ABSTRACT
Shanbei White Cashmere goat is a typical goat species, the growth of cashmere is affected by photoperiod. To further investigate the effects of short photoperiod treatment on cashmere growth, hormone concentrations and hair follicle development-related gene expression in cashmere goats, twenty Shannbei white cashmere goats were selected and randomly divided into long-day photoperiod treatment group and short-day photoperiod treatment group. The results revealed the cashmere on short-day photoperiod treatment began to develop earlier, and its length and growth rate increased dramatically (P < 0.05). The short-day photoperiod treatment increased the concentration of serum Melatonin, reduced the concentration of serum prolactin, decreased the expression of estrogen receptor alpha and raised the expression of hair follicle development-related genes [catenin beta-1, Bone morphogenetic protein 2, Fibroblast growth factor 5 and platelet-derived growth factor subunit A] (P < 0.05), thereby accelerating the reconstruction of the secondary follicle, increasing the density of the secondary follicle (P < 0.05) and triggering the growth of cashmere goats. The present study provides new insights into the dynamic changes in cashmere growth, hormone concentrations and hair follicle development-related gene expression in cashmere goats under short photoperiod, and is expected to be useful for future studies on intensive feeding research.
Introduction
Cashmere goats have primary hair follicles (PHFs) and secondary hair follicles (SHFs), which are two different types of skin follicles (Zhang et al. Citation2020). SHFs evolved into cashmere, while PHFs produced coarse hair (Ansari-Renani et al. Citation2011). Cashmere is a crucial raw material for the textile industry in the world with a substantial economic value (Liu et al. Citation2012).
The SHFs of cashmere goats are clearly periodic, requiring passage through the anagen, catagen and telogen phases (Rile et al. Citation2018). The periodicity of SHFs in cashmere goats is directly to the photoperiod. The reduction of daylight encouraged the development and manufacturing of cashmere (Zhang et al. Citation2019).
Changes in photoperiod influence the growth of cashmere by modulating hormones. The light causes the pineal gland to secrete melatonin (MT) by transmitting nerve impulses through the retina to the superior chiasmal nucleus, then the paraventricular nucleus, and lastly the pineal gland (Cassone Citation1990). MT is essential to the growth of cashmere. Welch found that injecting MT to goats increased cashmere production in September (Welch et al. Citation1990). Implantation of melatonin from July to September improved cashmere production (Klören and Norton Citation1995). MT implantation significantly prolongs the growth phase of cashmere in the winter solstice (Cong et al. Citation2011). Recent research indicates that melatonin stimulated Cashmere growth by boosting antioxidant levels (Yang et al. Citation2019). Studies have pointed out that thyroid hormones and 17β-estradiol (E2) also are involved with the growth of cashmere (Rhind and McMillen Citation1995; Movérare et al. Citation2002). These hormones interact with each other. MT induces the secretion of prolactin, which causes hair follicles to enter the anagen phase (Nixon et al. Citation1993; Foitzik et al. Citation2006; Plikus et al. Citation2008). The transformation of anagen, catagen, and telogen phases were regulated by some specific pathways (Millar Citation2002; Blanpain and Fuchs Citation2006). The Wnt/β-catenin signalling pathway is the primary regulator of epidermal and mesenchymal cell differentiation (Andl et al. Citation2002). β-catenin (catenin beta-1) is a crucial downstream component of the canonical Wnt signalling pathway that binds to insulin-like growth factor 1 (IGF-1) to create transcription factors that regulate the growth and development of hair follicles in animals. Bone morphogenetic protein (BMP) is a growth and differentiation agent that stimulates bone production (Myllylä R et al. Citation2014). The ratio of BMP to β-catenin activity controls the direction of hair follicle stem cell development (Plikus et al. Citation2008; Kandyba et al. Citation2013). Bone morphogenetic protein 2 (BMP2) and bone morphogenetic protein 4 (BMP4) are the two most biologically active genes in the BMP family, which primarily influence the periodic growth and development of hair follicles (Bai W et al. Citation2016). Platelet-derived growth factor subunit A (PDGFA) is expressed in epidermal and follicular epithelial cells, promotes the formation of dermal papilla, stromal sheath, and dermal fibroblasts, IGF-1 treatment can up-regulate the expression of PDGFA, inhibit apoptosis, promote hair follicle growth, and maintain hair follicles in the anagen phase (Ahn et al. Citation2012). However, the pattern of hair regeneration varies among different species (Plikus et al. Citation2011), with the majority of these studies focusing on humans and other animals. There are few studies relating the short-day photoperiod treatment to the cashmere growth of goats, particularly the Shanbei White Cashmere goat. Therefore, it is necessary to evaluate the association between the short-day photoperiod treatment and cashmere growth in cashmere goats. In this context, We hypothesized that the short-day photoperiod treatment could affect the Shanbei White Cashmere goat’s cashmere development, and the aim of this study was to compare the cashmere growth, hormone concentrations and hair follicle development-related gene expression of cashmere goats under short-day photoperiod and natural conditions, and to determine the effect of short-day light on the cashmere growth of the Shanbei White Cashmere goat by comparing these differences. This research will be of great use for farming cashmere goat in places with short photoperiods and enhancing cashmere production through suitable light regulation.
Materials and methods
Animals
This study was conducted from 15 May 2020 to 15 May 2021 at the Shaanbei White Cashmere Goat Farm in Yulin, Shaanxi province, China (latitude N37°38′, longitude E109°12′; altitude: 1498 m). Randomly selected 20 empty pregnant ewes from 130 healthy Shaanbei white cashmere goats aged 2.5 years old, similar in weight and body condition, and divided evenly between the treatment group and the control group. The use of animals and all experimental protocols (protocol number: 100403) were authorized by the Institutional Animal Care and Use Committee of Northwest A&F University (Yangling, Shaanxi, China, Approval ID: 2014ZX08008-002).
Photoperiodic manipulations
After a two-week acclimation period on a long-day photoperiod, ten female Shanbei White Cashmere goats (2.5 years old with an initial body weight of 34.12 ± 1.54 kg) were placed into short-day photoperiod treatment conditions (Receive light from 9:30 am to 16:30 pm, 7 h of light per day) as the treatment group, and they were housed in a dark environment for the remainder of the time. The illumination was controlled at approximately 0.1 lux (Illuminance in the dark) by closing the doors and using shade cloth to avoid sunlight. As the control group, 10 additional goats were housed in an identical shed with long-day photoperiod (Natural photoperiod, 12–13.5 h of light per day) conditions. All goats were fed and allowed to drink from 9:30 am to 16:30 pm daily . These goats were administered to identical feeding and management environmental conditions, and the shed was routinely sanitized and poop removed. These Shanbei White Cashmere goats were exposed to photoperiod treatment between 15 May 2020 and 15 October 2020 (153 days in total). The sampling period lasted from 15 May 2020 to 15 May 2021 (365 days in total).
Cashmere collection
On May 15, a 10-by-10-centimeter region on the right side of each goat was sheared to the skin level. Fibre samples from the shorn area were clipped at skin level using stainless steel clippers on 21 July and 21 August. Each clipping was obtained adjacent to the location of the last shearing but was always distinct from any previously sampling location. A 30 mm2 patch of cashmere sample on the left scapular region of each goat was obtained at the beginning of harvest in the following April. Samples were separated into cashmere fibre and guard hair samples. Then, the cashmere fibre samples were stored in sealed polythene bags at room temperature for fibre characteristic analyses. Cashmere samples were collected annually at the end of April by combing or shearing and weighed using an electronic scale (CP214; OHAUS, USA).
Cashmere measurement
The cashmere fibre samples were soaked in carbon tetrachloride detergent solution overnight, rinsed thoroughly washed with deionized water and dried at 80°C. A total of 100 fibres were randomly chosen from each sampling date of each goat to measure the stretched length of the cashmere using a steel ruler to calculate the amount of cashmere growth, while 200 fibres were randomly selected for the diameter measurement using an optical fibre diameter analyzer (FZ-002; SRI, China).
Hair follicle density measurement
From the body side of the goats, approximately 1 cm2 of skin tissues was taken, placed in Sample Protector (Takara, Dalian, China) and frozen at −80°C. After drying, the skin tissues were trimmed according to the cross-section, dehydrated by gradient ethanol, cleaned with xylene, embedded in paraffin, stained with HE, sealed with neutral gum and observed. Using digital microphotography (KEYENCE, Japan), The number of primary and secondary hair follicles in 10 complete hair follicles was recorded. ImageJ 1.60 image processing software was utilized to calculate the density of PHFs and SHFs in the skin area. The ratio of PHFs and SHFs was calculated.
Blood sampling
Blood samples were collected from a jugular vein using 5 mL EDTA-coated vacutainer tubes (KANGJIAN, China) to examine the effect of photoperiod treatment on hormone levels and hormone receptor gene expression in Shaanbei cashmere goats. Blood was drawn on the 15th of each month at 9:30 am Blood samples were allowed to stand at 37°C for 30 min before being centrifuged at 3 000 r / min for 15 min. The upper serum samples were collected and stored at −20°C for further analysis.
Hormone assay
Serum melatonin concentrations were measured using a commercial radioimmunoassay kit (HY 10177). The inter and intra-assay coefficients of variation (CVs) were 14% and 8.3%, respectively, while the sensitivity of the assay was 1.0 pg/ml. Serum prolactin concentrations were assessed using a 125I-prolactin radioimmunoassay kit (HY-10026), and inter and intra-assay CVs were 10% and 5%, respectively. The sensitivity of the assay was 1.0 ng/ml. All commercial kits were provided by SINO-UK Institute of Biological Technology (Beijing, China).
Quantitative real-time polymerase chain reaction (qRT-PCR)
According to the manufacturer’s instructions, total RNA was extracted from skin tissues using the Eastep® Super Total RNA Extraction Kit (Promega, Shanghai, China). The concentration and quality of the extracted total RNA were assessed with the NanoDrop spectrophotometer (Bio-Rad, Benicia, USA). Complementary DNAs (cDNAs) were obtained from the reverse transcription of RNA with oligo (dT) primer using M-MLV reverse transcriptase kit (TaKaRa, Dalian, China). qRT-PCR was performed in triplicate on a Bio-Rad IQ5 Real-Time PCR system with a final volume of 25 μl containing 12.5 μl 2×SYBR® Premix Ex TaqTMII (TaKaRa, Dalian, China), 1 μl of forward or reverse primer (10 μmol/L), 2 μl diluted template cDNA and 8.5 μl ddH2O. The reaction conditions were carried out in accordance with the manufacturer's guidelines. Three technical replicates were allocated to each sample, and the average Ct value was determined. Using succinate dehydrogenase complex flavoprotein subunit A (SDHA) as the reference gene (Bai et al. Citation2014), the 2-ΔΔCt technique was used to calculate the relative expression of each gene. All sequences of primers are shown in .
Table 1. Primer sequences for quantitative real-time polymerase chain reaction (PCR).
Statistical analysis
The data were done using Statistical Package for the Social Sciences (SPSS) ver. 20.0 (SPSS Inc., Chicago, IL, USA). The Shapiro–Wilk test was used to examine the data's normality. Student's t-test was used to examine differences between groups, and P values less than 0.05 were considered statistically significant. Results were presented as mean ± standard error (SE).
Results
Cashmere growth, fibre quality and hair follicle density
The cashmere treated with short-day photoperiod treatment began to develop earlier, and its length increased significantly from June to July and September to April the following year (P < 0.05; A). In June and July, the cashmere growth rate increased significantly (P < 0.05; B). The effect of short-day photoperiod treatment on the weight of cashmere fluff mixes and cashmere fibre diameter was insignificant (P > 0.05; ). The cashmere fibre length of the treatment group rose by 1.25 cm (P < 0.05), although the cashmere weight did not differ substantially. (P > 0.05; ).
Figure 1. Effects of short-day photoperiod treatment on growth length and growth rate of Shaanbei white cashmere goats. (A) Cashmere growth length of Shaanbei white cashmere goats. * P < 0.05. (B) Cashmere growth rate of Shaanbei white cashmere goats.Different letters means significant difference P < 0.05. Control: Long-day photoperiod treatment group; Treatment: Short-day photoperiod treatment group.
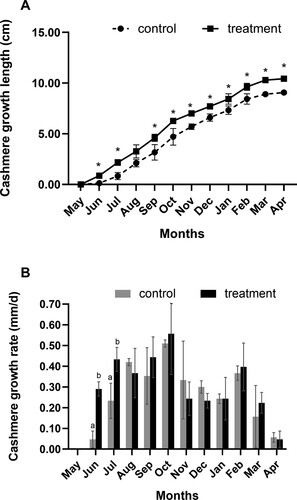
Table 2. Effects of short-day photoperiod treatment on cashmere fibre quality of Shaanbei white cashmere goats. Data are expressed as mean ± standard error (SE).
Compared to the long-day photoperiod treatment, the short-day photoperiod treatment significantly decreased the primary hair follicle density in September (P < 0.05; ), and increased the secondary hair follicle density in June and July (P < 0.05; ). April had the lowest ratio of secondary to primary follicle density (S:P). In July and September, short-day photoperiod treatment significantly enhanced the S:P ratio (P < 0.05; ).
Table 3. Effects of short-day photoperiod treatment on hair follicle density of Shaanbei white cashmere goats. Data are expressed as mean ± standard error (SE).
Serum hormone concentration
In June, July and September, Short-day photoperiod treatment significantly raised MT concentrations (P < 0.05; A). In the short-day photoperiod treatment group, the cyclical fluctuations of MT concentrations fluctuated differently than in the long-day photoperiod treatment group from May to August, and MT concentrations kept at a high level from June to October in the short-day photoperiod treatment group (A).
Figure 2. Effects of short-day photoperiod treatment on related hormone concentrations of Shaanbei white cashmere goats. (A) Melatonin (MT). (B) Prolactin (PRL). (C) Thyroxine (T4). (D) Estradiol (E2). * P < 0.05. Control: Long-day photoperiod treatment group; Treatment: Short-day photoperiod treatment group.
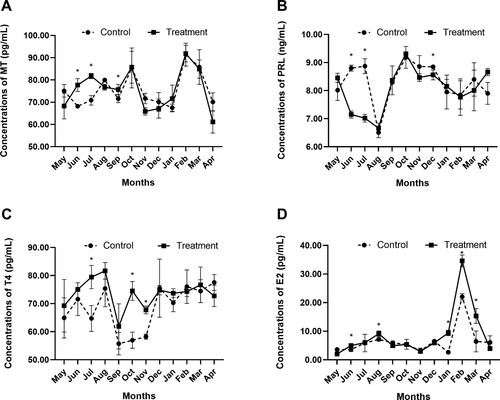
The initial peak of prolactin (PRL) concentrations in serum of long-day photoperiod treatment group occurred in July, followed by the second peak in October. Short-day photoperiod treatment significantly reduced PRL concentrations in June, July and December (P < 0.05; B) and altered the periodic fluctuation of PRL (B).
Short-day photoperiod treatment increased thyroxine 4 (T4) concentrations significantly in July, October and November (P < 0.05), but no significant difference in other months (C).
In the short-day photoperiod treatment group, blood E2 concentrations were substantially higher in June, August, January, February and March (P < 0.05; D).
Hormone receptor expression
In the long-day photoperiod treatment group, retinoid-related orphan receptor alpha (RORα) expression was lower in August, October and February and greater in November, March and April. The expression of RORα was considerably up-regulated in June, October, December and February and significantly down-regulated in July, November, January, March and April in the short-day photoperiod treatment group (P < 0.05; A), delaying the expression peak to December (A).
Figure 3. Effects of short-day photoperiod treatment on related hormone receptor expression of Shaanbei white cashmere goats. (A) Retinoid-related orphan receptor alpha (RORα).(B) Prolactin receptor (PRLR).(C) Thyroid hormone receptor alpha (TRα).(D) Thyroid hormone receptor beta (TRβ). (E) Estrogen receptor alpha (ERα). (F) Estrogen receptor beta (ERβ). * P < 0.05. Control: Long-day photoperiod treatment group; Treatment: Short-day photoperiod treatment group.
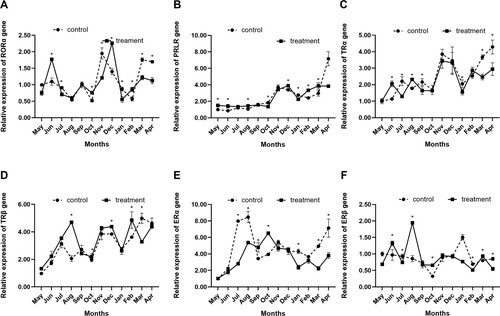
As shown in , the expression of prolactin receptor (PRLR) in the long-day photoperiod treatment group increased from May until November, when it reached its initial peak, and then decreased. The short-day photoperiod treatment group showed similar patterns. With the short-day photoperiod treatment, PRLR expression was substantially higher in May, June, August, December, February and March (P < 0.05) and significantly lower in October and January (P < 0.05; B).
The expression of thyroid hormone receptor alpha (TRα) in the short-day photoperiod treatment group was significantly higher in June and August and significantly lower in July, September, January, March and April (P < 0.05; C).
The expression of thyroid hormone receptor beta (TRβ) in the long-day photoperiod treatment group showed a trend of increasing volatility from May and peaked in March of the following year. The short-day photoperiod treatment group advanced the expression peak of TRβ to February, significantly up-regulated the expression of TRβ in August, October, December and February, and significantly down-regulated the expression of TRβ in March (P < 0.05; D).
The expression of estrogen receptor alpha (ERα) in the long-day photoperiod treatment group increased from May, reached a peak in August and then dropped. Short-day photoperiod treatment group significantly down-regulated the expression of ERα in July, August, January, March and April (P < 0.05). In September and October, the concentrations of ERα were elevated in the short-day photoperiod group (P < 0.05). The peak was postponed to October (E).
In the long-day photoperiod treatment group, the expression of estrogen receptor beta (ERβ) was lowest in October and highest in January. In the short-day photoperiod treatment group, ERβ expression was significantly up-regulated (P < 0.05) in June, August, October and March, and significantly down-regulated in July, February and April (P < 0.05; F), with the peak occurring in August.
Gene expression associated with hair follicle development
The expression of β-catenin in two groups increased gradually from May and reached the highest peak in November and then decreased. From May to July, the expression of β-catenin and Platelet Derived Growth Factor Subunit A (PDGFA) was considerably upregulated by Short-day photoperiod treatment (P < 0.05; A; B). With short-day photoperiod treatment, the first expression peak of PDGFA was observed in August, one month earlier than with long-day photoperiod treatment (B). Compared with the long-day photoperiod treatment, the short-day photoperiod treatment increased the expression of bone morphogenetic protein-2 considerably from June to August (P < 0.05; C). Short-day photoperiod treatment reduced the expression of bone morphogenetic protein-4 during July (P < 0.05; D). Except for March, short-day photoperiod treatment dramatically raised the expression of Fibroblast growth factor 5 gene in all months (P < 0.05; E).
Figure 4. Effects of short photoperiod treatment on the hair follicle development-related gene expression of Shaanbei white cashmere goats. (A) Catenin beta-1 (β-catenin). (B) Platelet-derived growth factor subunit A (PDGFA). (C) Bone morphogenetic protein 2 (BMP2). (D) Bone morphogenetic protein 4 (BMP4). * P < 0.05. Control: Long-day photoperiod treatment group; Treatment: Short-day photoperiod treatment group.
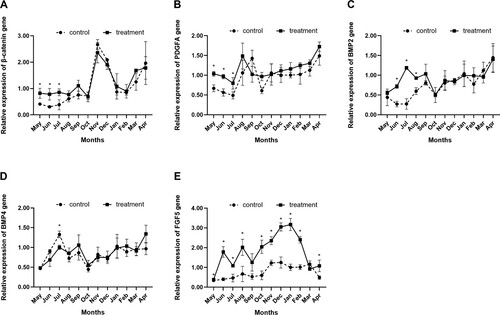
Discussion
Length, diameter and yield are important traits of cashmere, which determine the economic value of cashmere. Feral doe goats have seasonal characteristics, and the change of illumination time affects the growth rate, length and diameter of Australian cashmere goats (McDonald and Hoey Citation1987). The results of our investigation revealed that the short-day photoperiod treatment boosted cashmere length by 1.25 cm and cashmere output by 99.34 g, which increased by 16.75% year-on-year. Both hair follicle density and the S:P ratio are essential morphological parameters of villus hair follicles, having high heritability related to sheep wool production (Ansari-Renani et al. Citation2011). Previous research has demonstrated that melatonin can stimulate the growth of hair follicles (Feng and Gun Citation2021). Embedding MT in vitro induced cashmere growth in advance, prolonged the growth of the cashmere and increased the length of the cashmere (Welch et al. Citation1990; Cong et al. Citation2011). In this study, short-day photoperiod treatment reduced sunshine hours in June, July and September, and as melatonin production is directly related to light, there was less melatonin secretion during the day and more at night (Bedrosian et al. Citation2013). Due to the prolonged darkness, the MT concentration of the short-day photoperiod treatment group was significantly higher than that of the long-day photoperiod treatment group in June, July and September, which advanced the reconstruction of secondary hair follicles and stimulated the accelerated growth of cashmere.
MT has both membrane receptors (MT1, MT2 and MT3) and nuclear receptors (RORα, RORβ and RORγ) (Fischer et al. Citation2008; Cutando et al. Citation2011). The expression of membrane receptor was not detected in goat skin (Dicks et al. Citation1996). In addition, a prior study demonstrated that RORα was expressed in the secondary hair follicles of the hair shaft, inner root sheath, outer root sheath and medulla (Zhao et al. Citation2015). On the basis of these studies, we detected the relative expression of RORα gene in the samples and found that the expression of RORα in the long-day photoperiod treatment group peaked in November, but the short-day photoperiod treatment delayed the peak expression to December. Melatonin affects the transcriptional control of ROR (Karasek et al. Citation2003), and Fischer suggests that MT mediates the role of RORα in regulating hair growth (Fischer et al. Citation2008). Therefore, we compared MT concentration and RORα expression, and found that in the long-day photoperiod treatment group, RORα expression and MT concentration appeared opposite peaks in October, February and November. The result confirmed the negative correlation between MT concentration and RORα expression (Wang et al. Citation2013). In June and January, however, contrasting results emerged in the short-day photoperiod treatment group. We hypothesize that short-day treatment could affect the interaction between MT and RORα. But the relevant mechanism of action has not been elucidated and there are various controversies as to whether MT directly impacts RORα (Ma et al. Citation2021), so further research is required.
Previous research indicates that PRL has an inhibitory effect on hair cycle, with high concentrations promoting the termination of cashmere growth (Kloren and Norton Citation1993; Dicks et al. Citation1994; Craven et al. Citation2001). This is consistent with the results of our study. During the early stage of cashmere growth, from May to August, PRL concentrations were considerably lower in the short-day photoperiod treatment group than in the long-day photoperiod treatment group, Additionally, PRL and MT levels exhibited opposing tendencies simultaneously. MT is able to reduce PRL secretion (Rose et al. Citation1985; Emesih et al. Citation1993; Nixon et al. Citation1993; Dicks et al. Citation1995; Duan et al. Citation2017). By boosting MT concentrations to inhibit PRL levels in cashmere's early growth period, short-day photoperiod treatment may enhance the advanced growth of cashmere. PRL exerts its biological effects via receptor binding (Morammazi et al. Citation2016). During the anagen phase (August to December), high concentrations of PRL up-regulated PRLR expression, which peaked during the catagen (January to February) and telogen (March to April) phases, which supports the research findings of Nixon et al (Nixon et al. Citation2002).
T4 regulates the metabolism and growth of the body. Its secretion is related to the physiological state of an individual. The T4 concentrations of Australian cashmere goats were higher in the summer than in the winter, although the seasonal variation was not obvious (Kloren et al. Citation1993). The findings of this study agree with previous reports. Short-day photoperiod treatment significantly increased the T4 concentrations in the short-day photoperiod group in July, October and November, but did not significantly change the T4 secretion trend. In addition, short-day photoperiod treatment had no significant effect on their expression patterns.
The research conducted by Chanda demonstrated that E2 could promote suppression of the telogen to anagen transition. It induced hair follicles in the catagen phase to enter the telogen phase and regulated the hair follicle cycle (Chanda et al. Citation2000a). E2 increased gradually during the late catagen phase of SHFs (December). The SHFs were transformed from the anagen phase to catagen phase. In male mice, E2 regulated the vital movement of hair follicles by mediating the inhibition of telogen–anagen transition (Movérare et al. Citation2002). The catagen-promoting activities of E2 were mediated by Erα (Chanda et al. Citation2000b; Ohnemus et al. Citation2005). ERβ antagonized the mediated action of ERα and MT inhibited the expression of ERα (Ohnemus et al. Citation2005; Martinez-Campa et al. Citation2006). Our study demonstrated that short-day photoperiod treatment inhibited the expression of ERα between June and August, which is due to the increase of serum MT concentration and the up-regulation of expression of ERβ. These regulations attenuated the inhibition of hair follicle growth by E2 and promoted the growth and development of hair follicles.
Wnt / β-catenin signalling pathway plays a key role in the development of hair follicles. The expression of Wnt signalling can promote the development, occurrence and differentiation of hair follicles (Andl et al. Citation2002; Fu and Hsu Citation2013). Low expression of β-catenin stimulates hair follicle stem cell differentiation to epithelial and hair follicle sebaceous glands, while high expression of β-catenin induces hair follicle reconstruction (Silva-Vargas et al. Citation2005). Implantation of MT in vitro significantly up-regulated the expression of β-catenin gene in Inner Mongolia cashmere goats (Liu et al. Citation2016). The present study found that short-day photoperiod treatment boosted β-catenin gene expression during the early anagen phase of the cashmere growth (May to July).
The bone morphogenetic protein (BMP) signal pathway plays a crucial role in hair follicle morphogenesis and hair follicle cycling (Botchkarev and Kishimoto Citation2003). BMP signalling limits hair follicle cell growth and maintains hair follicles in the telogen period (Millar Citation2002). Our research revealed that short-day photoperiod treatment increased the expression of BMP2 gene during the early anagen phase, but had no effect on the expression of BMP4 during the telogen and catagen phases.
PDGF is a cell growth factor that inhibits apoptosis (Gruber et al. Citation2000). It is associated with hair follicle growth and angiogenesis (Kamp et al. Citation2003). Insulin-like growth factor 1 (IGF-1) treatment up-regulated the expression of PDGFA and platelet-derived growth factor subunit B (PDGFB), thereby inhibiting cell apoptosis. These regulations promote hair follicle growth and maintain hair follicles in the anagen phase (Ahn et al. Citation2012; Pazzaglia et al. Citation2019). Our research demonstrated that short-day photoperiod treatment increased PDGFA gene expression significantly during the early anagen phase.
Noggin is an inhibitor of BMP (Zimmerman et al. Citation1996). BMP is located downstream of Wnt /β-catenin (Suzuki et al. Citation2009). High expression of β-catenin up-regulated noggin expression (Plikus et al. Citation2008). PDGFA and SHH enhanced the expression of the noggin gene in dermal papilla cells. By upregulating the expression of -catenin and PDGFA genes in anagen, short-day photoperiod treatment suppressed the expression of the BMP4 gene, hence encouraging hair follicle rebuilding.
It has been reported that human hair follicles cultured in vitro and exposed to recombinant FGF5 enters into catagen phase prematurely (Higgins et al. Citation2014). In the early stages of the study, the serum FGF5 concentration and cashmere length of goats in the short-day photoperiod treatment group were significantly higher than those of the control group, suggesting that the increase in serum FGF5 concentration lead to the catagen phase of hair follicles, thus promoting cashmere growth.
Conclusion
In summary, under the short-day photoperiod treatment, primary hair follicle density dropped by 0.91 follicles/mm2 in September, secondary hair follicle density rose by 5.52 and 11.31 follicles/mm2 in June and July. In addition, short photoperiod treatment elevated serum MT concentration, decreased serum PRL concentration, down-regulated the expression of ERα and up-regulated the expression of hair follicle development-related genes (β-catenin, BMP2,FGF5 and PDGFA). These changes accelerated the reconstruction and increased the density of secondary hair follicles, as well as raised the S:P ratio, which stimulated the faster growth and increased the quantity of cashmere.
Disclosure statement
No potential conflict of interest was reported by the author(s).
Additional information
Funding
References
- Ahn, S.-Y., Pi, L.-Q., Hwang, S.T., Lee, W.-S., 2012. Effect of IGF-I on hair growth is related to the anti-apoptotic effect of IGF-I and up-regulation of PDGF-A and PDGF-B. Ann Dermatol. 24, 26-31. doi:10.5021/ad.2012.24.1.26
- Andl, T., Reddy, S.T., Gaddapara, T., Millar, S.E., 2002. WNT signals are required for the initiation of hair follicle development. Dev Cell 2, 643-653. doi:10.1016/S1534-5807(02)00167-3
- Ansari-Renani, H., Ebadi, Z., Moradi, S., Baghershah, H., Ansari-Renani, M., Ameli, S., 2011. Determination of hair follicle characteristics, density and activity of Iranian cashmere goat breeds. Small Ruminant Res 95, 128-132. doi:10.1016/j.smallrumres.2010.09.013
- Bai, W. L., Dang, Y. L., Wang, J. J., Yin, R. H., Wang, Z. Y., Zhu, Y. B., Cong, Y. Y., Xue, H. L., Deng, L., Guo, D., Wang, S. Q., Yang, S. H. 2016. Molecular characterization, expression and methylation status analysis of BMP4 gene in skin tissue of Liaoning cashmere goat during hair follicle cycle. Genetica, 144(4): 457-467. doi:10.1007/s10709-016-9914-1
- Bai, W.L., Yin, R.H., Yin, R.L., Jiang, W.Q., Wang, J.J., Wang, Z.Y., Zhu, Y.B., Zhao, Z.H., Yang, R.J., Luo, G.B., He, J.B., 2014. Selection and validation of suitable reference genes in skin tissue of Liaoning cashmere goat during hair follicle cycle. Livest Sci 161, 28–35. doi:10.1016/j.livsci.2013.12.031
- Bedrosian, T.A., Herring, K.L., Walton, J.C., Fonken, L.K., Weil, Z.M., Nelson, R.J., 2013. Evidence for feedback control of pineal melatonin secretion. Neurosci Lett 542, 123–125. doi:10.1016/j.neulet.2013.03.021
- Blanpain, C., Fuchs, E., 2006. Epidermal stem cells of the skin. Annu Rev Cell Dev Biol. 22, 339-373. doi:10.1146/annurev.cellbio.22.010305.104357
- Botchkarev, V.A., Kishimoto, J., 2003. Molecular control of epithelial–mesenchymal interactions during hair follicle cycling. J Investig Dermatol Symp Proc, pp. 46-55. doi:10.1046/j.1523-1747.2003.12171.x
- Cassone, V.M., 1990. Effects of melatonin on vertebrate circadian systems. Trends Neurosci 13, 457-464. doi:10.1016/0166-2236(90)90099-V
- Chanda, S., Robinette, C.L., Couse, J.F., Smart, R.C., 2000a. 17beta-estradiol and ICI-182780 regulate the hair follicle cycle in mice through an estrogen receptor-alpha pathway. Am J Physiol Endocrinol Metab 278, E202-E210. doi:10.1152/ajpendo.2000.278.2.E202
- Chanda, S., Robinette, C.L., Couse, J.F., Smart, R.C., 2000b. 17β-Estradiol and ICI-182780 regulate the hair follicle cycle in mice through an estrogen receptor-α pathway. Am J Physiol Endocrinol Metab 278, E202-E210. doi:10.1152/ajpendo.2000.278.2.E202
- Cong, Y., Deng, H., Feng, Y., Chen, Q., Sun, Y., 2011. Melatonin implantation from winter solstice could extend the cashmere growth phase effectively. Small Ruminant Res 99, 48-53. doi:10.1016/j.smallrumres.2011.03.055
- Craven, A.J., Ormandy, C.J., Robertson, F.G., Wilkins, R.J., Kelly, P.A., Nixon, A.J., Pearson, A.J., 2001. Prolactin signaling influences the timing mechanism of the hair follicle: analysis of hair growth cycles in prolactin receptor knockout mice. Endocrinology 142, 2533-2539. doi:10.1210/endo.142.6.8179
- Cutando, A., Aneiros-Fernández, J., López-Valverde, A., Arias-Santiago, S., Aneiros-Cachaza, J., Reiter, R.J., 2011. A new perspective in oral health: potential importance and actions of melatonin receptors MT1, MT2, MT3, and RZR/ROR in the oral cavity. Arch Oral Biol 56, 944-950. doi:10.1016/j.archoralbio.2011.03.004
- Dicks, P., Morgan, C., Morgan, P.J., Kelly, D., Williams, L., 1996. The localisation and characterisation of insulin-like growth factor-I receptors and the investigation of melatonin receptors on the hair follicles of seasonal and non-seasonal fibre-producing goats. J Endocrinol 151, 55-63. doi:10.1677/joe.0.1510055
- Dicks, P., Russel, A., Lincoln, G., 1994. The role of prolactin in the reactivation of hair follicles in relation to moulting in cashmere goats. J Endocrinol 143, 441-448. doi:10.1677/joe.0.1430441
- Dicks, P., Russel, A., Lincoln, G., 1995. The effect of melatonin implants administered from December until April, on plasma prolactin, triiodothyronine and thyroxine concentrations and on the timing of the spring moult in cashmere goats. Anim Sci 60, 239-247 doi:10.1017/S1357729800008407
- Duan, C., Xu, J., Zhang, Y., Zhang, W., Sun, Y., Jia, Z., 2017. Effects of melatonin implantation on cashmere growth, hormone concentrations and cashmere yield in cashmere-perennial-type Liaoning cashmere goats. Anim Prod Sci 57, 60-64. doi:10.1071/AN15183.
- Emesih, G., Newton, G., Teh, T., Zia, J.-H., 1993. Effects of photoperiod and continuous administration of melatonin on plasma concentrations of prolactin in cashmere goats. Small Ruminant Res 11, 247-256. doi:10.1016/0921-4488(93)90049-N
- Feng, Y., Gun, S., 2021. Melatonin supplement induced the hair follicle development in offspring rex rabbits. J Anim Physiol Anim Nutr (Berl) 105, 167-174. doi:10.1111/jpn.13417
- Fischer, T.W., Slominski, A., Tobin, D.J., Paus, R., 2008. Melatonin and the hair follicle. J Pineal Res 44, 1-15. doi:10.1111/j.1600-079X.2007.00512.x
- Foitzik, K., Krause, K., Conrad, F., Nakamura, M., Funk, W., Paus, R., 2006. Human scalp hair follicles are both a target and a source of prolactin, which serves as an autocrine and/or paracrine promoter of apoptosis-driven hair follicle regression. Am J Pathol 168, 748-756. doi:10.2353/ajpath.2006.050468
- Fu, J., Hsu, W., 2013. Epidermal Wnt controls hair follicle induction by orchestrating dynamic signaling crosstalk between the epidermis and dermis. J Invest Dermatol 133, 890-898. doi:10.1038/jid.2012.407
- Gruber, H.E., Norton, H.J., Hanley Jr, E.N., 2000. Anti-apoptotic effects of IGF-1 and PDGF on human intervertebral disc cells in vitro. Spine 25, 2153-2157. doi:10.1097/00007632-200009010-00002
- Higgins, C., Petukhova, L., Harel, S., Ho, Y.-Y., Drill, E., Shapiro, L., Wajid, M., Christiano, A., 2014. FGF5 is a crucial regulator of hair length in humans. Proc Natl Acad Sci U S A 111. doi:10.1073/pnas.1402862111
- Kamp, H., Geilen, C., Sommer, C., Blume-Peytavi, U., 2003. Regulation of PDGF and PDGF receptor in cultured dermal papilla cells and follicular keratinocytes of the human hair follicle. Exp Dermatol 12, 662-672. doi:10.1034/j.1600-0625.2003.00089.x
- Kandyba E, Leung Y, Chen Y B, Widelitz R, Chuong C M, Kobielak K. 2013. Competitive balance of intrabulge BMP/Wnt signaling reveals a robust gene network ruling stem cell homeostasis and cyclic activation. Proc Natl Acad Sci U S A, 110(4): 1351-1356. doi:10.1073/pnas.1121312110
- Karasek, M., Gruszka, A., Lawnicka, H., Kunert-Radek, J., Pawlikowski, M., 2003. Melatonin inhibits growth of diethylstilbestrol-induced prolactin-secreting pituitary tumor in vitro: possible involvement of nuclear RZR/ROR receptors. J Pineal Res 34, 294-296. doi:10.1034/j.1600-079X.2003.00046.x
- Kloren, W., Norton, B., 1993. Fleece growth in Australian cashmere goats. IV. The role of prolactin in the initiation and cessation of cashmere growth. Aust J Agric Res 44, 1051-1061. doi:10.1071/AR9931051
- Klören, W., Norton, B., 1995. Melatonin and fleece growth in Australian cashmere goats. Small Ruminant Res 17, 179-185. doi:10.1016/0921-4488(95)00659-9
- Kloren, W., Norton, B., Waters, M., 1993. Fleece growth in Australian cashmere goats. III. The seasonal patterns of cashmere and hair growth, and association with growth hormone, prolactin and thyroxine in blood. Aust J Agric Res 44, 1035-1050. doi:10.1071/AR9931035
- Liu, B., Gao, F., Guo, J., Wu, D., Hao, B., Li, Y., Zhao, C., 2016. A microarray-based analysis reveals that a short photoperiod promotes hair growth in the Arbas cashmere goat. PloS one 11, e0147124. doi:10.1371/journal.pone.0147124
- Liu, H., Liu, C., Yang, G., Li, H., Dai, J., Cong, Y., Li, X., 2012. DNA polymorphism of insulin-like growth factor-binding protein-3 gene and its association with cashmere traits in cashmere goats. Asian-Australas J Anim Sci 25, 1515. doi:10.5713/ajas.2012.12351
- Ma, H., Kang, J., Fan, W., He, H., Huang, F., 2021. ROR: nuclear receptor for melatonin or not? Molecules 26, 2693. doi:10.3390/molecules26092693
- Martinez-Campa, C., Alonso-González, C., Mediavilla, M., Cos, S., Gonzalez, A., Ramos, S., Sánchez-Barceló, E., 2006. Melatonin inhibits both ERα activation and breast cancer cell proliferation induced by a metalloestrogen, cadmium. J Pineal Res 40, 291-296. doi:10.1111/j.1600-079X.2006.00315.x
- McDonald, B., Hoey, W., 1987. Effect of photo-translation on fleece growth in cashmere goats. Aust J Agric Res 38, 765-773. doi:10.1071/AR9870765
- Millar, S.E., 2002. Molecular mechanisms regulating hair follicle development. J Invest Dermatol 118, 216-225. doi:10.1046/j.0022-202x.2001.01670.x
- Morammazi, S., Masoudi, A.A., Vaez Torshizi, R., Pakdel, A., 2016. Changes in the expression of the prolactin receptor (PRLR) gene in different physiological stages in the mammary gland of the Iranian adani goat. Reprod Domest Anim Zuchthyg 51, 585-590. doi:10.1111/rda.12723
- Movérare, S., Lindberg, M.K., Ohlsson, C., Faergemann, J., Gustafsson, J.-Å., 2002. Estrogen receptor α, but not estrogen receptor β, is involved in the regulation of the hair follicle cycling as well as the thickness of epidermis in Male mice. J Invest Dermatol 119, 1053-1058. doi:10.1046/j.1523-1747.2002.00637.x
- Myllylä R M, Haapasaari K M, Lehenkari P, Tuukkanen J. 2014. Bone morphogenetic proteins 4 and 2/7 induce osteogenic differentiation of mouse skin derived fibroblast and dermal papilla cells. Cell Tissue Res, 355(2): 463-470. doi:10.1007/s00441-013-1745-0
- Nixon, A., Ford, C., Wildermoth, J., Craven, A., Ashby, M., Pearson, A., 2002. Regulation of prolactin receptor expression in ovine skin in relation to circulating prolactin and wool follicle growth status. J Endocrinol 172, 605-614. doi:10.1677/joe.0.1720605
- Nixon, A.J., Choy, V.J., Parry, A.L., Pearson, A.J., 1993. Fiber growth initiation in hair follicles of goats treated with melatonin. J Exp Zool 267, 47-56. doi:10.1002/jez.1402670108
- Ohnemus, U., Uenalan, M., Conrad, F., Handjiski, B., Mecklenburg, L., Nakamura, M., Inzunza, J., Gustafsson, J.-A.K., Paus, R., 2005. Hair cycle control by estrogens: catagen induction via estrogen receptor (ER)-α is checked by ERβ signaling. Endocrinology 146, 1214-1225. doi:10.1002/jez.1402670108
- Pazzaglia, I., Mercati, F., Antonini, M., Capomaccio, S., Cappelli, K., Dall’Aglio, C., La Terza, A., Mozzicafreddo, M., Nocelli, C., Pallotti, S., Pediconi, D., Renieri, C., 2019. PDGFA in cashmere goat: a motivation for the hair follicle stem cells to activate. Animals (Basel) 9, 38. doi:10.3390/ani9020038
- Plikus, M.V., Baker, R.E., Chen, C.C., Fare, C., de la Cruz, D., Andl, T., Maini, P.K., Millar, S.E., Widelitz, R., Chuong, C.M., 2011. Self-Organizing and stochastic behaviors during the regeneration of hair stem cells. Science 332, 586-589. doi:10.1126/science.1201647
- Plikus, M.V., Mayer, J.A., de La Cruz, D., Baker, R.E., Maini, P.K., Maxson, R., Chuong, C.-M., 2008. Cyclic dermal BMP signalling regulates stem cell activation during hair regeneration. Nature 451, 340. doi:10.1038/nature06457
- Rhind, S., McMillen, S., 1995. Seasonal changes in systemic hormone profiles and their relationship to patterns of fibre growth and moulting in goats of contrasting genotypes. Aust J Agric Res 46, 1273-1283. doi:10.1071/AR9951273
- Rile, N., Liu, Z., Gao, L., Qi, J., Zhao, M., Xie, Y., Su, R., Zhang, Y., Wang, R., Li, J., Xiao, H., Li, J., 2018. Expression of vimentin in hair follicle growth cycle of inner Mongolian cashmere goats. BMC Genomics 19, 38. doi:10.1186/s12864-017-4418-7
- Rose, J., Stormshak, F., Oldfield, J., Adair, J., 1985. The effects of photoperiod and melatonin on serum prolactin levels of mink during the autumn molt. J Pineal Res 2, 13-19
- Silva-Vargas, V., Celso, C.L., Giangreco, A., Ofstad, T., Prowse, D.M., Braun, K.M., Watt, F.M., 2005. β-catenin and hedgehog signal strength can specify number and location of hair follicles in adult epidermis without recruitment of bulge stem cells. Dev Cell 9, 121-131. doi:10.1111/j.1600-079X.1985.tb00624.x
- Suzuki, K., Yamaguchi, Y., Villacorte, M., Mihara, K., Akiyama, M., Shimizu, H., Taketo, M.M., Nakagata, N., Tsukiyama, T., Yamaguchi, T.P., 2009. Embryonic hair follicle fate change by augmented β-catenin through Shh and Bmp signaling. Development 136, 367-372. doi:10.1242/dev.021295
- Wang, L., Cai, T., Ou, Y.X.Q., Zu, H. L., Zhao, Y. H. 2013. Research progress on physiological functions of melatonin nuclear receptor RORα and Its relation to the growth of cashmere. Chin J Anim Sci 49, 75-79. doi:10.3969/j.issn.0258-7033.2013.21.020
- Welch, R., Gurnsey, M., Betteridge, K., Mitchell, R., 1990. Goat fibre response to melatonin given in spring in two consecutive years. Proceedings of the New Zealand Society of Animal Production, pp. 335–338.
- Yang, C.H., Xu, J.H., Ren, Q.C., Duan, T., Mo, F., Zhang, W., 2019. Melatonin promotes secondary hair follicle development of early postnatal cashmere goat and improves cashmere quantity and quality by enhancing antioxidant capacity and suppressing apoptosis. J Pineal Res 67, e12569. doi:10.1111/jpi.12569
- Zhang, C.Z., Sun, H.Z., Li, S.L., Sang, D., Zhang, C.H., Jin, L., Antonini, M., Zhao, C.F., 2019. Effects of photoperiod on nutrient digestibility, hair follicle activity and cashmere quality in Inner Mongolia white cashmere goats. Asian-Australas J Anim Sci 32, 541-547. doi:10.5713/ajas.18.0154
- Zhang, Y., Wu, K., Wang, L., Wang, Z., Han, W., Chen, D., Wei, Y., Su, R., Wang, R., Liu, Z., 2020. Comparative study on seasonal hair follicle cycling by analysis of the transcriptomes from cashmere and milk goats. Genomics 112, 332-345. doi:10.1016/j.ygeno.2019.02.013
- Zhao, Y., Liu, Z., Wang, L., Xiao, H., Du, C., Zhang, Y., Su, R., Li, J., 2015. Expression of the RORα gene in inner Mongolian cashmere goat hair follicles. Genet Mol Res 14, 380-388. doi:10.4238/2015.January.23.11
- Zimmerman, L.B., De Jesús-Escobar, J.M., Harland, R.M., 1996. The Spemann organizer signal noggin binds and inactivates bone morphogenetic protein 4. Cell 86, 599-606. doi:10.1016/S0092-8674(00)80133-6