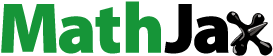
ABSTRACT
The effects of dietary Bacillus protease on broiler performance were examined. Three hundred ‘Cobb 500’ day-old broilers were randomly assigned to five dietary groups with five replicates of 12 birds each. Treatments include: PROT0; without protease addition (0 g/kg; control), PROT10, PROT15, PROT20 and PROT25 diets supplemented with Bacillus protease at the level of 1, 1.5, 2 and 2.5 g/kg, respectively. Birds fed PROT25 had the highest (p < 0.05) villi height and villi height to crypt depth ratio, as well as crypt depth at the duodenum, jejunum, and ileum of intestine. At starter and finisher phases, BWG was the highest (p < 0.05), whereas FI and FCR had their lowest values in PROT25 group (p < 0.05). PROT25 broilers had the best (p < 0.05) energy efficiency ratio. Crude protein, dry matter, and crude fibre digestibility were improved (p < 0.05) with increased inclusion levels of Bacillus protease. Lactobacillus and Bifidobacteria count increased (p < 0.05) as the inclusion levels of Bacillus protease increased in both ileum and caecum, while E. coli population decreased. Chickens fed with the PROT25 diets had the highest (p < 0.05) values for carcass, thigh, breast and drumstick weight. Cooking loss decreased (p < 0.05) as the inclusion levels of Bacillus protease increased, while water holding capacity increased (p < 0.05). Highest Bacillus protease (2.5 g/kg) inclusion improved digestibility, gut integrity, and health status with a better FCR, body weight gain, and retail cut yields.
1. Introduction
Diet formulation attracts great attention in poultry production, since it constitutes about 60–70% of the production cost (Oyeagu et al. Citation2019a; Wu et al. Citation2020). One of the biggest challenges to commercial poultry production is quality feeds at sustainable and stable prices. Kamel et al. (Citation2015) reported 80–85% digestibility of protein compared to 90% for starch in corn-soy diets. They argued that a certain amount of protein passed via gastrointestinal tract (GIT) undigested (Lemme et al. Citation2004; Schoenfeld and Aragon Citation2018). Feed ingredients of protein origin (soybean meal and sunflower meal) contain several anti-nutritional factors. Non-starch polysaccharides (NSP) are one of the anti-nutrient which reduce the nutrient bioavailability in poultry. These anti-nutritional factors suppress the production of insulin, which can impair glucose and amino acids uptake and utilization in the peripheral intestinal tissues such as striated muscle by non-ruminant animals, resulting in poor feed efficiency and growth (Masey O'Neill et al. Citation2014; Qaid and Abdelrahman Citation2016). An improved performance of birds was a result of exogenous enzyme effect (Shakouri et al. Citation2008; Oyeagu et al. Citation2019a). Furthermore, protein digestion in non-ruminant animals is promoted by the inherent proteases in two major stages: protein breakdown (gastric digestion) in an acidic setting and pancreatic digestion in the gut. The inherent proteases are produced and released in the GIT. They are relatively sufficient to optimize feed protein use (Ndazigaruye et al. Citation2019), but a significant amount of undigested protein (18–20%) escapes via the GIT (Lemme et al. Citation2004; Angel et al. Citation2011). Exogenous protease can enhance animal feed (Oyeagu et al. Citation2019b). According to Ahmed et al. (Citation2020) the supplementation of exogenous serine-protease (Ronozyme® ProAct) at levels of 200∼300 mg/kg can enhance animal protein utilization and resulted in positive effects on growth performance, nutrients utilization, cut yields and encourage beneficial bacteria in the intestine of broilers. Epithelium of the intestine operates as a natural obstruction against pathogenic bacteria and toxic substances. These bio-activities take place in the intestinal lumen. Some of the stressors (anti-nutritional factors and pathogens) cause disturbances in the normal micro-flora epithelium. They change the permeability of these natural obstructions and increase pathogens’ attacks, altering the metabolism process. They also impair the capacity to digest and absorb nutrients, resulting in chronic inflammatory activities at the intestinal mucosa (Markowiak and Śliżewska Citation2017; Pickard et al. Citation2017). The addition of protease induced some changes in microbial richness and diversity, and tended to change some specific microbial taxa (e.g. increase the abundance of Bacteroidetes). These microbes (Bacteroidetes) are very important because they produce the favourable metabolites, including SCFAs, which have been correlated with an alleviation of gut inflammation (Jeferson et al. Citation2020). Exogenous proteases may complement pancreatic enzymes and increase the rate of intestinal protein degradation (Mahmood et al. Citation2017). It has been demonstrated that exogenous protease can change gut morphology, pancreatic enzyme production and secretion, the microbial populations along the gastrointestinal tract, and the short chain fatty acid profile in the digesta (Cowieson Citation2010). Consequently, shorter villi speed up cell turnover and decrease digestive and absorptive activities (Kai Citation2021). According to Rodrigues and Choct (Citation2018), dietary phytase and protease reduced the viscosity of the digesta, the relative weight and length of the small intestine, and the number of goblet cells in the duodenum. The microbial community and its activities in the intestinal tract of broilers are influenced by diet composition. It has been demonstrated that anti-nutritional effects on broiler chicken are partly mediated through intestinal microbial activity (Adedokun and Olojede Citation2019; de Carvalho et al. Citation2021). However, the restriction on antibiotic growth promoters increased interest concerning the study of micro-flora in broiler GIT. Due to the ban, the persistent proliferation of diseases such as Clostridium perfringens (necrotic enteritis) and E. coli are expected (Yadav and Jha Citation2019; Jha et al. Citation2020). The exogenous enzymes help to remove/reduce anti-nutritional factors’ challenging effect (Oyeagu et al. Citation2015; Citation2016; Citation2019a). The use of protease as a dietary enzyme may offer an opportunity to overcome some of the potential drawbacks imposed by vegetable–protein-based diets that may negatively affect the carcass yield of chicken (Mushtag et al. Citation2009; Alagawany et al. Citation2018). Factors that influence meat quality can be controlled throughout the chicken production chain, mainly through diets or during slaughter and processing. The carcass yield is closely linked with the nutritional status of broilers since animals supplied with adequate nutrients will promote the development of muscle tissue (Dalólio et al. Citation2015; Oyeagu et al. Citation2019b). The inclusion of protease in the diet has improved protein digestibility and enhanced nutrient availability and absorption for feed conversion to meat yield (Dessimoni et al. Citation2019; Gervais et al. Citation2019). Protease inclusion in the corn-soybean diet can alter the nutritional status and improve the retail cut yields and meat quality of broiler chickens (Buyse et al. Citation2002; Erdaw et al. Citation2019). According to Medhi et al. (Citation2003), yield of chickens fed diets supplemented with multi-enzyme (xylanase, protease, and amylase) did not differ from those that received the control diet. diet. On the other hand, the inclusion of a blend of protease and xylanase to a fibrous diet improved carcass and retail cut yields (Khan et al. Citation2006). There is limited information on Bacillus protease effect on histomorphometric characteristics, gut microflora, and meat quality of broilers. Therefore, the present study aims to determine Bacillus protease's impact on intestinal micro-flora, histomorphology, meat quality, growth performance and carcass traits of broilers.
2. Materials and methods
2.1. Animal welfare statement
The authors confirm that the ethical policies that guide research of this nature were adhered to. The appropriate ethical review committee approval was received from the University of Fort Hare, South Africa.
2.2. Research trial location
The research trial was conducted at the poultry section of the North-West University research farm (Molelwane) in the North-West province of South Africa. The research area normally experience summer from August to March and has an ambient temperature within 22–35°C. The average yearly rainfall ranges between 200 and 450 mm.
2.3. Additive traits
Exogenous enzyme under investigation (RONOZYME® ProAct (RPA), DSM Nutritional Products Johannesburg South Africa) is a granulated heat-stable formulated product from Bacillus strain E A.1 with an enzyme activity of 75,000 PROT/g. One PROT is one protease unit and is defined as the amount of enzyme that releases one mmol of p-nitroaniline from one mM substrate (Suc-Ala-Ala-Pro-Phe-pNA) per Adler minute at pH 9.0 and 37°C. The Bacillus protease was selected as a feed enzyme due to its inherent bioactive traits. According to the manufacturer, at peptic and acidic conditions (pH), the enzyme retains more than 90% residual activity after 2hrs at 40°C (DSM).
2.4. Experimental diets
Iso-nitrogenous and iso-caloric experimental maize-soybean meal diets were used for the research trial. Starter (0–21 d) and finisher (22–42 d) feeds were formulated ( and ) to satisfy the birds’ dietary nutrient needs (NRC Citation1994). At each feeding phase (i.e. starter and finisher), five dietary treatments were formulated by adding the Bacillus protease at five different levels. The five experimental diets for starter and finisher generated were Protease0, Protease10, Protease15, Protease20 and Protease25 represented as PROT0 (only basal diet; BD), PROT10 (BD + 1 g protease/kg diet), PROT15 (BD + 1.5 g protease/kg diet), PROT20 (BD + 2 g protease/kg diet) and PROT25 (BD + 2.5 g protease/kg diet) respectively. Composition and proximate analysis of the five dietary groups (starter and finisher) are presented in and , respectively.
Table 1. Ingredient (%) and chemical composition (g/kg DM unless otherwise stated) of experimental diets for broiler chicks at the starter phase (0–3 weeks).
Table 2. Ingredient (%) and chemical composition (g/kg DM unless otherwise stated) of experimental diets for broilers at the finisher phase (4–6 weeks).
2.5. Research chickens and management
Three hundred-day-old chicken (Cobb 500®) was used for the research trial and was randomly allocated to the five dietary groups (PROT0, PROT10, PROT15, PROT20, or PROT25). Each dietary group was replicated into five cells, while 12 birds were allotted to each cell. The cells had wood shaving as litter. Chickens were offered diets and clean water ad libitum throughout the research trial. The research duration was 6-weeks.
2.6. Growth performance
Growth traits assessed were body weight, feed intake, and feed conversion ratio. Feed consumed was determined on a daily basis by removing the feed refusal from the provided feed, divided by the number of birds in each cell/replicate. Live weight was measured on a weekly basis for all the birds. The feed and live weight grams were determined by using a 10,100 g (10.1 kg) capacity precision weighing balance (A and D Weighing GF-10 K industrial balance, Japan). Feed conversion ratio was calculated by dividing feed consumed with weight gain.
2.7. Slaughter procedure
The chickens were sent to the abattoir (Rooigrond poultry abattoir, North West province, South Africa) for slaughter at the end of the feeding trial (day 42). The chickens were starved for 12 h before slaughter and gas stunned. The chickens were gas stunned by exposing them to relatively low concentrations of carbon dioxide (< 40% by volume in air), and then, once they were unconscious, exposed to a higher concentration (approximately 80% to 90% by volume in air). At the abattoir, all the chickens were hung onto a movable metal rack that held them upside down by their feet. Chickens were then sacrificed by cutting the jugular vein with a sharp knife and they were left hanging until bleeding stopped.
2.8. Carcass parameters and meat quality assessments
Carcass and meat quality traits were determined using 5 birds per pen selected at random immediately after slaughter when the feathers were plucked and the gastro intestinal tract (GIT) was removed. After cutting the carcass into different parts, the breast muscle, neck, wings, shank, thighs, drumsticks and vertebrae (back) were weighed separately. Furthermore, dressing percentage and cut yield of different cuts were also determined according to the following formulae:
2.9. Meat pH and temperature measurements
Measurements on meat pH and temperatures were recorded immediately after slaughter and 24 h post slaughter on the breast muscle (central area of the breast) using a Corning Model 4 pH-temperature meter (Corning Glass Works, Medfield, MA) equipped with an Ingold spear-type electrode (Ingold Messtechnik AG, Urdorf, Switzerland).
2.10. Meat colour
The meat colour was assessed as L* = Lightness, a* = Redness, and b* = Yellowness, 24 h after slaughter on the breast muscle using a Minolta colour-guide (BYK-Gardener GmbH, Geretsried, Germany).
2.11. Water holding capacity (WHC)
The water holding capacity was carried out using the pressure method as described by Delezie et al. (Citation2007). About 100 g chicken meat sample from the pectoral major (PM) was cut and weighed as initial weight using a digital sensitive scale up to 0.01 g. The meat sample was placed in between 2 filter papers, and placed on a flat surface. Furthermore, approximately 60 kg weight was applied on the sample for 5 min. The meat sample was weighed again to generate the final (second) weight of the sample. Water holding capacity was calculated as the ratio of the amount of water retained over the initial sample weight.
2.12. Drip loss
The drip loss involved the use of approximately 30 g meat strips samples from the breast muscle parallel to the fibre direction. The samples were weighed for the initial weight using a digital scale sensitive to 0.01 g. The samples were stored inside a plastic container, sealed under atmospheric pressure, and placed at 2°C for 72 h after which they were removed from the container. The samples were blotted with paper towels to remove excess surface moisture and were then re-weighed. The drip loss was then calculated by subtracting the blotted sample weight from the initial sample weight.
2.13. Cooking loss measurement
Raw meat cubes were cut from the breast muscle part of the chicken, weighed in natura, then placed in a plastic bag and cooked in a water bath at 75°C for 45 min (Rizz et al. Citation2007). The samples were cooled in running water for 15 min, dried and weighed (Sanka and Mbanga Citation2014). Cooking loss was calculated as percentage loss of weight after cooking relative to the weight of raw muscle samples before cooking (Gopinger et al. Citation2014) according to the following formula:
2.14. Tenderness
Samples of breast chicken muscles were wrapped in aluminium foil and baked to reach an internal temperature of 85°C, which was maintained for 30 min. Warner Bratzler shear device mounted on Universal Instron apparatus (cross head speed = 200 mm / minute, one shear in the centre of each core), was used for tenderness analysis. The average peak force value of each sample (in Newtons) was recorded using the Texture analyser (TA XT plus).
2.15. Gut histomorphological analysis
Gut histological analysis was implemented using two chickens from each cell at the end of the research trial. About 2 cm segment of different intestinal sections (duodenum, jejunum and ileum) were collected from each selected bird, rinsed with a 0.9% saline and fixed at 10% buffered formalin solution. The samples were maintained in the formalin at room temperature until analyses. Samples were transferred to the laboratory for histological examination and this involved washing, trimming, dehydration with alcohol, clearing with xylene and impregnation with paraffin wax. For each segment of intestine, tissue sections of 2 µm were cut by a microtome, fixed on slides and stained using haematoxylin and eosin (H and E) for histological analysis. A 2.5× magnification objective lens of a Zeiss Axio light microscope, equipped with a digital camera, was used to examine slides. Images were analysed using Axiovision image-analysis software, version 4.7.2 (Carl Zeiss microscopy). Images was viewed to measure morphometric characteristics of the villi height (VH), villi width (VW), crypt depth (CD), VH/CD ratio, thickness of epithelium and thickness of muscularis.
2.16. Caecal and ileum microflora composition
Two chickens from each cell were used for microbial examination at the end of the research trial. The ileum and caeca parts of the gut were collected immediately under aseptic conditions into sterile glass bags and put on ice before they were transported to the laboratory for microbial examination. The contents of caecal and ileum were emptied in a new sterile bag and were immediately diluted 10-fold (ie 10% wt/vol) with sterile ice-cold anoxic PBS (0.1 m; pH 7.0) and subsequently homogenized for 3 min in a stomacher (Bagmixer 100 Minimix, Interscience, Arpents, France). After the homogenization of each of the caecal and ileum digesta, they were subjected to serial dilution from 10−1–10−7. The dilutions were later plated on duplicate selective agar media for inventory of target bacterial groups. In particular, E. Coli, Lactobacillus spp and Bifidobacterium spp were enumerated using VRB agar (MERCK, 1.01406), Rogosa agar (MERCK, 1.10660), and Beerens agar respectively according to Tuohy et al. (Citation2002). Plates were incubated at 39°C for 48–120 h anaerobically (Beerens, Rogosa agars) or 24–48 h anaerobically at 37°C (VRB agar). The colonies of the bacteria were recorded, and the average number of live bacteria was calculated based on the weight of original ileum and caecum contents. All the quantitative data generated were converted into logarithmic colony forming units (cfu/g) (Koc et al. Citation2010).
2.17. Nutrient utilization efficiency
2.17.1. Protein utilization efficiency
Daily protein intake (g); this was calculated as the CP (crude protein) percentage of the diet multiplied with the DFI (daily feed intake). Calculations were made for each bird, where, Total protein intake (TPI) was calculated and was used to calculate protein efficiency ratio (PER).
where TPI = total protein intake, FI = feed intake, CP = crude protein.
2.17.2. Energy utilization efficiency
From the two phases (starter and finisher) of feeding trial, Total metabolizable energy intake (TMEI) was calculated and was used to calculate energy efficiency ratio (EER). TMEI was calculated as ME of a diet in kcal multiplied by the feed intake during the whole experimental period.
where TMEI = Total metabolizable energy intake, FI = feed intake, ME = metabolizable energy
2.18. Nutrient digestibility trial
One week before the end of the research, two birds were removed from each cell to clean metabolic cages. There were three days of adaptation before the four-day sample (droppings) collection. The samples (droppings) were air dried at room temperature. Dried faecal samples were ground and analysed according to AOAC (Citation2006) methods. The original sample was weighed, the weighed sample was placed in a 105°C oven for 12–16 h, and the sample was reweighed. The dry matter and moisture content of the feedstuff were calculated. Crude protein was subjected to Kjeldahl method where a dried sample was boiled in sulphuric acid, was then diluted with water and neutralized with sodium hydroxide, and finally, the sample was distilled and the distilled ammonium was titrated with a known concentrate of sulphuric acid. To determine crude protein content, the nitrogen value from the procedure was multiplied by 6.25. The crude fat or aether extract (EE) procedure estimates the quantity of lipids. The dried samples were ground and extracted with an organic solvent for 4 h and the remaining residue was dried and weighed. Aether extract was then calculated as the difference between the original sample and the aether extract residue. The sample was boiled in a sulphuric acid solution for 30 min and rinsed, and then boiled in a sodium hydroxide solution for 30 min and rinsed. Finally, the residue was dried, weighed, ashed, and reweighed. The crude fibre content was estimated as the difference between the pre-ash weight and the post-ash weight. Nutrient digestibility was calculated as:
2.19. Statistical design and analysis
The study was subjected to a completely randomized design (Steel and Torrie Citation1980) using SAS's General Linear Model Procedure (Citation2010). The applied model is shown below:
where Aij = Dependent variable; µ = Overall mean; Bi = Treatment effect; and Eij = Residual error.
The Tukey test was used for mean significance at p < 0.05.
3. Results
3.1. Gut histology
shows the gut mucosal morphological development of birds fed different inclusion levels of Bacillus protease. The histomorphometric characteristics measured in the present study were influenced (p < 0.05) by the different supplemental levels of Bacillus protease. The thickness of muscularis in jejunum and that of epithelium in ileum section of the intestine did not differ (p > 0.05). Chickens that consumed PROT25 recorded a higher (p < 0.05) villi height, and villi height to crypt depth ratio, with a lower (p < 0.05) crypt depth in the duodenum, jejunum and ileum sections. Chickens that consumed the experimental diets (PROT10, PROT15, PROT20 and PROT25) had greater (p < 0.05) villi height than those of the PROT0 group (control diet). On the other hand, birds in the treatment groups had a decreased (p < 0.05) crypt depth compared to the control group in the three sections of the gut. There was an increased (p < 0.05) villi height to crypt depth ratio for the three gut sections (duodenum, jejunum, and ileum) in protease supplemented chickens compared to the controls (PROT0). and present the histomicrograph of broiler jejunum and ileum as affected by dietary supplementation with Bacillus protease. showed the histomicrograph of broiler Jejunum fed different levels of Bacillus protease, while represents the histomicrograph of ileum intestinal part of broilers.
Figure 1. Histomicrograph of the Jejunum of broilers fed PROT0, PROT10, PROT15, PROT20 and PROT25 which shows the villi height, crypt depth, thickness of the epithelium, and muscularis. Two birds from each cell/replicate were used for the analysis.
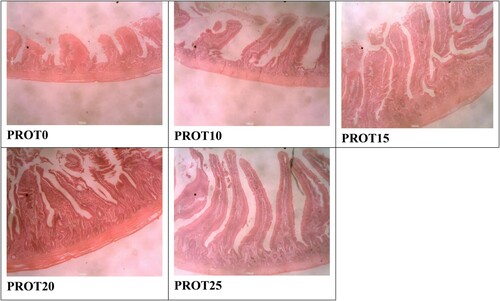
Figure 2. Histomicrograph of the ileum of broilers fed PROT0, PROT10, PROT15, PROT20 and PROT25 which shows the villi height, crypt depth, thickness of the epithelium, and muscularis. Two birds from each cell/replicate were used for the analysis.
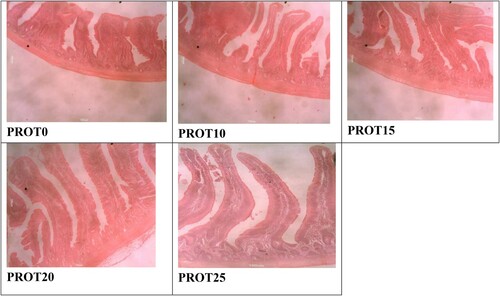
Table 3. The effect of dietary Bacillus protease supplementation on the gut histomorphometric traits of broiler birds.
3.2. Growth traits
shows the effect of Bacillus protease dietary supplementation on growth traits. The data for the starter phase (1 to 21 d) showed a lower (p < 0.05) value of FCR for chickens that consumed PROT25 than that of the other dietary groups (PROT0, PROT10, PROT15, and PROT20). It was noticed that BWG was higher (p < 0.05) for chickens fed PROT25, while their feed intake was the lowest (p < 0.05). Although, chickens that consumed dietary PROT0, PROT10, PROT15, and PROT20 had greater feed intake, yet they recorded lower values for BWG (p < 0.05). During the finisher phase (22 to 42d), the BWG of PROT25 birds was the highest (p < 0.05) but similar to that of PROT20 group. PROT10 birds displayed the lowest (p < 0.05) BWG similar to that of PROT0 group. Chickens that consumed the PROT25 diet had the lowest (p < 0.05) FCR of 1.23 while a higher (p < 0.05) FCR of 1.91 and 1.79 was observed in birds fed with PROT0 and PROT10 diets, respectively. Chickens fed PROT25 consumed less (p < 0.05) feed compared to that of PROT0, PROT10, PROT15 and PROT20 group. As shown on the overall phase performance, birds fed PROT25 recorded the lowest FI with a better (p < 0.05) BWG of 3060 g, than those that received dietary PROT0 and PROT10. Birds fed the PROT25 diet recorded a better FCR than those received the PROT0 and PROT10 diets.
Table 4. The effect of Bacillus protease inclusion on feed intake, body weight gain, and feed conversion ratio of broiler chicken.
3.3. Utilization efficiency of energy and protein
The impact of dietary Bacillus protease inclusion on protein/energy utilization efficiency is presented in . As indicated in all the phases (starter phase, finisher phase, and overall performance) of productions, the protein efficiency ratio (PER) of birds fed PROT25 had a greater value (p < 0.05) than that of birds of the other treatments (PROT0, PROT10, PROT15, and PROT20). The other treatment groups had lower (p < 0.05) intake of energy and a higher energy efficiency ratio compared to the control (PROT0) group at all phases or stages of production (starter phase, finisher phase, and overall performance). On the contrary, birds fed PROT25 consumed less (p < 0.05) energy and had the best energy efficiency ratio (EER) throughout the feeding trial. Daily protein intake increased (p < 0.05) in chickens that consumed the control, PROT10, PROT15, and PROT20 diets, while PROT25 fed birds consumed the lowest amount of protein. During the starter phase, protein intake for birds fed PROT0, PROT10, PROT15 and PROT20 was significantly (p < 0.05) higher, while birds fed PROT25 consumed less (p < 0.05) protein. The protein intake of chickens at the finisher and overall phases showed that birds fed PROT25 consumed less (p < 0.05) protein than those in other groups (PROT0, PROT10, PROT15 and PROT20).
Table 5. The effect of dietary protease supplementation on protein intake and protein efficiency ratio of broiler birds.
3.4. Nutrient digestibility
The apparent nutrient digestibility values in broiler chickens fed dietary Bacillus protease supplementation are presented in . Crude fat digestibility was the same (p > 0.05). Crude protein digestibility was higher (p < 0.05) for chickens that consumed PROT20 and PROT25. The level of digestibility recorded for crude fibre and dry matter was also higher (p < 0.05) for chickens provided PROT20 and PROT25 diets, but they are statistically the same (p > 0.05) with those that received dietary PROT15. The result showed that crude protein, dry matter, and crude fibre digestibility improved (p < 0.05) as the inclusion rate of Bacillus protease increased.
Table 6. Effects of dietary protease supplementation on apparent digestibility (%) of dry matter, crude protein, crude fat, and crude fibre in broiler finisher diets.
3.5. Intestinal micro examination
and showed the ileum and caecum microbial population changes in birds fed dietary supplemented with Bacillus protease. From , different Bacillus protease levels influenced (p < 0.05) the counts of Lactobacillus, Bifidobacteria, and E.coli found in the ileum section of the small intestine. Lactobacillus and Bifidobacteria count increased (p < 0.05) as the addition of Bacillus protease levels increased. The population of these bacteria (Lactobacillus and Bifidobacteria) was higher (p < 0.05) in the ileum section of the intestine for chickens that consumed the supplemented diets than the controls (PROT0) and PROT10 diet. Birds that consume PROT15, PROT20 and PROT25 recorded decreased (p > 0.05) counts for E.coli compared to the control (PROT0) and PROT10 group. Chickens that consume PROT25 recorded the lowest (p < 0.05) E.coli population in the ileum section of the gut. Meanwhile, represents the microbial population changes in the caecum section of the intestine. The Lactobacillus and Bifidobacteria populations in the caecum were the highest (p < 0.05) for chickens that consumed the PROT25 diet than those that consumed PROT0 (control diet). The lowest (p < 0.05) counts for E. coli at the caecum section of the small intestine were recorded for chickens fed PROT25 compared with those fed with PROT0 diet.
3.6. Carcass traits
shows the carcass traits of chickens that were supplemented with Bacillus protease. The carcass weight, drumstick weight, thigh weight, and breast weight were significantly affected (p < 0.05) by the Bacillus protease inclusion. Conversely, neck, wing, and shank weights were the same (p > 0.05). Carcass and thigh weights were higher (p < 0.05) for chickens provided with PROT25 than PROT0 and PROT10 diets. Breast weight was the highest (p < 0.05) for chickens fed PROT20 or PROT25 compared to other groups. The highest (p < 0.05) drumstick weight was also observed in birds fed PROT25 diets. Carcass yield (dressing percentage), drumstick yield, thigh yield, and breast yield percentage were influenced (p < 0.05) by Bacillus protease supplementation, while the neck, wing, and shank yields were the same (p > 0.05). Birds fed PROT20, and PROT25 recorded the highest (p < 0.05) breast yield percentage. The thigh yield percentage was higher (p < 0.05) for chickens that consumed PROT25 than those fed PROT0, PROT10, and PROT15. PROT25 birds had an improved (p < 0.05) percentage for carcass yield and drumstick yield than those receiving other dietary treatments.
Table 7. The effect of dietary Bacillus protease supplementation on carcass characteristics of broiler birds.
3.7. Meat quality traits
The data for meat quality parameters of broilers fed dietary Bacillus protease is shown in . Only cooking loss (CL) and water holding capacity (WHC) was influenced (p < 0.05) by dietary Bacillus protease among the determined meat quality parameters. The values for CL decreased (p < 0.05) as the inclusion rate of Bacillus protease increased. Cooking loss decreased (p < 0.05) for birds fed PROT15, PROT20 and PROT25 compared to those provided PROT0 and PROT10. WHC was higher (p < 0.05) in birds fed all the dietary treatments except for those that consumed PROT0.
Table 8. The effect of dietary protease supplementation on meat quality attributes of broiler birds.
4. Discussion
4.1. Gut histology
Intestinal morphology involves the display and study of the structure of the small intestine under a microscope. Some of the microscopic structure includes villus height and crypt depth. The villus height and crypt depth are core pointers of gut development, health, and functionality, influencing the digestion and absorption of nutrients (Biasato et al. Citation2018). The results obtained from the present study showed that dietary Bacillus protease at a 2.5 g/kg diet (PROT25) enhanced the growth and development of the gut. Generally, birds fed PROT25 recorded an increased villi height in the duodenum, jejunum, and ileum sections of the gut than the control group. The results of the present study showed that Bacillus protease in caecum could improve the development of the small intestine by reducing the crypt depth of ileum and increasing the villus height of ileum and duodenum (Wexler Citation2007). In the previous studies, Bacillus protease could produce volatile fatty acid (VFAs) through carbohydrate fermentation (Louis and Flint Citation2009), which could maintain gut health and served as the major source of energy for the gut mucosa to promote the growth of gut villus (Louis and Flint Citation2009). According to Vu et al. (Citation2021), Bacillus protease could actually be beneficial for the development of intestinal morphology through the regulation and stabilization of gastrointestinal microbiota, the improvement of the gut morphology and mucosal immunity and the elevation of beneficial bacteria to maintain the overall growth and development of gut morphology in broilers. As protease increases protein digestibility, the amino acids and polypeptides absorbed by the chicken would increase. In this scenario, protease help to create the availability of more amino acids for birds’ utilization. It also influences the production of short-chain fatty acid concentrations (Fan et al. Citation2015; Apajalahti and Vienola Citation2016). The short-chain fatty acids (SCFAs) are the primary products of the breakdown of non-digestible carbohydrates and amino acids by gut bacteria. Collectively, they are a major source of energy for colon cells (Peng et al. Citation2009). SCFAs improve the gut health through a number of local effects, ranging from maintenance of intestinal barrier integrity, mucus production, and protection against inflammation to reduction of the risk of colorectal cancer (Gaudier et al. Citation2009; Lewis et al. Citation2010; O'Keefe Citation2016). The height of the villi is one of the morphological traits, and an increase in the villi height will result in an enhanced absorption ability (Markovic et al. Citation2009; Parviz et al. Citation2018). The small intestinal villi structure mainly utilizes nutrients (Fuller Citation2004; Chwen et al. Citation2013) and the increase in villi height increases nutrient absorption capacity in the gut due to increased absorptive area, expression of brush border enzyme, and nutrient transport system, leading to a higher body weight gain of chicken (Parviz et al. Citation2018). Bacillus protease in PROT25 group may have reduced the intestinal colonization and infections process, hence reducing intestinal mucosa inflammation, which improved the height of villi and function of secretion, digestion, and absorption of nutrients (Khan and Iqbal Citation2016). According to Sobolewska et al. (Citation2017), higher villi height and their increased absorbent surface area translate into improved feed utilization and chicken health. The villi height was lowest for the duodenum, jejunum, and ileum of PROT0 birds, which may be the reason for their poor performance. The condition of the crypt dept explains the state of health and functional status of the chicken intestine. Their sizes determine the rate of the renewal process of intestinal epithelium (Haihan et al. Citation2019; Ivana et al. Citation2019). The proliferation of the intestinal cells takes place, particularly in the crypts (Kaunitz and Akiba Citation2019), and a large deeper crypt shows increased nutrient needs for intestinal care and low efficiency of chicken. Lower (shallow) crypt depth observed in our study for chickens fed Bacillus protease inclusion at 2.5 g/kg diet (PROT25) is a gainful approach to reduce the cost of intestinal care in chickens. According to Xu et al. (Citation2003) and Ivana et al. (Citation2019), the crypt is the villi factory, and a large crypt indicates a faster tissue turnover and an increased request for new tissue. Again, the decreased crypt depth in our study may indicate a proficient tissue turnover, good gut condition, and improved chicken body weight. Moreover, some results showed that the villi and microvilli of intestinal mucosa could affect the production of the digestive enzymes (Wu et al. Citation2013). Our study showed an increase in villi height and villus height: crypt ratio for PROT25 chickens. The improved mucosal morphology was attributed to increased enzymatic bio-activities of gastrointestinal contents. Enhanced intestinal morphology results in better nutrient absorption, decreased secretion in the gastrointestinal tract, increased diseased resistance, and improved overall performance (Ebrahimzadeh et al. Citation2018). The lowest villi height: crypt depth ratio was recorded for chickens fed the control diet (PROT0). According to Parsaie et al. (Citation2007) and Ensari and Marsh (Citation2018), higher villi is related with the efficient absorption capacity of the enterocytes, while short villi decrease the surface area for nutrient absorption. However, the epithelial cells of the villi emanate from the crypt, and a large crypt shows fast enterocyte turnover and increased need for mucosal tissue maintenance requirements (Ayoola et al. Citation2015). The rapid enterocyte production and the epithelial cell renewal rate impact the small intestinal mucosa's protein and energy requirements, reducing the animal's feed efficiency (Luo et al. Citation2009; Liisa et al. Citation2020). Reduced villi height and deepened crypts increased secretion in the GI tract, resulting in birds’ poor growth performance (Parsaie et al. Citation2007; Ivana et al. Citation2019). According to Kim and Khan (Citation2013), the mucus deposit in the small intestine is from goblet cells, which permits gastric secretion and forms a shielding obstruction against mechanical and chemical damages such as ingested feed, microorganisms, and pathogens (Hollingsworth and Swanson Citation2004; Sobolewska et al. Citation2017). Meanwhile, goblet cells are modified epithelial cells that produce mucus on the mucous membrane surface (epithelium layer and an underlying lamina propria of loose connective tissue) of the small intestine. The mucous membrane secretes mucous, a thick protective fluid that prevents pathogens and dirt from entering the body and prevents bodily tissue from becoming dehydrated. Muscularis is a thin layer of smooth muscle that supports mucous and provides it to move and fold. It is responsible for the segmental contractions and peristaltic movement in the GIT. These muscles cause feed to move and mix together with the digestive enzyme down the GIT. In the present study, only the muscularis thickness for the duodenum and ileum was affected by the dietary Bacillus protease. The study of Ebrahimzadeh et al. (Citation2018) contradicts the results of the present study. They reported a decreased muscularis thickness with the inclusion of enzyme fortified grape pomace.
4.2. Growth performance traits
The inclusion of Bacillus protease impacted the BWG, FI, and FCR of broilers at all growth phases (starter phase, finisher phase, and overall phase). Chickens fed with the PROT25 diet had a higher BWG and improved FCR with less FI compared to those provided with the other dietary treatments. Our research findings align with Ghazi et al. (Citation2002) and Cerrate et al. (Citation2019). They attributed a better body weight and feed conversion ratio to improved true metabolizable energy and true nitrogen digestibility. Dietary fibre is implicated in the fast passage rate of the digesta (Wilfarta et al. Citation2007; Sayehban et al. Citation2015; Al-harthi Citation2016), impairing broiler growth. The better performance of birds fed with PROT25 diet in our study may be due to the slow digesta passage rate, which offers maximum opportunity for increased bio-activities of Bacillus protease in the gut. As the slow passage rate of fibre digesta persists in the intestine, it provides greater chances for improved feed digestion and growth performance (Sayehban et al. Citation2016). Dietary whole wheat (high fibre) decreases the bio-activities of amylase in the pancreatic tissue, while the inclusion of protease increases the bio-activities of chymotrypsin and lipase (Engberg et al. Citation2005; Selle et al. Citation2010). The present findings are in line with the study of Dosković et al. (Citation2013), who suggested that exogenous protease complemented endogenous protease to increase intestinal and pancreatic protease production in young birds. Essentially, this phenomenon clearly suggests that the production of endogenous protease may be inadequate in the initial post-hatch period in poultry. Our results are in accordance with that of Odetallah et al. (Citation2003) and Mahmood et al. (Citation2017), who reported a significant increase in BWG with a decreased FI after supplying protease fortified standard protein diet to broilers for 21 days. Similar results (Odetallah et al. Citation2005) recorded an improved BWG and FCR in broilers fed dietary protease (versazyme) in high and normal protein diets. Improved BWG and FCR of chickens fed dietary protease may be attributed to efficient nutrient digestibility and its utilization, mostly when feed ingredients are of inferior/low quality and low bioavailability (Kocher et al. Citation2002; Cowieson and Adeola Citation2008; Gervais et al. Citation2019). According to Freitas et al. (Citation2011), improvement in BWG and FCR with protease supplementation is due to its direct effect in protein digestibility, liberating more amino acids for protein synthesis. Gao et al. (Citation2008) reported that supplementation of protease could change the nutritional and physiological status of the broilers and improve growth rate. The studies of Freitas et al. (Citation2011) and Law et al. (Citation2018) showed a general decrease in FI of chickens fed dietary protease, similar to the results generated in our study. The fact that birds consumed less on a nutrient-dense diet and satisfied their nutrient requirements may explain the decreased FI reported for birds fed PROT25 in the present study. The ingredients of each diet determine whether the animal will consume more or less before satisfying its nutritional requirement (Ani and Oyeagu Citation2012). Several authors also share the same view (Alam et al. Citation2003; Oyeagu et al. Citation2016; Oyeagu et al. Citation2019a). Indeed, Hajati et al. (Citation2009) and Hajati (Citation2010) suggested that exogenous enzyme inclusion may improve broiler performance by improving nutrient digestibility. The reduction of the digesta passage rate in the gut, which activates viscosity reduction is one of the mechanisms of achieving nutrient digestibility (Oyeagu et al. Citation2015). However, Fru-Nji et al. (Citation2011) reported no change on the BWG of broilers fed dietary inclusion of protease at 3% during the finisher phase, which contradicts the present results. Such discrepancies may be due to variations in feed ingredient contents, levels of exogenous enzymes inclusion, or breeds of chicken used (Angel et al. Citation2011).
4.3. Protein and energy utilization efficiency
Bacillus protease supplementation increased energy and protein utilization efficiency with less protein and energy intake for birds fed with the PROT25 diet throughout the feeding period. The efficient utilization of energy and protein recorded for PROT25 birds may be the reason for their improved BWG and FCR (), as supported by Kamran et al. (Citation2008) findings. This study, however, contradicts the findings of Moosavi et al. (Citation2012), who recorded no effect of dietary protease on both PER and EER of broiler chickens. According to Olukosi et al. (Citation2015), NSPase and protease inclusion improved the metabolizable energy. It is important to note that different ingredients of broiler diets and the differences within them affect nutrient digestibility (Jha and Mishra Citation2021; Tejeda and Kim Citation2021). For instance, protein digestibility is high in corn-soybean meal-based broiler diets but lower in animal-derived meals. The disparity may result from excessive thermal processing and the type of contained animal by-products (Douglas et al. Citation2000; Goldflus et al. Citation2006; de Coca-Sinova et al. Citation2008; Oyeagu et al. Citation2016). Variation in protein and energy digestibility could be observed within the same feed ingredient (Lemme et al. Citation2004; Rojas and Stein Citation2017). Considering the diet composition used in this study, birds fed with the PROT25 diet showed improved BWG () due to efficient utilization of protein and energy. Also, it may be that the level of Bacillus protease in PROT25 diet improved protein availability as it broke down protein–starch bonds within feed ingredients (Selle et al. Citation2013). The effective separation of protein–starch bond due to protease inclusion increases digestion and facilitates the utilization of extra energy by the animal (du Plessis and van Rensburg Citation2014). The diets contain amino acids complexes with reducing sugars that could potentially make them impossible to digest. The possibility of beneficial impact of exogenous protease in liberating amino acids from reducing complex sugars cannot be ignored. It has been suggested that exogenous protease acts by releasing peptides from anti-nutritional factors present in the feed ingredients, cleaving linkage between amino acids-starch complexes, supplementing the endogenous production of peptidases, and reducing enzymatic secretions and protein turnover; thereby providing amino acids for protein synthesis and deposition (Cowieson and Roos Citation2013). It was reported that age of a bird and diet are known to influence pancreatic output and enzyme composition (Isaksen et al. Citation2010). Although more research is needed, in line with our results, there is evidence that the addition of exogenous protease to the diet increases pancreatic production and secretion of endogenous proteolytic enzymes (Jeferson et al. Citation2020), an effect that result in higher protein digestibility (Yuan et al. Citation2017). It is important to note that amino acid deficiency is known to reduce growth rate and feed efficiency in broilers (Freitas et al. Citation2011). The better performance of birds fed the highest Bacillus protease inclusion level (PROT25) than other groups in our study may be due to the maximum pancreatic enzyme secretion that increased protein digestibility. According to Lemme et al. (Citation2004) and Freitas et al. (Citation2011), significant amounts of protein and energy escape via the GI tract without being completely digested; thus, the addition of exogenous protease optimized feed protein and energy digestibility and utilization (Mohammadigheisar and Kim Citation2018; Ndazigaruye et al. Citation2019). Dietary protease enhanced BWG and the efficiency of feed digestion by improving amino acid content and energy utilization (Kaczmarek et al. Citation2014). It also reduces proteolytic fermentation, bacterial toxins, and the number of nutrients excreted in faeces, reducing potential pollutants in the environment (Mahmood et al. Citation2017). Improved digestion, utilization, and bio-availability of amino acids were reported when protease cleaved trypsin inhibitor (anti-nutrient) (Ndazigaruye et al. Citation2019). Better growth performance reported for broilers fed PROT25 may result from increased protein digestibility and capacity for energy utilization. Also, it may be due to alterations in intestinal morphological traits (Cowieson, Lu, et al. Citation2017) or other gut health indicators, such as volatile fatty acids (VFA) and secretory immunoglobulin A (IgA).
4.4. Apparent nutrient digestibility
Improved feed efficiency is of vital importance in animal nutrition because it reduces environmental pollution from poultry and production costs. Poultry cannot digest certain compounds in the feedstuffs, and these compounds may interfere with the animal's digestive system (Rada et al. Citation2016). These problems were made evident or noticed because animals cannot endogenously produce the necessary enzymes to degrade these compounds (Khattak et al. Citation2006), restricting the full release of nutrients to the animal. However, Bacillus protease was used in this study to reduce the total nutrient excretion, which results from incomplete digestion. In the present study, Bacillus protease inclusion improved the digestibility of crude fibre, crude protein, and dry matter but not crude fat. Improved nutrient digestibility traits enhance growth performance, reduce nitrogen excretion, and improve overall gut health due to reduced putrefaction in the distal intestine (Walk et al. Citation2019). The higher nutrient digestibility values recorded in this study for birds fed PROT25 may be due to the positive effects of the gastrointestinal tract fermentation by lowering gastric acids, decreasing the pathogenic microbial activity, and enhancing mucosal architecture or structure (Scott Citation2002; Akinola et al. Citation2015). Furthermore, fermentation results to the catabolism of feed constituents, reducing the viscous nature of grain-legumes, synthesizing B-vitamins, and increasing mineral extractability (Badau et al. Citation2005). According to Angel et al. (Citation2011); and Liu et al. (Citation2013), the inclusion of protease in poultry diets improved amino acid digestibility. They reported beneficial impacts of exogenous protease on amino acid and crude protein digestibility which might be attributed to their capacity to target protease inhibitors and/or target the cereal portion of the diet (Liu et al. Citation2013). It has been suggested that protease supplementation may help neutralize anti-nutritional factors such as antigenic proteins, trypsin inhibitors, and lectins; improving protein digestibility (Douglas et al. Citation2000). Previous studies (Cowieson and Ravindran Citation2008; Amerah et al. Citation2017) also found that supplementary protease improved protein digestibility and nitrogen retention. Nevertheless, Campasino et al. (Citation2015) reported that protease supplementation did not improve protein digestibility at 10% inclusion level. Surprisingly, Crude Protein digestibility was improved at 15% protease inclusion level. According to Kim et al. (Citation2020) Supplementation of protease has been employed in diets to reduce crude protein levels in order to minimize dietary protein waste, nitrogen excretion, and nitrogen emission into the environment without compromising bird performance. In the past, (Olukosi et al. Citation2015; Opoku et al. Citation2015; Hussain et al. Citation2019) protease addition has led to improved nitrogen digestibility when an alternate raw material with more indigestible fraction was added. Walk et al. (Citation2019) and Brown et al. (Citation2020) recorded improvements on BWG and FCR of chicken and attributed the results to improved digestibility of protein caused by dietary protease. It improved the crude protein and amino acid digestibility (Cowieson and Roos Citation2013) and enhanced the ileal digestibility of energy (Kalmendal and Tauson Citation2012). According to Cowieson, Zaefarian, et al. (Citation2017), a beneficial effect of exogenous protease on mucin secretion, intestinal integrity, and immunity was recorded. Freitas et al. (Citation2011) and Akinola et al. (Citation2015) found a significant effect of protease supplementation only in the dry matter, while Kamel et al. (Citation2015) and Zuo et al. (Citation2015) recorded an improved crude protein digestibility. This study showed that Bacillus protease could increase dry matter, crude fibre, and crude protein digestibility, thus affecting growth performance (). Dietary protease increased the digestion of many essential amino acids in chicken (Allouche et al. Citation2015; Kamel et al. Citation2015).
4.5. Gut micro examination
There is a synergy between gut micro-flora that colonized the gastrointestinal tract and their host at the early post-hatch period. The gut micro-flora performs an essential task in the health and nutrition of the host by encouraging digestion and absorption of nutrients, preventing the migration of the pathogen, and sustaining normal mucosal immunity. The significant traits to explain the microbial structure and diversity are the richness and evenness of the bacteria. According to Kiarie et al. (Citation2014) and Leeming et al. (Citation2019), there is a general acceptance that diet manipulation can influence the microbial structure and diversity of the intestine. Exogenous enzymes can control the population of microbes due to the changes they impose in the gut content (Hubner et al. Citation2002; Masey O'Neill et al. Citation2014; Oyeagu et al. Citation2019b). The changes recorded in the present study for bacteria populations in the ileum and caecum sections of the gut are not consistent with the report of Gao et al. (Citation2008), who recorded no effect for Lactobacillus and Coliform counts in the caecum of 21d old birds. Luo et al. (Citation2009) reported no difference in the population of E. coli, Lactobacillus, and total aerobes in the ileum and caecum of chicken at 42d of the feeding trial. There were increased Lactobacillus and Bifidobacteria counts in both ileum and caecum parts of the gut as the level of Bacillus protease increased. Also, there was a drop/decrease in E. coli counts as the level of Bacillus protease increased in both the ileum and caecum parts of the intestine. These observations may be due to the ability of the enzyme to increase the levels of available substrate for microbial fermentation, which improves the digestibility of protein and the production of short-chain fatty acid (SCFA) in the GI tract. This process typically occurs due to the non-digested protein diet available to the intestinal microbes (Morrison and Preston Citation2016). A similar observation was reported by Nabizadeh et al. (Citation2017). Dietary Bacillus protease influenced the proliferation of Lactobacillus and Bifidobacteria populations in the present study. These beneficial bacteria species are found throughout the digestive tract, predominantly in the small intestine. They are thought to contribute to nutrient absorption and are involved with bile salt hydrolysis (Xiao et al. Citation2017). Lactobacillus and Bifidobacteria are gram-positive and facultative anaerobic, and are fastidious with complex nutritional requirements, including fermentable carbohydrates, amino acids, peptides, vitamins, salts, and fatty acids (Staley et al. Citation2017). Apajalahti and Vienola (Citation2016) hypothesized that protease would decrease Lactobacilli located in the small intestine; however, our data do not support this hypothesis, as Bacillus protease used in the present study increased the proliferation of Lactobacillus and Bifidobacteria in both caecum and ileum sections of the gut. Jozefiak et al. (Citation2010) and Yadav and Jha (Citation2019) reported that external protease reduced the viscosity in the intestine; it also helps developing competitive microbial communities and even higher intra-microbial competition. It diminishes microbial interference in nutrient absorption and contributes to the potential reduction of pathogenic microbes with improved growth traits. Dietary factors can influence the bacteria population. Some authors (Sugiharto and Ranjitkar Citation2019) reported a higher population of E. coli and Lactobacillus in the digesta of broilers that consumed wheat and barley than those that consumed corn diets. As the intestinal bacteria of chicken changes, it may alter the mucosal structure, which may subsequently influence the absorptive capacity of the nutrient (Munyaka et al. Citation2016). An increase in the SCFA density stimulates a gradual reduction of the proliferation rate of Enterobacteria but not that of Lactobacillus and Bifidobacteria (Van der Wielen et al. Citation2000; Nabizadeh et al. Citation2017). Inclusion of Bacillus protease influenced the production of SCFAs which are end products of bacterial fermentation of carbohydrates and amino acids (Jeferson et al. Citation2020). The SCFAs encourage the proliferation of beneficial microbes (such as Lactobacillus) and prevent the inflammation of the gut (Morrison and Preston Citation2016). The majority of short-chain fatty acids are produced by the fermentation of carbohydrates and amino acids that escaped digestion in the small intestine. Some gastrointestinal microbes ferments amino acids and can deaminate them rapidly (Lee et al. Citation2018). Exogenous enzymes balance chicken intestinal bacteria, which may affect the host's health status and the extent of digestion (Bedford and Cowieson Citation2012; Alagawany et al. Citation2018; Sudhir and Rajesh Citation2019).
4.6. Carcass traits
Our study confirms that the Bacillus protease significantly affects the carcass, drumstick, thigh, and breast weights and carcass yield (dressing percentage), drumstick yield, thigh yield, and breast yield of broiler chickens. Improved carcass yield is as a result of adequate diet and chicken nutrition (Cardoso et al. Citation2011). For example, livestock will deposit more tissue (muscle) when they consume balanced diets. Dietary Bacillus protease at 2.5 g/kg offers improved nutrient digestibility and increases nutrients utilization for enhanced development of the muscle (tissue) and breast growth since breast cut yield accounts for approximately 40% of aggregate carcass yield (Oyeagu et al. Citation2019b). The addition of protease to feed improved poultry growth rates, carcass yield and caused some changes in the diversity of the broiler gut micro-biota. The presence of protease in the feed improved protein absorption, which increased the live body weight and cut yield of broiler chickens by 0.5 kg, and also reduced feed intake (Daria et al. Citation2020). Comparable to our results, Dalólio et al. (Citation2015) confirmed the impact of dietary enzyme complexes on the carcass yield of chicken. According to Marapana (Citation2016), dressing percentage and meat yield of different chicken body parts could be altered by diets. The increased yield of carcass, drumstick, thigh and breast meat of broilers fed with the PROT25 diet compared to other dietary treatments in our study may be due to the increased available nutrients for the development of tissue (retail cut yields). Dietary exogenous enzymes enhanced diet digestibility, substrates availability, and increased absorption of nutrients, which positively impacted muscle development (Oyeagu et al. Citation2019b). Dietary external enzymes such as amylase, xylanase, or protease boost the actions of the pancreatic and intestinal enzymes determined at 14 and 42-d-old chickens (Horvatovic et al. Citation2015). Intestinal enzymes play a crucial role in breaking down feeds, while pancreatic enzymes break down sugars, fats, and starches. These proteins speed up chemical reactions that turn nutrients into available substances for absorption. Dietary barley, maize, sorghum, and wheat without enzymes negatively impact intestinal enzyme activities, unlike enzyme supplemented diets (Shakouri et al. Citation2008). According to Yadav and Sah (Citation2005) and Barletta (Citation2011), microbial protease could break down stored proteins and proteinaceous anti-nutrients in diets. It was evident from the slaughter weight, carcass weight, and carcass yield (dressing percentage) that chickens with heavier muscle have a higher dressing percent and higher cut-yield than lighter chickens (). According to Wilfarta et al. (Citation2007) and Sayehban et al. (Citation2015), dietary fibre was implicated in the fast passage rate of the digesta which impairs broiler growth. The better performance of birds fed with the PROT25 diet in our study may be due to the slow digesta passage rate, which offers maximum opportunity for increased bio-activities of Bacillus protease in the gut. As the slow passage rate of fibre digesta persists in the intestine, it provides greater chances for improved feed digestion and absorption with higher muscle deposit which influences the cut yield percentage (Sayehban et al. Citation2016). It was reported that broilers with higher muscle deposit produces higher cut-yield percentage (Al-harthi Citation2016; Oyeagu et al. Citation2019b). The range of dressing percentage (77.19% to 82.24%) recorded in the present study is above the threshold reported by Schweihofer (Citation2011). They noted that the average dressing percentage (carcass yield) of broiler chicken is 71%. Breast meat yield is one of the carcass components with the highest economic value. Breast meat continuously increases as a percentage of body weight in our study. A similar result was reported by Petracci et al. (Citation2004) and Kanagaraju et al. (Citation2019). The success of poultry meat production may be attributed to improvements in growth and carcass yield, mainly by increasing the proportion of chicken breast part. Some chicken parts such as the thigh, drumstick, and breast have higher commercial value (Marapana Citation2016). The sale of a whole chicken and its cut-up parts allows the consumers to have different cooking and taste choices (Cevger et al. Citation2004; Kaygisiz and Cevger Citation2010). The demand for high-quality cut-up parts at meat shelves in the shops has increased, and it has also influenced the poultry industry to change its marketing strategies. Today, the most marketed form of chicken is always the cut-up parts. However, the yield of breasts, thighs, and broiler drumsticks has become critical to processors (Young et al. Citation2001). Due to increased aggregate value, there is a higher tendency to sell cut-up parts more than the whole carcass. Silveira et al. (Citation2010) reported a 25% leg quarter yield increase for birds fed dietary protease at 21 days of age than those fed the control diet. The authors reported no effects on breast meat yield. According to Cardoso et al. (Citation2011), there was no difference in carcass yield at day 42 of chickens fed dietary multi-enzyme. Our results are similar to the findings of Alam et al. (Citation2003), Wang et al. (Citation2005), and Hajati (Citation2010). They reported an increased carcass and breast yield of birds-fed dietary enzymes (xylanase + protease, phytase + protease, and xylanase + protease + amylase) due to improved digestion, higher nutrient availability, and absorption.
4.7. Meat quality traits
Chicken meat has specific sensory attributes that increase consumers’ acceptance, such as appearance, texture, succulence, and flavour (Petracci and Baéza Citation2011). The most important factor affecting consumer choice is broiler meat colour (Werner et al. Citation2009). Our results showed no significant change in the chicken meat colour. Meat quality traits depend directly on environmental and management conditions, mainly the feed offered (Neves et al. Citation2014). Dietary enzymes influence efficient digestibility and promote muscle fibre deposit and whole carcass yield (Allouche et al. Citation2015). Increased protein deposition may adjust muscle fibre type and shape, altering meat quality parameters (Fatufe et al. Citation2004; Dalólio et al. Citation2016). Our study showed that the inclusion of Bacillus protease increased the values of WHC and decreased the CL of the breast meat. WHC concerns the ability of the meat to retain its water upon application of an external force (Omojola et al. Citation2014), and it is a primary indicator of the juiciness of the meat. Dietary Bacillus protease reduced CL which implies reduced water loss during cooking. Omojola et al. (Citation2014) reported that meat with increased water loss (exudate) results in more nutrient loss and should be considered poor-quality meat. Dietary Bacillus protease used in our study reduced nutrient loss while also increasing the cooking yield of chicken. This is attributed to the specific hydrolyzing activities of Bacillus protease (from Bacillus strain EA1). It may be that microbial protease (modified or wild) from non-pathogenic microbes improved meat quality (Alaa et al. Citation2014). On the contrary, the traditional plant protease has broad specificities and breaks down connective tissue and myofibrillar proteins indiscriminately (Ashie et al. Citation2002; Madhusankha and Thilakarathna Citation2021). It leads to undesirable attributes in the tenderized meat (i. e., mushy texture, high water loss, bitterness, and off-flavour). The findings of our trial are in contrast to the studies of Werner et al. (Citation2009), Zakaria et al. (Citation2010), and Dalólio et al. (Citation2015); they did not observe any effect of exogenous enzyme addition on meat quality parameters such as pH, WHC, colour, CL, shear force among others. According to Omojola et al. (Citation2014), the cooking yield of meat is dependent on cooking loss, while decreased cooking loss results to higher WHC. The present study showed that supplementing Bacillus protease at a 2.5 g/kg diet improved performance compared to other treatment groups.
The dietary level (2.5 g PROT/kg feed) confirms the maximum/highest activities of Bacillus protease on the substrate, reflecting the efficient utilization of protein and energy and the overall performance of chickens. Since the peak growth performance of broiler birds was observed at the highest level of Bacillus protease supplementation (2.5 g PROT/kg feed), further study is required to evaluate the effects of Bacillus protease on the performance of broilers beyond 2.5 g PROT/kg feed (PROT25) inclusion. Nevertheless, our findings revealed that the inclusion of Bacillus protease in maize-soybean meal-based diets improved the health status, encouraged the development of gut histology, and provided an excellent gut microbiota environment for chickens (Khan and Naz Citation2013). We concluded that broiler chickens respond positively to supplementation with Bacillus protease concerning meat quality traits. The gut microflora and intestinal histological characteristics were improved with the highest inclusion of Bacillus protease (Abudabos et al. Citation2017). In other words, the highest inclusion of Bacillus protease improved nutrient digestion and utilization as it protects the gut's integrity, which helps stabilize the health status of chickens. Summarily, birds fed 2.5 g Bacillus protease/kg feed (PROT25) produced a better FCR and higher weight gain, retail cut yields, carcass yields, and improved gut integrity.
Acknowledegments
The authors acknowledge Late Prof Voster Muchenje for his support. The authors wish to appreciate the Cape Peninsula University of Technology, Department of Agriculture Wellington Campus, Cape Town, South Africa, for their support.
Disclosure statement
No potential conflict of interest was reported by the author(s).
References
- Abudabos AM, Alyemni AH, Dafalla YM, Rifat UK. 2017. Effect of organic acid blend and Bacillus subtilis alone or in combination on growth traits, blood biochemical and antioxidant status in broilers exposed to Salmonella typhimurium challenge during the starter phase. J Appl Anim Res. 45(1):538–542. doi:10.1080/09712119.2016.1219665.
- Adedokun SA, Olojede OC. 2019. Optimizing gastrointestinal integrity in poultry: the role of nutrients and feed additives. Front Vet Sci. 5:348. doi:10.3389/fvets.2018.00348.
- Ahmed AS, Mustafa MD, Nemat AB, Tarek AE, Khairy AA, Mahmoud MA. 2020. Effect of supplemental serine-protease from Bacillus licheniformis on growth performance and physiological change of broiler chickens. J Appl Anim Res. 48:86–92. doi:10.1080/09712119.2020.1732986.
- Akinola OS, Onakomaiya AO, Agunbiade JA, Oso AO. 2015. Growth performance, apparent nutrient digestibility, intestinal morphology and carcass traits of broiler chickens fed dry, wet and fermented-wet feed. Livest Sci. 177:103–109. doi:10.1016/j.livsci.2015.04.016.
- Alaa AB, David LH, Geert G, Adnan AB, Philip F. 2014. Exogenous proteases for meat tenderization. Crit Rev Food Sci Nutr. 54:1012–1031. doi:10.1080/10408398.2011.623247.
- Alagawany M, Elnesr SS, Farag MR. 2018. The role of exogenous enzymes in promoting growth and improving nutrient digestibility in poultry. Iran J Vet Res. 19:157–164.
- Alam MJ, Howlider MAR, Pramanik MAH, Haque MA. 2003. Effect of exogenous enzyme in diet on broiler performance. Int J Poult Sci. 2:168–173. doi:10.3923/ijps.2003.168.173.
- Al-harthi MA. 2016. The efficacy of using olive cake as a by-product in broiler feeding with or without yeast. Ital J Anim Sci. 15:512–520. doi:10.1080/1828051X.2016.1194173.
- Allouche L, Madani T, Ait Hamouda Z, Boucherit MR, Taleb H, Samah O, Rahmani K, Touabti A. 2015. Effect of addition of exogenous enzymes in hypocaloric diet in broiler chicken on performance, biochemical parameters and meat characteristics. Biotechnol Anim Husb. 31:551–565. doi:10.2298/BAH1504551A.
- Amerah AM, Romero LF, Awati A, Ravindran V. 2017. Effect of exogenous xylanase, amylase, and protease as single or combined activities on nutrient digestibility and growth performance of broilers fed corn/soy diets. Poult Sci. 96:807–816. doi:10.3382/ps/pew297.
- Angel CR, Saylor W, Vieira SL, Ward N. 2011. Effects of a monocomponent protease on performance and protein utilization in 7- to 22-day-old broiler chickens. Poult Sci. 90:2281–2286. doi:10.3382/ps.2011-01482.
- Ani AO, Oyeagu CE. 2012. Response of shaver brown hens to feeds of different sources in the humid tropical environment. Agro-Sci J Trop Agric Food Environ Ext. 11(3):12–23. doi:10.4314/as.v11i3.2.
- [AOAC] Association of Official Analytical Chemists. 2006. Official methods of analysis. 18th ed. Washington (DC): AOAC. 2263.
- Apajalahti J, Vienola K. 2016. Interaction between chicken intestinal microbiota and protein digestion. Anim Feed Sci Technol. 221:323–330. doi:10.1016/j.anifeedsci.2016.05.004.
- Ashie INA, Sorensen TL, Nielsen PM. 2002. Effects of papain and a microbial enzyme on meat proteins and beef tenderness. J Food Sci. 67:2138–2142. doi:10.1111/j.1365-2621.2002.tb09516.x.
- Ayoola AA, Malheiros RD, Grimes JL, Ferket PR. 2015. Effect of dietary exogenous enzyme supplementation on enteric mucosal morphological development and adherent mucin thickness in Turkeys. Front Vet Sci. 2:45. doi:10.3389/fvets.2015.00045.
- Badau MH, Nkama I, Jideani AI. 2005. Phytic acid content and hydrochloric acid extractability of minerals in pearl millet as affected by germination time and cultivar. J Food Chem. 92:425–435. doi:10.1016/j.foodchem.2004.08.006.
- Barletta A. 2011. Introduction: current market and expected developments. In: Bedford MR, Partridge GG, editors. Enzymes in farm animal nutrition. Wallingford: CABI; p. 1–11. doi:10.1079/9781845936747.0001.
- Bedford MR, Cowieson AJ. 2012. Exogenous enzymes and their effects on intestinal microbiology. Anim Feed Sci Technol. 173:76–85. doi:10.1016/j.anifeedsci.2011.12.018.
- Biasato I, Ferrocino I, Biasibetti E, Grego E, Dabbou S, Sereno A, Gai F, Gasco L, Schiavone A, Cocolin L, Capucchio MT. 2018. Modulation of intestinal microbiota, morphology and mucin composition by dietary insect meal inclusion in free-range chickens. BMC Vet Res. 14:383. doi:10.1186/s12917-018-1690-y.
- Brown AT, Cantley S, Gutierrez O, Lemons ME, Wamsley KGS. 2020. Effects of varying diet nutrient density and enzyme inclusion strategy for Ross 708 male broilers under a natural disease challenge. J Appl Poult Res. 29(4):947–976. doi:10.1016/j.japr.2020.09.005.
- Buyse J, Jassens K, van der Geyten S, van As P, Decuypere E, Darras VM. 2002. Pre-and postprandial changes in plasma hormone and metabolite levels and hepatic deiodinase activities in meal-fed broiler chickens. Br J Nutr. 88:641–653. doi:10.1079/BJN2002741.
- Campasino A, Williams M, Latham R, Bailey CA, Brown B, Lee JT. 2015. Effect of increasing dried distillers’ with solubles and non-starch polysaccharide degrading enzyme inclusion on growth performance and energy digestibility in broilers. J Appl Poult Res. 24:135–144. doi:10.3382/japr/pfv018.
- Cardoso DM, Maciel MP, Passos DP, Silva FV, Reis ST, Aiura FS. 2011. Efeito do uso de complexo enzimático em rações para frangos de corte. Arch Zootec Córdoba. 60:1053–1064. doi:10.4321/S0004-05922011000400021.
- Cerrate S, Ekmay R, England JA, Coon C. 2019. Predicting nutrient digestibility and energy value for broilers. Poult Sci. 98:3994–4007. doi:10.3382/ps/pez142.
- Cevger Y, Savafl S, Hakan G. 2004. The effect of the sale of whole or cut up chicken meat on enterprise income according to season. Turk J Vet Anim Sci. 28:399–402. https://citeseerx.ist.psu.edu/viewdoc/download?doi=10.1.1.824.8266&rep=rep1&type=pdf.
- Chwen LT, Hooi LF, Nguyen TT, Choe DW. 2013. Growth performance, plasma fatty acids, villous height and crypt depth of preweaning piglets fed with medium chain triacylglycerol. Asian Australas J Anim Sci. 26:700–704. doi:10.5713/ajas.2012.12561.
- Cowieson A, Lu H, Ajuwon K, Knap I, Adeola O. 2017. Interactive effects of dietary protein source and exogenous protease on growth performance, immune competence and jejunal health of broiler chickens. Anim Prod Sci. 57(2):252–261. doi:10.1071/AN15523.
- Cowieson AJ. 2010. Strategic selection of exogenous enzymes for corn/soy-based poultry diets. J Poult Sci. 47:1–7. doi:10.2141/jpsa.009045.
- Cowieson AJ, Adeola O. 2008. Carbohydrases, protease, and phytase have an additive beneficial effect in nutritionally marginal diets for broiler chicks. Poult Sci. 87:1860–1867. doi:10.1093/ps/84.12.1860.
- Cowieson AJ, Ravindran V. 2008. Effect of exogenous enzymes in corn-based diets varying in nutrient density for young broilers: growth performance and digestibility of energy, minerals and amino acids. Br Poult Sci. 49:37–44. doi:10.1080/00071660701812989.
- Cowieson AJ, Roos FF. 2013. Bio-efficacy of a mono-component protease in the diets of pigs and poultry: a meta-analysis of effect on ileal amino acid digestibility. J Appl Anim Nutr. 2:1–8. doi:10.1017/jan.2014.5.
- Cowieson AJ, Zaefarian F, Knap I, Ravindran V. 2017. Interactive effects of dietary protein concentration, a mono-component exogenous protease and ascorbic acid on broiler performance, nutritional status and gut health. Anim Prod Sci. 57(2):1058–1068. doi:10.1071/AN15740.
- Dalólio FS, Moreira J, Vaz DP, Albino LFT, Valadares LR, Pires AV, Pinheiro SRF. 2016. Exogenous enzymes in diets for broilers. Rev Braz Saúde Prod Anim Salvador. 17:149–161. doi:10.1590/S1519-99402016000200003.
- Dalólio FS, Vaz DP, Moreira J, Albino LFT, Valadares LR. 2015. Carcass characteristics of broilers fed enzyme complex. Biotechnol Anim Husb. 31:153–162. doi:10.2298/BAH1502153D.
- Daria P, Anastasia K, Natalya R, Ayslu M, Margarita S. 2020. Effect of Bacillus pumilus 3-19 protease on growth parameters and gut microbiome of broiler chickens. E3S Web Conf. 222. doi:10.1051/e3sconf/202022202051.
- de Carvalho NM, Oliveira DL, Saleh MAD, Pintado ME, Madureira AR. 2021. Importance of gastrointestinal in vitro models for the poultry industry and feed formulations. Anim Feed Sci Technol. 271:114730. doi:10.1016/j.anifeedsci.2020.114730.
- de Coca-Sinova A, Valencia DG, JiménezMoreno E, Lázaro R, Mateos GG. 2008. Apparent ileal digestibility of energy, nitrogen, and amino acids of soybean meals of different origin in broilers. Poult Sci. 87:2613–2623. doi:10.3382/ps.2008-00182.
- Delezie E, Swennen Q, Buyse J, Decuypere E. 2007. The effect of feed withdrawal and crating density in transit on metabolism and meat quality of broilers at slaughter weight. Poult Sci. 86:1414–1423. doi:10.1093/ps/86.7.1414.
- Dessimoni GV, Dalólio FS, Moreira J, Teixeira LV, Bertechini AG, Hermes RG. 2019. Protease supplementation under amino acid reduction in diets formulated with different nutritional requirements for broilers. Braz J Poult Sci. 21:1–8. doi:10.1590/1806-9061-2017-0707.
- Dosković V, Bogosavljević-Bosković S, Pavlovski Z, Milošević B, Škrbić Z, Rakonjac S. 2013. Enzymes in broiler diets with special reference to protease. World’s Poult Sci J. 69:343–360. doi:10.1017/S0043933913000342.
- Douglas MW, Parsons CM, Bedford MR. 2000. Effect of various soybean meal sources and Avizyme on chick growth performance and ileal digestible energy. J Appl Poult Res. 9:74–80. doi:10.1093/japr/9.1.74.
- du Plessis RE, van Rensburg CJ. 2014. Carbohydrase and protease supplementation increased performance of broilers fed maize-soybean-based diets with restricted metabolizable energy content. S Afr J Anim Sci. 44:262–270. doi:10.4314/sajas.v44i3.8.
- Ebrahimzadeh SKB, Farhoomand NP, Aghjehgheshlagh FM. 2018. Effects of exogenous tannase enzyme on growth performance, antioxidant status, immune response, gut morphology and intestinal microflora of chicks fed grape pomace. S Afr J Anim Sci. 48:1–18. doi:10.4314/sajas.v48i1.2.
- Engberg RM, Hedemann MS, Steenfeldt S, Jensen BB. 2005. Influence of whole wheat and xylanase on broiler performance and microbial composition and activity in the digestive tract. Poult Sci. 83:925–938. doi:10.1093/ps/83.6.925.
- Ensari A, Marsh MN. 2018. Exploring the villus. Gastroenterol Hepatol Bed Bench. 11:181–190.
- Erdaw MM, Perez-Maldonado RA, Iji PA. 2019. Protease and phytase supplementation of broiler diets in which soybean meal is partially or completely replaced by raw full-fat soybean. S Afr J Anim Sci. 49:455–467. doi:10.4314/sajas.v49i3.6.
- Fan P, Li L, Rezaei A, Eslamfam S, Che D, Ma X. 2015. Metabolites of dietary protein and peptides by intestinal microbes and their impacts on gut. Curr Protein Pept Sci. 16:646–654. doi:10.2174/1389203716666150630133657.
- Fatufe AA, Timmler R, Rodehutscord M. 2004. Response to lysine intake in composition of body weight gain and efficiency of lysine utilization of growing male chickens from two genotypes. Poult Sci. 83:1314–1324. doi:10.1093/ps/83.8.1314.
- Freitas DM, Vieira SL, Angel CR, Favero A, Maiorka A. 2011. Performance and nutrient utilization of broilers fed diets supplemented with a novel mono component protease. J Appl Poult Res. 20:347–352. doi:10.3382/japr.2010-00295.
- Fru-Nji F, Kluenter AM, Fischer M, Pontoppidan K. 2011. A feed serine protease improves broiler performance and energy digestibility. J Poult Sci. 48:239–246. doi:10.2141/jpsa.011035.
- Fuller MF. 2004. The encyclopedia of farm animal nutrition. Wallingford, UK: CAB International Publishing. 10.1079/9780851993690.0000
- Gao F, Jiang Y, Zhou GH, Han ZK. 2008. The effects of xylanase supplementation on performance, characteristics of the gastrointestinal tract, blood parameters and gut microflora in broilers fed on wheat-based diets. Anim Feed Sci Technol. 142:173–184. doi:10.1016/j.anifeedsci.2007.07.008.
- Gaudier E, Rival M, Buisine MP, Robineau I, Hoebler C, Hoebler C. 2009. Butyrate enemas upregulate muc genes expression but decrease adherent mucus thickness in mice colon. Physiol Res. 58:111–119. doi:10.33549/physiolres.931271.
- Gervais N, Da-Hye K, Chang-Won K, Kyung-Rae K, Yong-Jin J, Sang-Rak L, Kyung-Woo L. 2019. Effects of low-protein diets and exogenous protease on growth performance, carcass traits, intestinal morphology, cecal volatile fatty acids and serum parameters in broilers. Animals. 9:226. doi:10.3390/ani9050226.
- Ghazi S, Rooke JA, Galbraith H, Bedford MR. 2002. The potential for the improvement of the nutritive value of soya-bean meal by different proteases in broiler chicks and broiler cockerels. Br Poult Sci. 43:70–77. doi:10.1080/00071660120109935.
- Goldflus F, Ceccantini M, Santos W. 2006. Amino acid content of soybean samples collected in different Brazilian states – harvest 2003/2004. Braz J Poult Sci. 8:105–111. doi:10.1590/S1516-635X2006000200006.
- Gopinger E, Xavier EG, Lemes JS, Moraes PO, Elias MC, Roll VFB. 2014. Carcass yield and meat quality in broilers fed with canola meal. Br Poult Sci. 55:817–823. doi:10.1080/00071668.2014.980394.
- Haihan Z, Dongfeng L, Lingbin L, Ling X, Mo Z, Xi H, Yang L. 2019. Cellular composition and differentiation signaling in chicken small intestinal epithelium. Animals. 9:870. doi:10.3390/ani9110870.
- Hajati H. 2010. Effects of enzyme supplementation on performance, carcass characteristics, carcass composition and some blood parameters of broiler chicken. Am J Anim Vet Sci. 5:221–227. doi:10.3844/ajavsp.2010.221.227.
- Hajati H, Rezaei M, Sayyahzadeh H. 2009. The effects of enzyme supplementation on performance, carcass characteristics and some blood parameters of broilers fed on corn-soybean meal-wheat diets. Int J Poult Sci. 8:1199–1205. doi:10.3923/ijps.2009.1199.1205.
- Hollingsworth MA, Swanson BJ. 2004. Mucins in cancer: protection and control of the cell surface. Nat Rev Cancer. 4:45–60.
- Horvatovic MP, Glamocic D, Zikic D, Hadnadjev TD. 2015. Performance and some intestinal functions of broilers fed diets with different inclusion levels of sunflower meal and supplemented or not with enzymes. Braz J Poult Sci. 17:25–30. doi:10.1590/1516-635x170125-30.
- Hubner K, Vahjen W, Simon O. 2002. Bacterial responses to different dietary cereal type and xylanase supplementation in the intestine of broiler chicken. Arch Anim Nutr. 56:167–187. doi:10.1080/00039420214191.
- Hussain M, Mirza MA, Nawaz H, Asghar M, Ahmed G. 2019. Effect of exogenous protease, Mannanase and xylanase supplementation in corn and Hi protein corn DDGS based diets on growth performance, intestinal morphology and nutrients digestibility in broiler chickens. Braz J Poult Sci. 21:1–10. doi:10.1590/1806-9061-2019-1088.
- Isaksen MF, Cowieson AJ, Kragh KM. 2010. Starch- and protein-degrading enzymes: biochemistry, enzymology and characteristics relevant to animal feed use. In: Bedford MR, Partridge GG, editors. Enzymes in farm animal nutrition. 2nd ed. Oxfordshire: CAB International; p. 85–95.
- Ivana P, Maja M, Mirela P, Ksenija M, Valerija B, Ivan M, Matija D. 2019. Intestinal morphology in broiler chickens supplemented with propolis and bee pollen. Animals. 9:301. doi:10.3390/ani9060301.
- Jeferson ML, Claire NS, Eliza JL, Robert DC, Todd RC, Michael JA. 2020. Effect of supplemental protease on growth performance and excreta microbiome of broiler chicks. Microorganisms. 2020(8):475. doi:10.3390/microorganisms8040475.
- Jha R, Das R, Oak S, Mishra P. 2020. Probiotics (direct-fed microbials) in poultry nutrition and their effects on nutrient utilization, growth and laying performance, and gut health: a systematic review. Animals. 10:1863. doi:10.3390/ani10101863.
- Jha R, Mishra P. 2021. Dietary fiber in poultry nutrition and their effects on nutrient utilization, performance, gut health, and on the environment: a review. J Anim Sci Biotechnol. 12:51. doi:10.1186/s40104-021-00576-0.
- Jozefiak D, Rutkowski A, Kaczmarek S, Jensen BB, Engberg RM, Hojbjerg O. 2010. Effect of β-glucanase and xylanase supplementation of barley- and rye-based diets on caecal microbiota of broiler chickens. Br Poult Sci. 51:546–557. doi:10.1080/00071668.2010.507243.
- Kaczmarek SA, Rogiewicz A, Mogielnicka M, Rutkowski A, Jones RO, Slominski BA. 2014. The effect of protease, amylase, and nonstarch polysaccharide-degrading enzyme supplementation on nutrient utilization and growth performance of broiler chickens fed corn-soybean meal-based diets. Poult Sci. 93:1745–1753. doi:10.3382/ps.2013-03739.
- Kai Y. 2021. Intestinal villus structure contributes to even shedding of epithelial cells. Biophys J. 120:699–710. doi:10.1016/j.bpj.2021.01.003.
- Kalmendal R, Tauson R. 2012. Effects of a xylanase and protease, individually or in combination, and an. ionophore coccidiostat on performance, nutrient utilization, and intestinal morphology in broiler chickens fed a wheat soybean meal-based diet. Poult Sci. 91:1387–1393. doi:10.3382/ps.2011-02064.
- Kamel NF, Ragaa M, El-Banna RA, Mohameda FF. 2015. Effects of a monocomponent protease on performance parameters and protein digestibility in broiler chickens. Agric Agric Sci Procedia. 6:216–225. doi:10.1016/j.aaspro.2015.08.062.
- Kamran Z, Sarwar M, Nisa M, Nadeem MA, Mahmood S, Babar ME, Ahmed S. 2008. Effect of low-protein diets having constant energy-to-protein ratio on performance and carcass characteristics of broiler chickens from one to thirty-five days of Age. Poult Sci. 87:468–474. doi:10.3382/ps.2007-00180.
- Kanagaraju P, Rathnapraba S, Churchil R, Madhan Kumar N, Swapnil S. 2019. Nutritional interventions to improve breast meat yield in broilers – review. Int J Livest Res. 9:49–61. doi:10.5455/ijlr.20170911084640.
- Kaunitz JD, Akiba Y. 2019. Control of intestinal epithelial proliferation and differentiation: the microbiome, enteroendocrine L cells, telocytes, enteric nerves, and GLP, too. Dig Dis Sci. 64:2709–2716. doi:10.1007/s10620-019-05778-1.
- Kaygisiz F, Cevger Y. 2010. Effects of marketing chicken meat as a whole or cut up on enterprise income. Turk J Vet Anim Sci. 34:17–23. doi:10.3906/vet-0709-24.
- Khan R, Naz S. 2013. The application of probiotics in poultry production. World’s Poult Sci J. 69(3):621–632. doi:10.1017/S0043933913000627.
- Khan SH, Iqbal J. 2016. Recent advances in the role of organic acids in poultry nutrition. J Appl Anim Res. 44:359–369.
- Khan SH, Sarder R, Siddique B. 2006. Influence of enzyme on performance of broiler fed sunflower corn based diets. Pak Vet J. 26:109–114.
- Khattak FM, Pasha TN, Hayat Z, Mahmud A. 2006. Enzymes in poultry nutrition. J Anim Plant Sci. 16:1–7.
- Kiarie E, Romero LF, Ravindran V. 2014. Growth performance, nutrient utilization, and digesta characteristics in broiler chickens fed corn or wheat diets without or with supplemental xylanase. Poult Sci. 93:1186–1196. doi:10.3382/ps.2013-03715.
- Kim JJ, Khan WI. 2013. Goblet cells and mucins: role in innate defense in enteric infections. Pathogens. 2:55–70.
- Kim YJ, Kim TH, Song MH, An JS, Yun W, Lee JH, Oh HJ, Lee JS, Kim GM, Kim HB, Cho JH. 2020. Effects of different levels of crude protein and protease on nitrogen utilization, nutrient digestibility, and growth performance in growing pigs. J Anim Sci Technol. 62(5):659–667. doi:10.5187/jast.2020.62.5.659.
- Koc H, Samli A, Okur M, Akyurek OH, Senkoylu N. 2010. Effects of saccharomyses cerevisiae and / or Mannan Oligosaccharides on performance, blood parameters and intestinal microbiota of broiler chicks. Bulg J Agric Sci. 16:643–650.
- Kocher A, Choct M, Porter MD, Broz J. 2002. Effects of feed enzymes on nutritive value of soyabean meal fed to broilers. Br Poult Sci. 43:54–63. doi:10.1080/00071660120109890.
- Law FL, Zulkifli I, Soleimani AF, Liang JB, Awad EA. 2018. The effects of low-protein diets and protease supplementation on broiler chickens in a hot and humid tropical environment. Asian-Australas J Anim Sci. 31:1291–1300. doi:10.5713/ajas.17.0581.
- Lee SA, Bedford MR, Walk CL. 2018. Meta-analysis: explicit value of mono-component proteases in monogastric diets. Poult Sci. 97:2078–2085. doi:10.3382/ps/pey042.
- Leeming ER, Johnson AJ, Spector TD, Le Roy CI. 2019. Effect of diet on the gut microbiota: rethinking intervention duration. Nutrients. 11:2862. doi:10.3390/nu11122862.
- Lemme A, Ravindran V, Bryden WL. 2004. Ileal digestibility of amino acids in feed ingredients for broilers. World’s Poult Sci J. 60:423–437. doi:10.1079/WPS200426.
- Lewis K, Lutgendorff F, Phan V, Söderholm JD, Sherman PM, McKay DM. 2010. Enhanced translocation of bacteria across metabolically stressed epithelia is reduced by butyrate. Inflamm Bowel Dis. 16:1138–1148. doi:10.1002/ibd.21177.
- Liisa A, Andrus S, van der Post S, Ana MRP, Anna E, Andre S, Fredrik B, Malin EVJ, Gunnar CH. 2020. Cell Rep. 30:1077–1087. doi:10.1016/j.celrep.2019.12.068.
- Liu SY, Sell PH, Court SG, Cowieson AJ. 2013. Protease supplementation of sorghum-based diets enhances amino acid digestibility coefficients in four small intestinal sites and accelerates their rates of digestion. Anim Feed Sci Technol. 183:175–183. doi:10.1016/j.anifeedsci.2013.05.006.
- Louis P, Flint HJ. 2009. Diversity, metabolism and microbial ecology of butyrate-producing bacteria from the human large intestine. FEMS Microbiol Lett. 294:1–8. doi:10.1111/j.1574-6968.2009.01514.x.
- Luo D, Yang F, Yang X, Yao J, Shi B, Zhou Z. 2009. Effects of xylanase on performance, blood parameters, intestinal morphology, microflora and digestive enzyme activities of broilers fed wheat-based diets. Asian-Australas J Anim Sci. 22:1288–1295. doi:10.5713/ajas.2009.90052.
- Madhusankha GDMP, Thilakarathna RCN. 2021. Meat tenderization mechanism and the impact of plant exogenous proteases: a review. Arab J Chem. 14:102967. doi:10.1016/j.arabjc.2020.102967.
- Mahmood T, Mirzaa MA, Nawaza H, Shahid M. 2017. Effect of different exogenous proteases on growth performance, nutrient digestibility, and carcass response in broiler chickens fed poultry by-product meal-based diets. Livest Sci. 200:71–75. doi:10.1016/j.livsci.2017.04.009.
- Marapana R. 2016. Effect of different dress weight categories on yield part percentage and relationship of live and dress weight of broiler carcasses slaughter at different conditions. J Food Sci Technol Nepal. 9:31–38. doi:10.3126/jfstn.v9i0.14760.
- Markovic R, Sefer D, Krstic M, Petrujkic B. 2009. Effect of different growth promoters on broiler performance and gut morphology. Arch Med Vet Sci. 41:163–169. doi:10.4067/S0301-732X2009000200010.
- Markowiak P, Śliżewska K. 2017. Effects of probiotics, prebiotics, and synbiotics on human health. Nutrients. 9:1021. doi:10.3390/nu9091021.
- Masey O'Neill HV, Smith JA, Bedford MR. 2014. Multicarbohydrase enzymes for non-ruminants. Asian-Australas J Anim Sci. 27:290–301. doi:10.5713/ajas.2013.13261.
- Medhi D, Ahmed HF, Konwar BK, Chakravarty P. 2003. Effect of dietary ajar seed meal with or without multienzyme supplementation on voluntary feed intake and nutrients digestibility in broilers. Indian Vet J. 80:667–671.
- Mohammadigheisar M, Kim IH. 2018. Addition of a protease to low crude protein density diets of broiler chickens. J Appl Anim Res. 46(1):1377–1381. doi:10.1080/09712119.2018.1512862.
- Moosavi M, Chaji M, Boujarpour M, Rahimnahal S, Kazemi AR. 2012. Effect of different levels of energy and protein with constant ratio on performance and carcass characteristics in broiler chickens. Int Res J Appl Basic Sci. 3(12):2485–2488.
- Morrison DJ, Preston T. 2016. Formation of short chain fatty acids by the gut microbiota and their impact on human metabolism. Gut Microbes. 7:189–200. doi:10.1080/19490976.2015.1134082.
- Munyaka PM, Nandha NK, Kiarie E, Nyachoti CM, Khafipour E. 2016. Impact of combined β-glucanase and xylanase enzymes on growth performance, nutrients utilization and gut microbiota in broiler chickens fed corn or wheat-based diets. Poult Sci. 95:528–540. doi:10.3382/ps/pev333.
- Mushtag TS, Ahmad M, Mirza G, Ahmad MA, Noreen T. 2009. Influence of sunflower meal based diet supplemented with exogenous enzyme and digestible lysine on performance, digestibility and carcass response of broiler chickens. Anim Feed Sci Technol. 149:275–286. doi:10.1016/j.anifeedsci.2008.06.008.
- Nabizadeh A, Golian A, Hassanabadi A, Zerehdaran S. 2017. Effects of nutrient density and exogenous enzymesin starter diet on performance, intestinal microflora, gut morphology and immune response of broiler chickens. Braz J Poult Sci. 19:509–518. doi:10.1590/1806-9061-2017-0501.
- Ndazigaruye G, Kim D-H, Kang C-W, Kang K-R, Joo Y-J, Lee S-R, Lee K-W. 2019. Effects of low-protein diets and exogenous protease on growth performance, carcass traits, intestinal morphology, cecal volatile fatty acids and serum parameters in broilers. Animals. 9:226. doi:10.3390/ani9050226.
- Neves DP, Banhazi TM, Nääs IA. 2014. Feeding behaviour of broiler chickens: a review on the biochemical characteristics. Rev Bras Ciênc Avíc. 16:1–16. doi:10.1590/1516-635x16021-16.
- NRC. 1994. Nutrient requirements of poultry, 9th rev. ed. Washington, DC: National Academy Press. p. 176.
- Odetallah NH, Wang JJ, Garlich JD, Shih JC. 2003. Keratinase in starter diets improves growth of broiler chicks. Poult Sci. 82:664–670. doi:10.1093/ps/82.4.664.
- Odetallah NH, Wang JJ, Garlich JG, Shih JC. 2005. Versazyme supplementation of broiler diets improves market growth performance. Poult Sci. 84:858–864. doi:10.1093/ps/84.6.858.
- O'Keefe SJD. 2016. Diet, microorganisms and their metabolites, and colon cancer. Nat Rev Gastroenterol Hepatol. 13:691–706. doi:10.1038/nrgastro.2016.165.
- Olukosi O, Beeson L, Englyst K, Romero L. 2015. Effects of exogenous proteases without or with carbohydrases on nutrient digestibility and disappearance of non-starch polysaccharides in broiler chickens. Poult Sci. 94:2662–2669. doi:10.3382/ps/pev260.
- Omojola AB, Otunla TA, Olusola OO, Adebiyi OA, Ologhobo AD. 2014. Performance and carcass characteristics of broiler chicken fed soybean and sesame/soybean based diets supplemented with or without microbial phytase. Am J Exp Agric. 4:1637–1648. doi:10.9734/ajea/2014/10513.
- Opoku EY, Classen HL, Scott TA. 2015. Evaluation of inclusion level of wheat distillers dried grains with solubles with and without protease or β-mannanase on performance and water intake of Turkey hens. Poult Sci. 94:1600–1610. doi:10.3382/ps/pev088.
- Oyeagu CE, Ani AO, Egbu CF, Akpolu ES, Iwuchukwu JC, Omumuabuike JN. 2015. Performance of broiler finisher birds fed Toasted Bambara nut (Vigna subterranean (L) verdc) Offal with supplementary enzyme. Asian J Sci Technol. 6:934–939.
- Oyeagu CE, Ani AO, Egbu CF, Udeh FU, Omumuabuike JN, Iwuchukwu JC. 2016. The effect of feeding toasted Bambara nut (Vigna subterranea (L) verdc) offal and supplementary enzyme on performance of broiler chicks. J Trop Agric (Trinidad). 93:271–283.
- Oyeagu CE, Mlambo V, Muchenje V. 2019a. Effect of dietary Aspergillus xylanase on nutrient digestibility and utilization, growth performance and size of internal organs in broiler chickens offered maize-soybean meal based-diets. Pak J Nutr. 18:852–865. doi:10.3923/pjn.2019.852.865.
- Oyeagu CE, Mlambo V, Muchenje V, Marume U. 2019b. Effect of dietary supplementation of Aspergillus xylanase in broiler chicken. Iran J Appl Anim Sci. 9:693–708.
- Parsaie S, Shariatmadari F, Zamiri MJ, Khajeh K. 2007. Influence of wheat-based diets supplemented with xylanas, bile acid and antibiotics on performance, digestive tract measurements and gut morphology of broilers compared with a maize-based diet. Br Poult Sci. 48:594–600. doi:10.1080/00071660701615788.
- Parviz A, Javad G, Heydar Z, Zohre S, Hassan K, Mohammad RED. 2018. Effect of probiotic and vinegar on growth performance, meat yields, immune responses, and small intestine morphology of broiler chickens. Ital J Anim Sci. 17(3):675–685. doi:10.1080/1828051X.2018.1424570.
- Peng L, Li ZR, Green RS, Holzman IR, Lin J. 2009. Butyrate enhances the intestinal barrier by facilitating tight junction assembly via activation of AMP-activated protein kinase in Caco-2 cell monolayers. J Nutr. 139:1619–1625. doi:10.3945/jn.109.104638.
- Petracci M, Baéza E. 2011. Harmonization of methodologies for the assessment of poultry meat quality features. World’s Poult Sci J. 67:137–151. doi:10.1017/S0043933911000122.
- Petracci M, Betti M, Bianchi M, Cavani C. 2004. Color variation and characterization of broiler breast meat during processing in Italy. Poult Sci. 83:2086–2092. doi:10.1093/ps/83.12.2086.
- Pickard JM, Zeng MY, Caruso R, Núñez G. 2017. Gut microbiota: role in pathogen colonization, immune responses, and inflammatory disease. Immunol Rev. 279:70–89. doi:10.1111/imr.12567.
- Qaid MM, Abdelrahman MM. 2016. Role of insulin and other related hormones in energy metabolism – a review. Cogent Food Agric. 2:1267691. doi:10.1080/23311932.2016.1267691.
- Rada V, Lichovníková M, Foltyn M, Šafařík I. 2016. The effect of exogenous protease in broiler diets on the apparent ileal digestibility of amino acids and on protease activity in jejunum. Acta Univ Agric Silvic Mendel Brun. 64(183):5. doi:10.11118/actaun201664051645.
- Rizz C, Marangon A, Chiericat GM. 2007. Effects of genotype on slaughtering performance and meat physical and sensory characteristics of organic laying chickens. Poult Sci. 86:128–135. doi:10.1093/ps/86.1.128.
- Rodrigues I, Choct M. 2018. The foregut and its manipulation via feeding practices in the chicken. Poult Sci. 97:3188–3206. doi:10.3382/ps/pey191.
- Rojas OJ, Stein HH. 2017. Processing of ingredients and diets and effects on nutritional value for pigs. J Anim Sci Biotechnol. 8:48. doi:10.1186/s40104-017-0177-1.
- Sanka YD, Mbanga SH. 2014. Evaluation of Tanzanian local chicken reared under intensive and semi-intensive systems: II. Meat quality attributes. Lives Res Rural Dev. 26:78–81.
- SAS. 2010. SAS users guide: statistics, version 9.3. Cary (NC): SAS Institute.
- Sayehban P, Seidavi A, Dadashbeiki M, Ghorbani A, Araújo WAG, Albino LFT. 2015. Effects of different dietary levels of two types of olive pulp and exogenous enzyme supplementation on the gastrointestinal tract size, immunology and hematology of broilers. Braz J Poult Sci. 17:73–85. doi:10.1590/1516-635XSPECIALISSUENutrition-PoultryFeedingAdditives073-086.
- Sayehban P, Seidavi A, Dadashbeiki M, Ghorbani A, Araújo WAG, Albino LFT. 2016. Effects of different levels of two types of olive pulp with or without exogenous enzyme supplementation on broiler performance and economic parameters. Braz J Poult Sci. 18:489–499. doi:10.1590/1806-9061-2015-0060.
- Schoenfeld BJ, Aragon AA. 2018. How much protein can the body use in a single meal for muscle-building? Implications for daily protein distribution. J Int Soc Sports Nutr. 15:10. doi:10.1186/s12970-018-0215-1.
- Schweihofer JP. 2011. Carcass dressing percentage and cooler shrink. Michigan State University Extension. https://www.canr.msu.edu/news/carcass_dressing_percentage_and_cooler_shrink.
- Scott TA. 2002. Impact of wet feeding wheat-based diets with or without enzyme on broiler chick performance. Can J Anim Sci. 82:409–417.
- Selle PH, Cadogan DJ, Ru YJ, Partridge GG. 2010. Impact of exogenous enzymes in sorghum or wheat based broiler diets on nutrient utilization and growth performance. Int J Poult Sci. 9:53–58. doi:10.3923/ijps.2010.53.58.
- Selle PH, Liu SY, Cai J, Cowieson AJ. 2013. Steam-pelleting temperatures, grain variety, feed form and protease supplementation of mediumly ground, sorghum-based broiler diets: influences on growth performance, relative gizzard weights, nutrient utilisation, starch and nitrogen digestibility. Anim Prod Sci. 53:378–387. doi:10.1071/AN12363.
- Shakouri MD, Iji PA, Mikkelsen LL, Cowieson AJ. 2008. Intestinal function and gut microflora of broiler chickens as influenced by cereal grains and microbial enzyme supplementation. J Anim Physiol Anim Nutr. 93:647–658. doi:10.1111/j.1439-0396.2008.00852.x.
- Silveira MHD, Usso JTZ, Rossi P, Rutz F, Anciuti MA, Zauk NF, Ribeiro CLG, Brum PAR, Nunes JK. 2010. Efeito da peletização em dietas contendo complexo enzimático para frangos de corte. Ciência Animal Brasileira. 11:326–333. doi:10.5216/cab.v11i2.3846.
- Sobolewska A, Bogucka J, Dankowiakowska A, Elminowska-Wenda G, Stadnicka K, Bednarczyk M. 2017. The impact of synbiotic administration through in ovo technology on the microstructure of a broiler chicken small intestine tissue on the 1st and 42nd day of rearing. J Anim Sci Biotechnol. 8:61. doi:10.1186/s40104-017-0193-1.
- Staley C, Weingarden AR, Khoruts A, Sadowsky MJ. 2017. Interaction of gut microbiota with bile acid metabolism and its influence on disease states. Appl Microbiol Biotechnol. 101:47–64. doi:10.1007/s00253-016-8006-6.
- Steel RGD, Torrie JH. 1980. Principles and procedures of statistics. A biometric approach. 2nd ed. New York (NY): McGraw-Hill Publishers.
- Sudhir Y, Rajesh J. 2019. Strategies to modulate the intestinal microbiota and their effects on nutrient utilization, performance, and health of poultry. J Anim Sci Biotechnol. 10:1–11. doi:10.1186/s40104-018-0310-9.
- Sugiharto S, Ranjitkar S. 2019. Recent advances in fermented feeds towards improved broiler chicken performance, gastrointestinal tract microecology and immune responses: a review. Animal Nutrition. 5(1):1–10. doi:10.1016/j.aninu.2018.11.001.
- Tejeda OJ, Kim WK. 2021. Role of dietary fiber in poultry nutrition. Animals. 11:461. doi:10.3390/ani11020461.
- Tuohy KM, Ziemer CJ, Klinder A, Knobel Y, Pool-Zobel BL, Gibson GR. 2002. A human volunteer study to determine the prebiotic effects of lactulose powder on human colonic micro-biota. Microb Ecol Health Dis. 14:165–173. doi:10.1080/089106002320644357.
- Van der Wielen PW, Biesterveld S, Notermans S, Hofstra H, Urlings BAP, van Knapen F. 2000. Role of volatile fatty acids in development of the cecal microflora in broiler chickens during growth. J Appl Environ Microbiol. 66:2536–2540. doi:10.1128/AEM.66.6.2536-2540.2000.
- Vu DH, Wainaina S, Taherzadeh MJ, Åkesson D, Ferreira JA. 2021. Production of polyhydroxyalkanoates (PHAs) by Bacillus megaterium using food waste acidogenic fermentation-derived volatile fatty acids. Bioengineered. 12(1):2480–2498. doi:10.1080/21655979.2021.1935524.
- Walk CL, Juntunen K, Paloheimo M, Ledoux DR. 2019. Evaluation of novel protease enzymes on growth performance and nutrientdigestibility of poultry: enzyme dose response. Poult Sci. 98:5525–5532. doi:10.3382/ps/pez299.
- Wang ZR, Qiao SY, Lu WQ, Li DF. 2005. Effects of enzyme supplementation on performance, nutrient digestibility, gastrointestinal morphology, and volatile fatty acid profiles in the hindgut of broilers fed wheat-based diets. Poult Sci. 84:875–881. doi:10.1093/ps/84.6.875.
- Werner C, Janisch S, Kuembet U, Wicke M. 2009. Comparative study of the quality of broiler and Turkey meat. Br Poult Sci. 50:318–324. doi:10.1080/00071660902806939.
- Wexler HM. 2007. Bacteroides: the good, the bad, and the nitty-gritty. Clin Microbiol Rev. 20:593–621. doi:10.1128/CMR.00008-07.
- Wilfarta A, Montagne L, Simmins H, Noblet J, van Milgen H. 2007. Digesta transit in different segments of the gastrointestinal tract of pigs as affected by insoluble fibre supplied by wheat bran. Br J Nutr. 98:54–62. doi:10.1017/S0007114507682981.
- Wu QJ, Zhou YM, Wu YN, Wang T. 2013. Intestinal development and function of broiler chicken on diets supplemented with clinoptilolite. Asian Australas J Anim Sci. 26:987–994. doi:10.5713/ajas.2012.12545.
- Wu S, Choct M, Pesti G. 2020. Historical flaws in bioassays used to generate metabolizable energy values for poultry feed formulation: a critical review. Poult Sci. 99:385–406. doi:10.3382/ps/pez511.
- Xiao Y, Xiang Y, Zhou W, Chen J, Li K, Yang H. 2017. Microbial community mapping in intestinal tract of broiler chicken. Poult Sci. 96:1387–1393. doi:10.3382/ps/pew372.
- Xu ZR, Hu CH, Xia MS, Zhan XA, Wang MQ. 2003. Effects of dietary fructooligosaccharide on digestive enzyme activities, intestinal microflora and morphology of male broilers. Poult Sci. 82:1030–1036. doi:10.1093/ps/82.6.1030.
- Yadav J, Sah R. 2005. Supplementation of corn-soybean based broiler’s diets with different levels of acid protease. J Inst Agric Anim Sci. 26:65–70. doi:10.3126/jiaas.v26i0.613.
- Yadav S, Jha R. 2019. Strategies to modulate the intestinal microbiota and their effects on nutrient utilization, performance, and health of poultry. J Anim Sci Biotechnol. 10:2. doi:10.1186/s40104-018-0310-9.
- Young LL, Northcutt JK, Buhr RJ, Lyon CE, Wane GO. 2001. Effects of age, sex and duration of postmortem aging on percentages yield of parts from broiler chicken carcasses. Poult Sci. 80:376–379. doi:10.1093/ps/80.3.376.
- Yuan L, Wang M, Zhang X, Wang Z. 2017. Effects of protease and non-starch polysaccharide enzyme on performance, digestive function, activity and gene expression of endogenous enzyme of broilers. PLoS One. 12:e0173941. doi:10.1371/journal.pone.0173941.
- Zakaria HAH, Jalai MAR, Ishmais MAA. 2010. The influence of supplemental multi-enzymes feed additive on the performance, carcass characteristics, and meat quality traits or broiler chickens. Int J Poult Sci. 9:126–133. doi:10.3923/ijps.2010.126.133.
- Zuo J, Ling B, Long L, Li T, Lahaye L, Yang C, Feng D. 2015. Effect of dietary supplementation with protease on growth performance, nutrient digestibility, intestinal morphology, digestive enzymes and gene expression of weaned piglets. Anim Nutr. 1:276–282. doi:10.1016/j.aninu.2015.10.003.