing foam preparation used by these authors contained a mixture of ozone, olive oil, glycerin, propylene glycol and various caring oils. Ozone has a half-life of approximately 20 min in the gaseous phase, which has restricted some applications before low-concentration exposures for prolonged periods, with limited effectiveness (Rangel et al. Citation2022; Torres-Mata et al. Citation2022). For this reason, we believe that the application of ozone in the form of ozonated oil represents a therapeutic advantage and would explain that after 72 h of being added to milk, the antimicrobial effect was maintained.
In a study which the capacity of ozone against bacteria such as S. aureus, E. coli and Bacillus stearothermophilus was evaluated in different types of substrate (sterile buffer solution, whipping cream, locust bean gum solution, starch solution soluble and sodium caseinate solution) the authors concluded that the cream and sodium caseinate solution promoted a greater protective effect of the bacteria, hindering the action of ozone (Güzel-Seydim et al. Citation2004). Furthermore, the amount of fat can also interfere with the solubility of this gas in liquid media. In a study carried out with many different samples of milk exposed to ozone in different presentations, the best results in reducing the microorganism count, were obtained from samples that contained low levels of fat (Afonso et al. Citation2022). In another study whose object of analysis was orange juice, it was verified that organic matter exerts a negative effect on the oxidative capacity of ozone on the inoculated bacteria, in this case, E. coli (Patil et al. Citation2009). Our results could be explained by this fact, since the highest concentrations of bacteria at 72 h were in sheep's milk, followed by goat's milk and finally cow's milk, which corresponds to the order of higher to lower organic matter (Moatsou and Park Citation2017). Therefore, the amount of organic matter found in sheep's milk could affect the bactericidal capacity of OSO, giving significant results only in the group in which the highest concentration was used (OSO 600).
On the other hand, we must not forget that ozone is a powerful oxidizing agent with a potential toxic effect on animal cells. In this way, there are several studies that warn about this characteristic (Córdoba-Lanús et al. Citation2022; Rangel et al. Citation2022), even finding in vivo studies on infectious models that show that rectal pre-treatment with ozonized oxygen aggravates clinic status in septic rats treated with amoxicillin/clavulanate (Martín-Barrasa et al. Citation2015).
To conclude, we must indicate that the greatest strength of this study is that it is a work with a powerful statistical evaluation of three types of milk with different standardized ozone concentrations, using an excipient widely used in the formulation of commercial presentations of intramammary drugs, and its effectiveness is evaluated in defined and controlled times.
Although the use of ozonated sunflower oil offers many therapeutic possibilities that would reduce the use of antibiotics for the prevention or treatments of mastitis, new studies are necessary to evaluate the effect of this gas on other common pathogens in mastitis. In addition, in vitro tests on bactericidal effects or glandular toxicity must be complemented with in vivo studies that provide certainty about the use of suboptimal, therapeutic or excessive doses of this powerful antiseptic, and its impact on milk production, toxicity, residues in milk or meat and/or organoleptic alterations in foods of animal origin.
Acknowledgements
The authors would like to thank Mr. Juan Ramirez Verona from the Illustration and Iconography Service at Hospital Universitario de Gran Canaria Dr. Negrín, and Mr. Miguel Martín Betancor for their technical assistance during the preparation and design of figures and tables. The authors also thank Laboratorio Pérez del Toro (Gran Canaria, Spain) that provided the ozonated oil at different concentrations.
Disclosure statement
No potential conflict of interest was reported by the author(s).
Additional information
Funding
References
- Afonso RB, Moreira RHR, de Almeida PLR. 2022. Can ozone be used as antimicrobial in the dairy industry? A systematic review. J Dairy Sci. 105(2):1051–1057. doi:10.3168/jds.2021-20900.
- Alekish M, Ismail ZB, Albiss B, Nawasrah S. 2018. In vitro antibacterial effects of zinc oxide nanoparticles on multiple drug-resistant strains of Staphylococcus aureus and Escherichia coli: an alternative approach for antibacterial therapy of mastitis in sheep. Vet World. 11(10):1428–1432. doi:10.14202/vetworld.2018.1428-1432.
- Ariffin SM, Hasmadi N, Syawari NM, Sukiman MZ, Ariffin MFT, Hian CM, Ghazali MF. 2019. Prevalence and antibiotic susceptibility pattern of Staphylococcus aureus, Streptococcus agalactiae and Escherichia coli in dairy goats with clinical and subclinical mastitis. J Anim Health Prod. 7(1):32–37. doi:10.17582/journal.jahp/2019/7.1.32.37.
- Ayala A, Muñoz MF, Argüelles S. 2014. Lipid peroxidation: production, metabolism, and signaling mechanisms of malondialdehyde and 4-hydroxy-2-nonenal. Oxid Med Cell Longevity. 2014, 1–31. doi:10.1155/2014/360438. Landes Bioscience.
- Baghal Asghari F, Dehghani MH, Dehghanzadeh R, Farajzadeh D, Shanehbandi D, Mahvi AH, Yaghmaeian K, Rajabi A. 2021. Performance evaluation of ozonation for removal of antibiotic-resistant Escherichia coli and Pseudomonas aeruginosa and genes from hospital wastewater. Sci Rep. 11:1. doi:10.1038/s41598-021-04254-z.
- Balemi A, Gumi B, Amenu K, Girma S, Gebru M, Tekle M, Rius AA, D’souza DH, Agga GE, Dego OK. 2021. Prevalence of mastitis and antibiotic resistance of bacterial isolates from cmt positive milk samples obtained from dairy cows, camels, and goats in two pastoral districts in southern Ethiopia. Animals (Basel). 11:1530. doi:10.3390/ani11061530.
- Bergonier D, de Crémoux R, Rupp R, Lagriffoul G, Berthelot X. 2003. Mastitis of dairy small ruminants. Vet Res. 34(5):689–716. doi:10.1051/vetres:2003030.
- Bizzini A, Greub G. 2010. Matrix-assisted laser desorption ionization time-of-flight mass spectrometry, a revolution in clinical microbial identification. Clin Microbiol Infect. 16(11):1614–1619. doi:10.1111/j.1469-0691.2010.03311.x.
- Bradley AJ, Breen JE, Payne B, Williams P, Green MJ. 2010. The use of a cephalonium containing dry cow therapy and an internal teat sealant, both alone and in combination. J Dairy Sci. 93(4):1566–1577. doi:10.3168/jds.2009-2725.
- Bradley AJ, Green MJ. 2001. An investigation of the impact of intramammary antibiotic dry cow therapy on clinical coliform mastitis. J Dairy Sci. 84(7):1632–1639. doi:10.3168/jds.S0022-0302(01)74598-5.
- Conte G, Fontanelli M, Galli F, Cotrozzi L, Pagni L, Pellegrini E. 2020. Mycotoxins in feed and food and the role of ozone in their detoxification and degradation: an update. Toxins (Basel). 12(8), 486. doi:10.3390/toxins12080486. MDPI AG.
- Cording F, Hoedemaker M, Krömker V. 2013. Relationship between residual milk and clinical mastitis in dairy cattle. Tierarztl Prax Ausg G Grosstiere Nutztiere. 41(6):379–386.
- Córdoba-Lanús E, García-Pérez O, Rodríguez-Esparragón F, Bethencourt-Estrella CJ, Torres-Mata LB, Blanco A, Villar J, Sanz O, Díaz JJ, Martín-Barrasa JL, et al. 2022. Ozone treatment effectively eliminates SARS-CoV-2 from infected face masks. PLoS One. 17(7):e0271826. doi:10.1371/journal.pone.0271826.
- da Soares CES, Weber A, Moecke EHS, de Souza CK, Reiter MGR, Scussel VM. 2018. Use of ozone gas as a green control alternative to beetles Alphitobius diaperinus (panzer) infestation in aviary bed utilized in the poultry industry. Chem Eng Trans. 64:589–594. doi:10.3303/CET1864099.
- Davis MA, Reinemann DJ. 2001. Methodology of measuring strip yield. Proc 2nd Int Symp Mastit Milk Qual; Sep 12–14; Vancouver, British Columbia. pp. 13–15.
- de Prado-Taranilla AI, Holstege MMC, Bertocchi L, Appiani A, Becvar O, Davidek J, Bay D, Jimenez LM, Roger N, Krömker V, et al. 2020. Incidence of milk leakage after dry-off in European dairy herds, related risk factors, and its role in new intramammary infections. J Dairy Sci. 103(10):9224–9237. doi:10.3168/jds.2019-18082.
- Díaz MF, Gavín Sazatornil JA, Ledea O, Hernández F, Alaiz M, Garcés R. 2005. Spectroscopic characterization of ozonated sunflower oil. Ozone: Sci Eng. 27(3):247–253. doi:10.1080/01919510590945822.
- Duričić D, Valpotić H, Samardžija M. 2015. Prophylaxis and therapeutic potential of ozone in Buiatrics: current knowledge. Anim Reprod Sci. 159:1–7. doi:10.1016/j.anireprosci.2015.05.017. Elsevier B.V.
- Epelle EI, Macfarlane A, Cusack M, Burns A, Thissera B, Mackay W, Rateb ME, Yaseen M. 2022. Bacterial and fungal disinfection via ozonation in air. J Microbiol Methods. 194, 106431. doi:10.1016/j.mimet.2022.106431
- Fitzpatrick E, Holland OJ, Vanderlelie JJ. 2018. Ozone therapy for the treatment of chronic wounds: a systematic review. Int Wound J. 15(4):633–644. doi:10.1111/iwj.12907.
- Getaneh AM, Gebremedhin EZ. 2017. Meta-analysis of the prevalence of mastitis and associated risk factors in dairy cattle in Ethiopia. Trop Anim Health Prod. 49(4):697–705. doi:10.1007/s11250-017-1246-3.
- Giagu A, Penati M, Traini S, Dore S, Addis MF. 2022. Milk proteins as mastitis markers in dairy ruminants - a systematic review. Vet Res Commun. 46(2):329–351. doi:10.1007/s11259-022-09901-y.
- Ginel PJ, Negrini J, Guerra R, Lucena R, Ruiz-Campillo MT, Mozos E. 2021. Effect of topical ozonated sunflower oil on second intention wound healing in turtles: a randomised experimental study. J Vet Sci. 22:1–13. doi:10.4142/jvs.2021.22.e27.
- Gonzalo C, Linage B, Carriedo JA, Juárez MT, Beneitez E, Martínez A, de La Fuente LF. 2009. Short communication: effect of dry therapy using an intramammary infusion on bulk tank somatic cell count in sheep. J Dairy Sci. 92(1):156–159. doi:10.3168/jds.2008-1403.
- Grandi G, Cavallo R, Zanotto E, Cipriani R, Panico C, Protti R, Scapagnini G, Davinelli S, Costagliola C. 2022. In vitro antimicrobial activity of ozonated oil in liposome eyedrop against multidrug-resistant bacteria. Open Med. 17(1):1057–1063. doi:10.1515/med-2022-0495.
- Gutiérrez-Falcón AI, Ramos-Nuez AM, de los Monteros y Zayas AE, Castillo DFP, García-Laorden MI, Chamizo-López FJ, Real Valcárcel F, Campelo FA, Benítez AB, Salgueiro PN, et al. 2021. Probiotic properties of Alcaligenes faecalis isolated from Argyrosomus regius in experimental peritonitis (Rat model). Probiotics Antimicrob Proteins. 13(5):1326–1337. doi:10.1007/s12602-021-09767-7.
- Güzel-Seydim Z, Bever PI, Greene AK. 2004. Efficacy of ozone to reduce bacterial populations in the presence of food components. Food Microbiol. 21(4):475–479. doi:10.1016/j.fm.2003.10.001.
- Hogan J, Larry Smith K. 2003. Coliform mastitis. Vet Res. 34(5):507–519. doi:10.1051/vetres:2003022.
- Islas-Rodrìguez AE, Marcellini L, Orioni B, Barra D, Stella L, Mangoni ML. 2009. Esculentin 1-21: a linear antimicrobial peptide from frog skin with inhibitory effect on bovine mastitis-causing bacteria. J Pept Sci. 15(9):607–614. doi:10.1002/psc.1148.
- Ismail ZB. 2017. Molecular characteristics, antibiogram and prevalence of multi-drug resistant Staphylococcus aureus (MDRSA) isolated from milk obtained from culled dairy cows and from cows with acute clinical mastitis. Asian Pac J Trop Biomed. 7(8):694–697. doi:10.1016/j.apjtb.2017.07.005.
- Jahan M, Rahman M, Parvej MS, Chowdhury SMZH, Haque ME, Talukder MAK, Ahmed S. 2015. Isolation and characterization of Staphylococcus aureus from raw cow milk in Bangladesh. J Adv Vet Anim Res. 2(1):49–55. doi:10.5455/javar.2015.b47.
- Jingar SC, Singh M, Roy AK. 2017. Economic losses due to clinical mastitis in cross-bred cows. Dairy and Vet Sci J. 3:2. doi:10.19080/JDVS.2017.03.555606.
- Johns PJ, Turberg M, Willard K, Matagne D, Cheesman D. 2001. Stability of a second-generation cephalosporin veterinary mastitis formulation after electron beam irradiation. AAPS PharmSciTech. 2(4):28–34. http://www.pharmscitech.com.
- Kannan G, Mahapatra AK, Degala HL. 2021. Preharvest management and postharvest intervention strategies to reduce Escherichia coli contamination in goat meat: a review. Animals (Basel) 11(10), 2943. doi:10.3390/ani11102943. MDPI.
- Koseman A, Seker I, Risvanli A. 2019. Influence of intra-mammary ozone administration on udder health in herds with contagious mastitis in the context of management practices. Pol J Vet Sci. 22(4):703–710. doi:10.24425/pjvs.2019.129983.
- Lakew BT, Fayera T, Ali YM. 2019. Risk factors for bovine mastitis with the isolation and identification of Streptococcus agalactiae from farms in and around Haramaya district, eastern Ethiopia. Trop Anim Health Prod. 51(6):1507–1513. doi:10.1007/s11250-019-01838-w.
- Lim GH, Kelton DF, Leslie KE, Timms LL, Church C, Dingwell RT. 2007. Herd management factors that affect duration and variation of adherence of an external teat sealant. J Dairy Sci. 90(3):1301–1309. doi:10.3168/jds.S0022-0302(07)71618-1.
- Martín-Barrasa JL, Cordovez CM, de Los Monteros Y Zayas AE, de Santa Ana MCJ, Varas BC, Thomas PH, Benitez AB, Montoya-Alonso JA, García-Bello M, Campelo FA, Tejedor-Junco MT. 2015. Rectal pre-treatment with ozonized oxygen (O3) aggravates clinic status in septic rats treated with amoxicillin/clavulanate. Enfermedades Infecciosas y Microbiología Clínica. 33(7):469–475. doi:10.1016/j.eimc.2014.09.006.
- Megersa B, Tadesse C, Abunna F, Regassa A, Mekibib B, Debela E. 2010. Occurrence of mastitis and associated risk factors in lactating goats under pastoral management in Borana, Southern Ethiopia. Trop Anim Health Prod. 42(6):1249–1255. doi:10.1007/s11250-010-9557-7.
- Meyer D, Haeussermann A, Hartung E. 2022. Amount and variation of strip yields collected by a defined hand-milking method after machine milking of Holstein dairy cows. Animal. 16(5):100508. doi:10.1016/j.animal.2022.100508.
- Moatsou G, Park YW. 2017. Goat milk products: types of products, manufacturing technology, chemical composition, and marketing. In: Young W. Park, D. Min, George F. W. Haenlein, William L. Wendorff, editors. Handb milk non-bovine mamm. Hoboken (NJ): John Wiley & Sons, Ltd; p. 84–150. doi:10.1002/9781119110316.ch2.3.
- Ogata A, Nagahata H. 2000. Intramammary application of ozone therapy to acute clinical mastitis in dairy cows. J Vet Med Sci. 62(7):681–686.
- Ohtsuka H, Ogata A, Terasaki N, Koiwa M, Kawamura S. 2006. Changes in leukocyte population after ozonated autohemoadministration in cows with inflammatory diseases. J Vet Med Sci. 68(2):175–178 . doi:10.1292/jvms.68.175.
- Önyay F, Küçükaslan İ, Ay SS, Findik M. 2015. Ozone treatment in veterinary gynecology and obstetrics: review. Turkiye Klinikleri J Vet Sci. 6(2):48–53. doi:10.5336/vetsci.2015-47260.
- Patil S, Bourke P, Frias JM, Tiwari BK, Cullen PJ. 2009. Inactivation of Escherichia coli in orange juice using ozone. Inno Food Sci Emerg Technol. 10(4):551–557. doi:10.1016/j.ifset.2009.05.011.
- Piletić K, Kovač B, Perčić M, Žigon J, Broznić D, Karleuša L, Lučić Blagojević S, Oder M, Gobin I. 2022. Disinfecting action of gaseous ozone on OXA-48-producing Klebsiella pneumoniae biofilm in vitro. Int J Environ Res Public Health. 19(10):6177. doi:10.3390/ijerph19106177.
- Pollock J, Salter SJ, Nixon R, Hutchings MR. 2021. Milk microbiome in dairy cattle and the challenges of low microbial biomass and exogenous contamination. Anim Microbiome. 3:1. doi:10.1186/s42523-021-00144-x.
- Rainard P, Foucras G, Martins RP. 2022. Adaptive cell-mediated immunity in the mammary gland of dairy ruminants. Front Vet Sci. 9. doi:10.3389/fvets.2022.854890.
- Ramirez-Peña AM, Sánchez-Pérez A, Campos-Aranda M, Hidalgo-Tallón FJ. 2022. Ozone in patients with periodontitis: a clinical and microbiological study. J Clin Med. 11(10):2946. doi:10.3390/jcm11102946.
- Rangel K, Cabral FO, Lechuga GC, Carvalh JPRS, Villas-Bôas MHS, Midlej V, De-Simone SG. 2022. Detrimental effect of ozone on pathogenic bacteria. Microorganisms. 10:40. doi:10.3390/microorganisms10010040.
- Remondino M, Valdenassi L. 2018. Different uses of ozone: environmental and corporate sustainability. literature review and case study. Sustainability. 10(12):4783. doi:10.3390/su10124783.
- Sechi LA, Lezcano I, Nunez N, Espim M, Dupre Á I, Pinna A, Molicotti P, Fadda G, Zanetti S. 2001. Antibacterial activity of ozonized sunflower oil (Oleozon). J Appl Microbiol. 90(2):279–284. doi:10.1046/j.1365-2672.2001.01235.x.
- Shinozuka Y, Hirata H, Ishibashi I, Okawa Y, Kasuga A, Takagi M, Taura Y. 2009. Therapeutic efficacy of mammary irrigation regimen in dairy cattle diagnosed with acute coliform mastitis. J Vet Med Sci. 71(3):269–273.
- Skalska K, Ledakowicz S, Perkowski J, Sencio B. 2009. Germicidal properties of ozonated sunflower oil. Ozone: Sci Eng. 31(3):232–237. doi:10.1080/01919510902838669.
- Sunder J, De AK, Jeyakumar S, Kundu A. 2013. Effect of feeding of Morinda citrifolia fruit juice on the biophysical parameters of healthy as well as mastitis-affected cow milk. J Appl Anim Res. 41(1):29–33. doi:10.1080/09712119.2012.738212.
- Taga I, Lan CQ, Altosaar I. 2012. Plant essential oils and mastitis disease: their potential inhibitory effects on pro-inflammatory cytokine production in response to bacteria related inflammation.. Nat Prod Commun. 7(5):675–682. PMID: 22799106.
- Tomasinsig L, de Conti G, Skerlavaj B, Piccinini R, Mazzilli M, D’Este F, Tossi A, Zanetti M. 2010. Broad-spectrum activity against bacterial mastitis pathogens and activation of mammary epithelial cells support a protective role of neutrophil cathelicidins in bovine mastitis. Infect Immun. 78(4):1781–1788. doi:10.1128/IAI.01090-09.
- Torres-Mata LB, García-Pérez O, Rodríguez-Esparragón F, Blanco A, Villar J, Ruiz-Apodaca F, Martín-Barrasa JL, González-Martín JM, Serrano-Aguilar P, Piñero JE, et al. 2022. Ozone eliminates SARS-CoV-2 from difficult-to-clean office supplies and clinical equipment. Int J Environ Res Public Health. 19:8672. doi:10.3390/ijerph19148672.
- Vishnupriya S, Antony PX, Mukhopadhyay HK, Pillai RM, Thanislass J, Vivek Srinivas VM, Sumanth Kumar R. 2014. Methicillin resistant staphylococci associated with bovine mastitis and their zoonotic importance. Veterinary World. 7(6):422–427. doi:10.14202/vetworld.2014.422-427.
- Wang H, Yuan L, Wang T, Cao L, Liu F, Song J, Zhang Y. 2022. Construction of the waaF subunit and DNA vaccine against Escherichia coli in cow mastitis and preliminary study on their immunogenicity. Front Vet Sci. 9:877685. doi:10.3389/fvets.2022.877685.
- Wang X, Liao D, Ji QM, Yang YH, Li MC, Yi XY, Li C, Chen Y, Tao HB, Zhai WH. 2022. Analysis of bactericidal effect of three medical ozonation dosage forms on multidrug-resistant bacteria from burn patients. Infect Drug Resist. 15:1637–1643. doi:10.2147/IDR.S353277.
- Whist AC, Østerås O, Sølverød L. 2006. Clinical mastitis in Norwegian herds after a combined selective dry-cow therapy and teat-dipping trial. J Dairy Sci. 89(12):4649–4659. doi:10.3168/jds.S0022-0302(06)72515-2.
- Zamora Rodríguez ZB, González Álvarez R, Guanche D, Merino N, Hernández Rosales F, Menéndez Cepero S, Alonso González Y, Schulz S. 2007. Antioxidant mechanism is involved in the gastroprotective effects of ozonized sunflower oil in ethanol-induced ulcers in rats. Mediat Inflamm. 2007:1–6. doi:10.1155/2007/65873.
- Zerillo L, Polvere I, Varricchio R, Madera JR, D’Andrea S, Voccola S, Franchini I, Stilo R, Vito P, Zotti T. 2022. Antibiofilm and repair activity of ozonated oil in liposome. Microb Biotechnol. 15(5):1422–1433. https://doi.org/10.1111/1751-7915.13949.