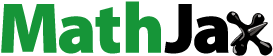
ABSTRACT
Saponin possessed antiprotozoal activity; therefore, it is generally used to mitigate enteric methane (CH4) emission in ruminant nutrition. The objective of this study was to evaluate the effects of Aloe saponaria (AS) supplementation on rumen fermentation, CH4, and microbial diversity in an in vitro experiment over 48 h incubation. Five treatments were used: (1) no additives (CON), 1% and 2% dosage of heat-dried AS (HAS), and freeze-dried AS (FAS), and the experiments were performed three times. After the incubation, volatile fatty acids (VFAs), CH4 proportion in total gas, and microbial diversity were evaluated. Gas production was assessed at 3, 6, 9, 12, 24, 36, and 48 h incubation. FAS addition significantly increased gas production compared to CON except for 6 and 48 h, whereas HAS addition did not significantly affect the gas production at all observed time points. AS addition significantly increased total VFA and the absolute abundances of fungi and Ruminococcus albus regardless of drying method. There was no significant change in the CH4 proportion at 48 h, although the reduction in ciliate protozoa was detected in FAS-treated groups. In conclusion, FAS may be an effective feed additive to improve ruminal fermentation via enhancing feed utilization by rumen microbes.
Introduction
The mitigation of enteric methane (CH4) emission in ruminants is one of the main goals which ruminant nutritionists have tried to solve because CH4 produced from enteric fermentation accounts for 39.1% of global greenhouse gas from the livestock supply chain (Gerber et al. Citation2013). Therefore, various feed additives have been applied to mitigate ruminal CH4 production through the modulation of ruminal microorganisms (Jayanegara et al. Citation2009; Bodas et al. Citation2012; Kim et al. Citation2020). Among them, plant secondary compounds (e.g. saponins, tannins, flavonoids, and essential oils) are non-nutritive plant metabolites and have been widely applied to feed additives to ruminant (Niu et al. Citation2021; Xie et al. Citation2022) because they contain distinct antimicrobial compounds with the potential to inhibit ruminal methanogens (Patra et al. Citation2017).
Saponin, a terpenoid subclass, is an important plant secondary compound found in numerous folk herbal plants (Wanapat et al. Citation2018; El Aziz et al. Citation2019; Gunun et al. Citation2019; Khejornsart et al. Citation2021). Saponin can bind to sterol on the protozoal membrane surface, causing protozoal lysis (Wina et al. Citation2005). Extensive research using saponin-containing plants (SCPs) or saponin extracts from various plants has been conducted to investigate the CH4 mitigation effects through specific reduction of protozoa in the rumen; however, the CH4 mitigation effect by saponin supplementation has been inconsistent depending on the extraction method, dose level, and plant type (Patra and Yu Citation2014; Cieslak et al. Citation2014; Belanche et al. Citation2015). Therefore, trials to identify new SCPs are ongoing.
Aloe saponaria (AS) consists of a terpenoid saponin (Choi et al. Citation2015) and various other plant secondary compounds such as flavonoids (rutin 21.30 mg/g, quercetin 10.94 mg/g, and kaempferol 3.86 mg/g) and phenols (gallic acid 10.53 mg/g and caffeic acid 4.72 mg/g) (Silva et al. Citation2013). Although AS may have the potential to inhibit ruminal CH4 production owing to its content of various plant metabolites, a few research has been tried to investigate the effect of AS supplementation on rumen fermentation and CH4 mitigation to the best of our knowledge. According to the previous studies, drying methods, especially drying temperature, could affect the plant's primary and secondary metabolites, and the freeze-drying method is a more suitable method for retaining phenols in Korean mint flower compared to the heat-drying method (Mahanom et al. Citation1999; Mediani et al. Citation2015; Park et al. Citation2021).
Therefore, we hypothesized that the supplementation of AS could reduce ruminal protozoa, thereby decreasing CH4 production, and there is a difference in antiprotozoal efficacy by drying method of AS (freeze-drying versus heat-drying). The objective of the present study was to evaluate the effects of the addition of differently dried AS on ruminal fermentation, antiprotozoal efficacy, CH4, and microbial diversity in an in vitro experiment.
Materials and methods
All experimental protocols for the use of animals were approved by the Animal Research Ethics Committee of Pusan National University (PNU-2020-2827).
Experimental diet, feed additives, and chemical analysis
The feed ingredients used for the experimental total mixed ration (TMR) and the estimated chemical compositions are presented in . The heat-dried AS (HAS) was purchased from a local aloe farm (KDYALOE®, Ulsan, Korea). To guarantee the same ingredient specification, whole AS was also purchased from the local aloe farm, and whole AS was freeze-dried (FAS) in the laboratory until the dry matter (DM) contents were over 90%. The analysed chemical compositions of AS are presented in . Before chemical analysis, all feed ingredients used in this study were dried and, ground using a cyclone mill (Foss Tecator Cyclotec 1093; Foss, Hillerød, Denmark) equipped with a 1 mm screen. The contents of DM (#934.01), acid detergent fibre (ADF; #973.18), crude protein (CP; #990.03), ash (#942.05), and ether extract (EE; #2003.05) were analysed (AOAC Citation2005). To measure the total amount of nitrogen, Kjeldahl analysis was carried out using a nitrogen combustion analyzer (LECO FP-528; LECO Corporation, St Joseph, MI, USA), and the CP content was estimated by multiplying the total nitrogen content by 6.25. The neutral detergent fibre after heat-stable amylase (α-amylase) treatment (aNDF) and lignin content were analysed (Van Soest et al. Citation1991), and aNDF was expressed inclusive of residual ash. The total digestible nutrients and net energy for maintenance were estimated (National Research Council Citation2001).
Table 1. Ingredients for the experimental diet and its estimated chemical composition.
Table 2. Analysed chemical compositions of Aloe saponaria.
Experimental treatment
A complete randomized design was used in the present study, and the main effect was treatment. The five experimental treatments were as follows: no addition of AS (CON), 1% and 2% addition of HAS (HAS1 and HAS2, respectively), and the same level of FAS addition (FAS1 and FAS2, respectively).
In vitro incubation
Rumen fluid was obtained from two cannulated Holstein steers (body weight, 450 ± 30 kg) fed a 60:40 timothy hay:concentrate diet, before morning feeding at the Center for Agriculture Research, Pusan National University (Korea). The collected rumen fluid contained in a thermos bottle was transferred to the laboratory within 30 min. Four layers of cheesecloth were used to strain the rumen fluid, and the strained rumen fluid was thoroughly mixed with 4 × volume of in vitro buffer solution (Goering and Van Soest Citation1970) under strictly anaerobic conditions. The experimental TMR (0.5 g) was put into pre-weighed nylon bags (R510; ANKOM Technology, Macedon, NY, USA). For the FAS and HAS treatments, each dosage of FAS and HAS was added to the nylon bags, and all nylon bags were heat-sealed and put into 500 mL duran bottles. In vitro experiments were performed three times, and for each experiment, four replicates were used per treatment, and each bottle contained four bags. Then, 250 mL of the mixture of rumen fluid and in vitro buffer solution was put into the duran bottles, with continuous flushing of O2-free CO2 gas. The duran bottles were sealed using bottle caps equipped with butyl rubber stoppers and rubber rings and incubated on a rotary shaker (JSSI-300 T, JS Research Inc., Gongju, Korea) at 20 rpm at 39°C for 48 h.
Experimental treatment and sample collection and analyses
Gas production was measured at 3, 6, 9, 12, 24, 36, and 48 h using a pressure transducer (Sun Bee Instrument Inc., Seoul, Korea) as described by Theodorou, Williams (Theodorou et al. Citation1994). The kinetic parameters of gas production were fitted to a simple exponential model using the following equations (Schofield et al. Citation1994):
where T is time (h), VT is the gas volume at T (mL), L is lag time (h), and e is the exponential function. Kg is the fractional rate of gas production (h−1). Vmax is the theoretical maximum gas production (mL).
The headspace gas generated by incubation was removed from the bottles every 2 h because of possible inhibition of microbial activity by headspace gas pressure. Before removing, the headspace gas was collected in 12-mL exetainer vacuum vials (Labco Limited, Lampeter, UK). After then, collected headspace gas was gathered into 12-ml Exetainer vacuum vials according to the proportion of the gas volume at each time point to the total gas volume. The concentration of CH4 at 48 h was analysed using an IGC-7200 gas chromatograph (DS Science, Gyeonggi-do, Korea) having a thermal conductivity detector and HayeSep Q 80/100 columns (Restek Corporation, Bellefonte, PA, USA). The carrier gas was helium at a flow rate of 20 mL/min. The column oven, injector, and detector temperatures were 40, 100, and 80°C, respectively.
The bottle caps were removed after 48 h incubation time; then, the duran bottles were kept on ice to stop fermentation. The nylon bags were then removed from the duran bottles and rinsed using tap water until the water ran clear. The washed nylon bags were dried at 60°C for 72 h to measure in vitro dry matter degradability (IVDMD). The aNDF of the weighed nylon bags was assessed to measure in vitro neutral detergent fibre degradability (IVNDFD) (Pell and Schofield Citation1993). The CP content of the weighed bags was analysed using Kjeldahl analysis to evaluate in vitro crude protein degradability (IVCPD). After keeping at 5 min in ice to stop the additional fermentation, the 1.8 mL of fluid was collected into the 2-mL collection tube and centrifuged at 20,000 × g at 4°C for 20 min (Castillejos et al. Citation2006). After centrifuging, the supernatant was removed and the remaining pellet was stored at – 80°C until microbial genomic DNA extraction.
Approximately 50 mL of culture was pipetted into a centrifugal tube and centrifuged at 3500 rpm at 4°C for 20 min. The supernatant was aliquoted to measure pH, volatile fatty acid (VFA), and ammonia nitrogen (NH3-N). The pH was measured by a pH meter (FP20, Mettler Toledo, Columbus, OH, USA). The aliquot for VFA analysis was mixed with 200 μL of 25% meta-phosphoric acid, and the other aliquot for NH3-N analysis was mixed with 200 μL of 0.2 M sulphuric acid, followed by storage at – 20°C before each analysis. To evaluate VFA concentration, the stored aliquot was centrifuged at 20,000 × g at 4°C for 20 min, and then the supernatant (200 μL) was diluted with anhydrous ethyl alcohol (800 μL) (4023-2304, Daejung Chemicals, Siheung-si, Korea). Each VFAs were measured using an Agilent 7890A gas chromatograph (Agilent Technologies, Santa Clara, CA, USA) having a flame ionization detector and capillary column (Nukol ™ Fused silica capillary column, 30 m × 250 μm × 0.25 μm; Supelco Inc., Bellefonte, PA, USA). The injector, oven, and detector temperatures were set at 90–200, 90, and 230°C, respectively. The carrier gas was nitrogen was at a flow rate of 30 mL/min. The NH3-N concentration was analysed with several modifications (Chaney and Marbach Citation1962). Briefly, the supernatant of aliquot (2 µL) centrifuged at 20,000 × g at 4°C for 20 min was mixed with 100 µL alkali hypochlorite solution (25 g of sodium hydroxide, 16.8 mL of sodium hypochlorite, and 1 L of distilled water) and 100 µL phenol colour solution (50 g of phenol, 0.25 g of sodium nitroferricyanide, and 1 L of distilled water). Then, the mixture was incubated at 37°C for 15 min. The concentration of NH3-N was assessed by measuring the optical density at 630 nm using a microplate reader (iMARK; Bio-Rad Laboratories, Hercules, CA, USA).
Microbial genomic DNA extraction and quantitative polymerase chain reaction
To extract microbial genomic DNA, the pellet stored at – 80°C was melted in ice, and the genomic DNA was extracted following the repeated bead-beating plus column method (Yu and Morrison Citation2004). The quality and quantity of DNA were measured using a NanoDrop ND-1000 spectrophotometer (Thermo Fisher Scientific, Waltham. MA, USA). Quantitative polymerase chain reaction (qPCR) was performed using a CFX 96 Touch system (Bio-Rad Laboratories). The information of the primer-set used in this study is presented in . The reaction mixture (20 μL) for the qPCR contained 10× buffer (2 μL, BioFACT Co., Ltd., Daejeon, Korea), 10 mM dNTP mixture (0.5 μL, BioFACT Co., Ltd.), 10-fold diluted genomic DNA (1 μL), forward primer (1 μL, 10 μm), reverse primer (1 μL, 10 μm), Taq polymerase (0.1 μL, BioFACT Co., Ltd.), Evagreen (1 μL, SolGent Co. Ltd., Daejeon, Korea), and ultra-pure water (13.4 μL, Biosesang, Gyeonggi-do, Korea). The conditions of qPCR were following as: initial denaturation (95°C for 10 min), and 40 cycles of denaturation (95°C for 30 s), annealing (60°C for 30 s), and extension (72°C for 30 s), followed by a final extension (72°C for 5 min). At the end of each extension stage, the fluorescence was recorded. The specificity of the amplicon was assessed by the dissociation curve (from 60°C to 95°C, at a rate of 1°C per 30 s). To compute the absolute abundances of each microbe, each standard plasmid having the respective target sequence was used. The plasmid copy number was calculated as described by Whelan, Russell (Whelan et al. Citation2003), and each standard curve of microbes was computed using the 10-fold serial diluted plasmid DNA.
Table 3. Information of primer-set used for quantitative polymerase chain reaction
Statistical analysis
Statistical analysis was carried out using the GLIMMIX procedure (SAS 9.4; SAS Institute, Cary, NC, USA):
where
is the jth observation in the ith treatment,
is the overall mean, Ti is the model’s fixed effect of the ith treatment (i = 1-5), and eij is the residual error on the jth observation in the ith treatment. The statistical differences among the treatments were analysed by Tukey’s range test. Orthogonal contrasts were utilized to analyse the linear effect regardless of drying method, the differences between CON and HAS-treated groups (CON vs. HAS), and CON vs. FAS-treated groups (CON vs. FAS). Statistical significance among the treatments was declared at p < 0.05, and a statistical trend was assumed at 0.05 ≤ p < 0.10.
Results
Gas production and fitted parameters
Gas production in the FAS2 group was the highest among all treatments at 3, 9, 12, and 24 h (; 3 h, TRT p = 0.0378; 9 h, TRT p = 0.0062; 12 h, TRT p = 0.0038; and 24 h, TRT p = 0.0216), whereas there was no significant difference in gas production at the final incubation time (, TRT p = 0.2397). The gas production at 3 and 12 h was significantly increased in a dose-response manner (; LIN 3 h, p = 0.0309 and 12 h, p = 0.0422), whereas there was no significant increase in gas production at other time points. Between drying methods, overall increases in gas production were detected between the CON and FAS-treated groups (; 3 h, CON vs. FAS p = 0.0071; 9 h, CON vs. FAS p = 0.0091; 12 h, CON vs. FAS p = 0.0043; 24 h, CON vs. FAS p = 0.0129; 36 h, CON vs. FAS p = 0.0208; and 48 h, CON vs. FAS p = 0.0523). However, there was no significant increase in gas production at all observed time points between CON vs. HAS-treated groups (). Concerning the CH4 proportion in total gas production at 48 h, no significant differences were observed in all the statistical parameters (). Regarding the fitted gas parameters, the Kg showed a statistical trend among the treatments (, TRT p = 0.0781), although there was no significant difference in Vmax ().
Table 4. Gas production and fitted parameters in each treatment.
Rumen fermentation profiles and degradability parameters
The pH at 48 h in the FAS1 group was the highest among all treatments (, TRT p = 0.0313), whereas no significant difference in pH was observed with increasing level of AS. The NH3-N concentration showed statistical tendency in a dose-response manner (, LIN p = 0.0879), but there were no effect in NH3-N by the addition of HAS and FAS. In the degradability indices, IVDMD was significantly increased by the addition of FAS (, CON vs. FAS p = 0.0210), but IVNDFD and IVCPD was not affected by the addition level and both types of drying methods ().
Table 5. Rumen fermentation characteristics among treatments after an in vitro batch culture at 48 h incubation.
The total VFA concentration was significantly increased by the added level of AS and both types of drying methods (; LIN p = 0.0188; CON vs. HAS p = 0.0284; and CON vs. FAS p = 0.0024). For individual VFA concentrations, the FAS1 group had the highest acetate (, TRT p = 0.0332), propionate (, TRT p = 0.0433), and butyrate (, TRT p = 0.0380) concentrations. The increasing levels of AS induced an overall increase in the concentrations of individual VFAs (; acetate, LIN p = 0.0102; iso-butyrate, LIN p = 0.0141; and iso-valerate, LIN p = 0.0379). Between drying methods, FAS-treated groups showed significantly higher individual VFAs than CON, except valerate concentration, whereas only the concentration of acetate and iso-butyrate was significantly increased by the addition of HAS (). Regarding the molar proportions of individual VFAs, acetate was significantly increased in a linear manner, whereas that of propionate was decreased (; acetate, LIN p = 0.0074; propionate, LIN p < 0.0001).
Microbial diversity
The differences in microbial diversity among the treatments are shown in . The absolute abundance of general bacteria was the highest in the HAS1 group and the lowest in the CON group, although only a statistical trend was observed among the treatments (, TRT p = 0.0717). The absolute abundance of ciliate protozoa in the FAS2 group was the lowest among the treatments, but there were no significant differences in multi-comparisons among treatments (, TRT p = 0.0263). The absolute abundance of fungi was significantly higher in the HAS2 group than in the CON group (, TRT p = 0.0065). The increasing levels of AS significantly increased the absolute abundance of Fungi (LIN, p = 0.0328) and Selenomonas ruminantium (LIN, p = 0.0109), but there were no effects in other microbial diversity.
Table 6. Microbial abundance among treatments after an in vitro batch culture at 48 h incubation.
Between drying methods, reduction in ciliate protozoa was only detected in the FAS-treated groups (, CON vs. HAS p = 0.9221 and CON vs. FAS p = 0.0182). The HAS-treated groups exhibited significantly higher absolute abundances of methanogenic archaea, S. ruminantium, P. ruminicola, and Ruminococcus flavefaciences than the CON group (; methanogenic archaea, CON vs. HAS p = 0.0014; S. ruminantium, CON vs. HAS p = 0.0016; P. ruminantium, CON vs. HAS p = 0.0414; and R. flavefaciences, p = 0.0441), whereas FAS treatment did not significantly affect these microbes.
Discussion
Protozoa constitute a considerable proportion (up to 50%) of rumen microbial biomass (Williams and Coleman Citation2012), and they play diverse roles related to feed digestion and H2 production (Newbold et al. Citation2015). Thus, specific inhibition of protozoa is conceptually a sound strategy to alter fermentation patterns and rumen microbial diversity. In this study, we demonstrated that AS addition enhanced gas production and VFA production with modulation of ruminal microbes.
Several reports showed that the addition of SCP affected decrease of ruminal NH3-N concentration by reducing protozoal count (Hu et al. Citation2005; Pen et al. Citation2006; Holtshausen et al. Citation2009), and the possible explanation for decreasing NH3-N is as follow: (1) decreasing bacterial lysis induced by saponin’s antiprotozoal effects and (2) through the binding affinity of the sugar moiety in SCP to NH3-N (Wallace et al. Citation1994). However, in this study, we observed no significant change in NH3-N concentration, despite a significant reduction in ciliate protozoa in the FAS-treated groups. Similarly, several studies showed that SCP supplementation did not alter NH3-N concentration, although the protozoal counts were significantly reduced (Pen et al. Citation2008; Patra et al. Citation2012). In addition, Pen, Sar (Pen et al. Citation2006) showed that the decrease of NH3-N could vary depending on SCP, and the variation might be induced according to differences of glycofraction in SCP.
The elimination of ciliate protozoa is associated with a decrease in CH4 of up to 11% (De Newbold et al. Citation2015), and SCP supplementation can negatively affect protozoal count inducing the interaction between saponin and cholesterol in the protozoal membrane (Wina et al. Citation2005). In addition, the result from the previous meta-analysis study showed that increasing SCP levels significantly decrease CH4 production and protozoal count (Jayanegara et al. Citation2014). Therefore, we expected that AS addition would negatively affect the CH4 proportion in gas production by reducing protozoa. In the present study, a significant reduction in ciliate protozoa was detected in the FAS-treated groups, whereas there was no significant reduction in ciliate protozoa by dose levels and HAS supplementation. In spite of significant reduction in ciliate protozoa in FAS-treated groups, methanogenic archaea and CH4 proportion at 48 h was not decreaed. Similarly, Goel et al. (Goel et al. Citation2008) reported that a higher suppression of protozoa is not always linked to a higher reduction of methanogens and methanogenesis when several types of saponin-rich fractions were tested in an in vitro experiment. Pen, Sar (Pen et al. Citation2006) observed that the supplementation of liquid extracts of Yucca schidigera and Quillaja saponaria significantly decreased protozoal count, but a CH4 mitigation effect was only observed with the Y. schidigera treatment in a dose-dependent manner. Generally, plant extracts contain a higher fraction of bioactive compounds than the whole plant product; therefore, we speculated that the proportion of saponin in the AS used in this study is not adequate to decrease CH4 production. In addition, considering that only difference between HAS and FAS was drying method, the heat treatment might diminish the antiprotozoal efficacy of AS.
VFAs are a metabolic end-product produced by rumen microbes; therefore, changes in total and each VFA proportions imply a shift in the microbial population. Previously, several inconsistent results in total VFA concentration following SCP addition have been reported, including no changes (Sar et al. Citation2006, Citation2008; Goel et al. Citation2008; Makkar and Becker Citation1996), reductions (Lu and Jorgensen Citation1987; Lovett et al. Citation2006), and increases (Wang et al. Citation2000; Lila et al. Citation2003). In the present study, total VFA concentration was significantly increased regardless of dose levels and drying methods. Aloe generally consisted of cell wall carbohydrates (cellulose and hemicellulose) and storage carbohydrates (including mannan, arabinan, and arabinogalactan) as the main polysaccharides, which can be readily utilized by ruminal microbes (Ni et al. Citation2004). Therefore, one possible explanation for increased VFA concentration is the nutritional enhancement by AS supplementation. However, the general improvement of each microbe investigated in this study was more observable in the HAS-treated group than in the FAS-treated group. Previously, the freeze-drying method is more suitable for retaining phenolic compounds than the heat-drying method (Mahanom et al. Citation1999; Mediani et al. Citation2015; Park et al. Citation2021), thus the method for retaining antimicrobial compounds in AS might be the freeze-drying method. In addition, considering the increase of IVDMD in FAS-treated groups, the addition of FAS might positively affect the energy utilization of ruminal microbes.
The major alteration in rumen fermentation caused by SCP supplementation is the increased proportion of propionate, and the decreased proportion of acetate, thereby reducing CH4 production (Jayanegara et al. Citation2014). In the current study, the opposite changes in VFA proportions were detected from those previously reported, regardless of dose levels and drying methods. R. flavefaciens, R. albus, and F. succinogenes are the major ruminal fibrolytic bacteria (Mathieu et al. Citation1996). Among these, the absolute abundance of R. albus was significantly increased by AS supplementation regardless of drying methods; R. flavefaciens was also higher in the HAS-treated groups than in the CON group. Several previous studies have reported on the effects of SCP on ruminal cellulolytic bacteria. Guo et al. (Weimer Citation1993) reported that supplementation with triterpenoid saponin extracted from Camellia sinesis increased F. succinogenes. In addition, Muetzel (Guo et al. Citation2008) showed a significant increase in R. albus and R. flavefaciens by supplementing with Sesbania pachycarpa leaves. Considering this enhancement of cellulolytic bacteria, SCP might improve cellulolytic bacteria, but the impact on microbial species may vary depending on SCP type. The primary fermentation product of R. albus in the rumen is acetate (Muetzel Citation2003); thus, we speculated that the increased proportion of acetate observed in the current study is related to the enhancement of R. albus with AS supplementation.
In summary, FAS supplementation increased overall gas production, IVDMD, and total VFA concentration without a significant reduction in CH4 proportion. Regarding microbial abundance, both drying methods of AS supplementation enhanced the absolute abundance of fungi, P. ruminicola, and R. albus at least statistical tendency. The antiprotozoal efficacy of AS was only observed in FAS-treated groups, implying that heat treatment might damage the saponin in AS. Therefore, FAS may be an effective feed additive for ruminants, improving ruminal fermentation via enhancing feed utilization by rumen microbes. However, this study was conducted as in vitro method, therefore in vivo study will be needed to verify the effects of FAS on antiprotozoal effect and improvement of ruminal fermentation characteristics on ruminants.
Disclosure statement
No potential conflict of interest was reported by the author(s).
Additional information
Funding
References
- AOAC. 2005. Official methods of analysis. 18th ed. Gaithersburg (MD): Association of Official Analytical Chemists.
- Belanche A, Pinloche E, Preskett D, Newbold CJ. 2015. Effects and mode of action of chitosan and ivy fruit saponins on the microbiome, fermentation and methanogenesis in the rumen simulation technique. FEMS Microbiol Ecol. 92(1). doi:10.1093/femsec/fiv160.
- Bodas R, Prieto N, García-González R, Andrés S, Giráldez FJ, López S. 2012. Manipulation of rumen fermentation and methane production with plant secondary metabolites. Anim Feed Sci Technol. 176(1-4):78–93. doi:10.1016/j.anifeedsci.2012.07.010.
- Castillejos L, Calsamiglia S, Ferret A. 2006. Effect of essential oil active compounds on rumen microbial fermentation and nutrient flow in in vitro systems. J Dairy Sci. 89(7):2649–2658. doi:10.3168/jds.S0022-0302(06)72341-4.
- Chaney AL, Marbach EP. 1962. Modified reagents for determination of urea and ammonia. Clin Chem. 8(2):130–132.
- Choi SM, Supeno D, Byun JY, Kwon SH, Chung SW, Kwon SG, Kwon DY, Choi WS. 2015. The identification of saponin to obtain the maximum benefit from Aloe saponaria. Adv Sci Technol Lett. 120:558–563. doi:10.14257/astl.2015.120.112.
- Cieslak A, Zmora P, Stochmal A, Pecio L, Oleszek W, Pers-Kamczyc E, Szczechowiak J, Nowak A, Szumacher-Strabel M. 2014. Rumen antimethanogenic effect of Saponaria officinalis L. phytochemicals in vitro. The J Agric Sci. 152(6):981–993. doi:10.1017/S0021859614000239.
- Denman SE, McSweeney CS. 2006. Development of a real-time PCR assay for monitoring anaerobic fungal and cellulolytic bacterial populations within the rumen. FEMS Microbiol Ecol. 58(3):572–582. doi:10.1111/j.1574-6941.2006.00190.x.
- El Aziz M, Ashour A, Melad A. 2019. A review on saponins from medicinal plants: chemistry, isolation, and determination. J Nanomed Res. 8:6–12. doi:10.15406/jnmr.2019.07.00199.
- Gerber PJ, Steinfeld H, Henderson B, Mottet A, Opio C, Dijkman J, Falcucci A, Tempio G. 2013. Tackling climate change through livestock: a global assessment of emissions and mitigation opportunities. Rome: Food and Agriculture Organization of the United Nations (FAO).
- Goel G, Makkar H, Becker K. 2008. Changes in microbial community structure, methanogenesis and rumen fermentation in response to saponin-rich fractions from different plant materials. J Appl Microbiol. 105(3):770–777. doi:10.1111/j.1365-2672.2008.03818.x.
- Goering HK, Van Soest PJ. 1970. Forage fiber analyses: apparatus, reagents, procedures, and some applications: agricultural research service. Washington (DC): US Department of Agriculture.
- Gunun P, Gunun N, Khejornsart P, Ouppamong T, Cherdthong A, Wanapat M, Sirilaophaisan S, Yuangklang C, Polyorach S, Kenchaiwong W, et al. 2019. Effects of Antidesma thwaitesianum Muell. Arg. pomace as a source of plant secondary compounds on digestibility, rumen environment, hematology, and milk production in dairy cows. Anim Sci J. 90(3):372–381. doi:10.1111/asj.13147.
- Guo Y, Liu JX, Lu Y, Zhu W, Denman S, McSweeney C. 2008. Effect of tea saponin on methanogenesis, microbial community structure and expression of mcrA gene, in cultures of rumen micro-organisms. Lett Appl Microbiol. 47(5):421–426. doi:10.1111/j.1472-765X.2008.02459.x.
- Holtshausen L, Chaves AV, Beauchemin KA, McGinn SM, McAllister TA, Odongo NE, Cheeke PR, Benchaar C. 2009. Feeding saponin-containing Yucca schidigera and Quillaja saponaria to decrease enteric methane production in dairy cows. J Dairy Sci. 92(6):2809–2821. doi:10.3168/jds.2008-1843.
- Hu W-L, Liu J-X, Ye J-A, Wu Y-M, Guo Y-Q. 2005. Effect of tea saponin on rumen fermentation in vitro. Anim Feed Sci Technol. 120(3-4):333–339. doi:10.1016/j.anifeedsci.2005.02.029.
- Iannotti E, Kafkewitz D, Wolin M, Bryant M. 1973. Glucose fermentation products of Ruminococcus albus grown in continuous culture with Vibrio succinogenes: changes caused by interspecies transfer of H2. J Bacteriol. 114(3):1231–1240.
- Jayanegara A, Togtokhbayar N, Makkar HP, Becker K. 2009. Tannins determined by various methods as predictors of methane production reduction potential of plants by an in vitro rumen fermentation system. Anim Feed Sci Technol. 150(3-4):230–237. doi:10.1016/j.anifeedsci.2008.10.011.
- Jayanegara A, Wina E, Takahashi J. 2014. Meta-analysis on methane mitigating properties of saponin-rich sources in the rumen: influence of addition levels and plant sources. Asian-Australas J Anim Sci. 27(10):1426. doi:10.5713/ajas.2014.14086.
- Khafipour E, Li S, Plaizier JC, Krause DO. 2009. Rumen microbiome composition determined using two nutritional models of subacute ruminal acidosis. Appl Environ Microbiol. 75(22):7115–7124. doi:10.1128/AEM.00739-09.
- Khejornsart P, Cherdthong A, Wanapat M. 2021. In vitro screening of plant materials to reduce ruminal protozoal population and mitigate ammonia and methane emissions. Fermentation. 7(3):166. doi:10.3390/fermentation7030166.
- Kim H, Lee HG, Baek Y-C, Lee S, Seo J. 2020. The effects of dietary supplementation with 3-nitrooxypropanol on enteric methane emissions, rumen fermentation, and production performance in ruminants: a meta-analysis. J Anim Sci Technol. 62(1):31. doi:10.5187/jast.2020.62.1.31.
- Lila Z, Mohammed N, Kanda S, Kamada T, Itabashi H. 2003. Effect of sarsaponin on ruminal fermentation with particular reference to methane production in vitro. J Dairy Sci. 86(10):3330–3336. doi:10.3168/jds.S0022-0302(03)73935-6.
- Lovett D, Stack L, Lovell S, Callan J, Flynn B, Hawkins M, et al. 2006. Effect of feeding Yucca schidigera extract on performance of lactating dairy cows and ruminal fermentation parameters in steers. Livest Sci. 102(1-2):23–32. doi:10.1016/j.livsci.2005.11.005.
- Lu CD, Jorgensen NA. 1987. Alfalfa saponins affect site and extent of nutrient digestion in ruminants. J Nutr. 117(5):919–927. doi:10.1093/jn/117.5.919.
- Mahanom H, Azizah A, Dzulkifly M. 1999. Effect of different drying methods on concentrations of several phytochemicals in herbal preparation of 8 medicinal plants leaves. Malays J Sci. 5(1 & 2):47–54.
- Makkar HP, Becker K. 1996. Effect of Quillaja saponins on in vitro rumen fermentation. saponins used in food and agriculture: Springer; 1996. 387–94. doi:10.1007/978-1-4613-0413-5_33
- Mathieu F, Jouany J, Senaud J, Bohatier J, Bertin G, Mercier M. 1996. The effect of Saccharomyces cerevisiae and Aspergillus oryzae on fermentations in the rumen of faunated and defaunated sheep; protozoal and probiotic interactions. Reprod Nutr Dev. 36(3):271–287. doi:10.1051/rnd:19960305.
- Mediani A, Abas F, Khatib A, Tan CP, Ismail IS, Shaari K, Ismail A, Lajis NH. 2015. Relationship between metabolites composition and biological activities of Phyllanthus niruri extracts prepared by different drying methods and solvents extraction. Plant Foods Hum Nutr. 70(2):184–192. doi:10.1007/s11130-015-0478-5.
- Muetzel S. 2003. Supplementation of barley straw with Sesbania pachycarpa leaves in vitro: effects on fermentation variables and rumen microbial population structure quantified by ribosomal RNA-targeted probes. Br J Nutr. 89(4):445–453. doi:10.1079/BJN2002813.
- National Research Council. 2001. Nutrient requirements of dairy cattle: 2001. Washington (DC): National Academies Press.
- Newbold CJ, De La Fuente G, Belanche A, Ramos-Morales E, McEwan NR. 2015. The role of ciliate protozoa in the rumen. Front Microbiol. 6:1313. doi:10.3389/fmicb.2015.01313.
- Ni Y, Yates KM, Tizard IR. 2004. Aloe polysaccharides. Boca Raton: CRC Press.
- Niu H, Xu Z, Yang HE, McAllister TA, Acharya S, Wang Y. 2021. In vitro ruminal fermentation of fenugreek (Trigonella foenum-graecum L.) produced less methane than that of alfalfa (Medicago sativa). Anim Biosci. 34(4):584–593. doi:10.5713/ajas.20.0114.
- Ouwerkerk D, Klieve A, Forster R. 2002. Enumeration of Megasphaera elsdenii in rumen contents by real-time Taq nuclease assay. J Appl Microbiol. 92(4):753–758. doi:10.1046/j.1365-2672.2002.01580.x.
- Ozutsumi Y, Tajima K, Takenaka A, Itabashi H. 2006. Real-time PCR detection of the effects of protozoa on rumen bacteria in cattle. Curr Microbiol. 52(2):158–162. doi:10.1007/s00284-005-0266-9.
- Park CH, Yeo HJ, Park C, Chung YS, Park SU. 2021. The effect of different drying methods on primary and secondary metabolites in Korean mint flower. Agronomy. 11(4):698. doi:10.3390/agronomy11040698.
- Patra A, Park T, Kim M, Yu Z. 2017. Rumen methanogens and mitigation of methane emission by anti-methanogenic compounds and substances. J Anim Sci Biotechnol. 8(1):1–18. doi:10.1186/s40104-017-0145-9.
- Patra A, Stiverson J, Yu Z. 2012. Effects of Quillaja and Yucca saponins on communities and select populations of rumen bacteria and archaea, and fermentation in vitro. J Appl Microbiol. 113(6):1329–1340. doi:10.1111/j.1365-2672.2012.05440.x.
- Patra AK, Yu Z. 2014. Effects of vanillin, Quillaja saponin, and essential oils on in vitro fermentation and protein-degrading microorganisms of the rumen. Appl Microbiol Biotechnol. 98(2):897–905. doi:10.1007/s00253-013-4930-x.
- Pell A, Schofield P. 1993. Computerized monitoring of gas production to measure forage digestion in vitro. J Dairy Sci. 76(4):1063–1073. doi:10.3168/jds.S0022-0302(93)77435-4.
- Pen B, Sar C, Mwenya B, Kuwaki K, Morikawa R, Takahashi J. 2006. Effects of Yucca schidigera and Quillaja saponaria extracts on in vitro ruminal fermentation and methane emission. Anim Feed Sci Technol. 129(3-4):175–186. doi:10.1016/j.anifeedsci.2006.01.002.
- Pen B, Sar C, Mwenya B, Takahashi J. 2008. Effects of Quillaja saponaria extract alone or in combination with Yucca schidigera extract on ruminal fermentation and methanogenesis in vitro. Anim Sci J. 79(2):193–199. doi:10.1111/j.1740-0929.2008.00517.x.
- Schofield P, Pitt R, Pell A. 1994. Kinetics of fiber digestion from in vitro gas production. J Anim Sci. 72(11):2980–2991. doi:10.2527/1994.72112980x.
- Silva MA, Trevisan G, Klafke JZ, Rossato MF, Walker CIB, Oliveira SM, et al. 2013. Antinociceptive and anti-inflammatory effects of Aloe saponaria Haw on thermal injury in rats. J Ethnopharmacology. 146(1):393–401. doi:10.1016/j.jep.2012.12.055.
- Stevenson DM, Weimer PJ. 2007. Dominance of Prevotella and low abundance of classical ruminal bacterial species in the bovine rumen revealed by relative quantification real-time PCR. Appl Microbiol Biotechnol. 75(1):165–174. doi:10.1007/s00253-006-0802-y.
- Theodorou MK, Williams BA, Dhanoa MS, McAllan AB, France J. 1994. A simple gas production method using a pressure transducer to determine the fermentation kinetics of ruminant feeds. Anim Feed Sci Technol. 48(3-4):185–197. doi:10.1016/0377-8401(94)90171-6.
- Van Soest P, Robertson J, Lewis B. 1991. Methods for dietary fiber, neutral detergent fiber, and nonstarch polysaccharides in relation to animal nutrition. J Dairy Sci. 74(10):3583–3597. doi:10.3168/jds.S0022-0302(91)78551-2.
- Wallace R, Arthaud L, Newbold C. 1994. Influence of Yucca schidigera extract on ruminal ammonia concentrations and ruminal microorganisms. Appl Environ Microbiol. 60(6):1762–1767.
- Wanapat M, Phesatcha K, Viennasay B, Phesatcha B, Ampapon T, Kang S. 2018. Strategic supplementation of cassava top silage to enhance rumen fermentation and milk production in lactating dairy cows in the tropics. Trop Anim Health Prod. 50(7):1539–1546. doi:10.1007/s11250-018-1593-8.
- Wang R-F, Cao W-W, Cerniglia CE. 1997. PCR detection of Ruminococcus spp. in human and animal faecal samples. Mol Cell Probes. 11(4):259–265. doi:10.1006/mcpr.1997.0111.
- Wang Y, McAllister TA, Yanke LJ, Xu ZJ, Cheeke PR, Cheng KJ. 2000. In vitro effects of steroidal saponins from Yucca schidigera extract on rumen microbial protein synthesis and ruminal fermentation. J Sci Food Agric. 80(14):2114–2122. doi:10.1002/1097-0010(200011)80:14<2114::AID-JSFA755>3.0.CO;2-0.
- Weimer PJ. 1993. Microbial and molecular mechanisms of cell wall degradation—session synopsis. Forage Cell Wall Struct Dig. 485–498. doi:10.2134/1993.foragecellwall.c19.
- Whelan JA, Russell NB, Whelan MA. 2003. A method for the absolute quantification of cDNA using real-time PCR. J Immunol Methods. 278(1-2):261–269. doi:10.1016/S0022-1759(03)00223-0.
- Williams AG, Coleman GS. 2012. The rumen protozoa. New York (NY): Springer Science & Business Media.
- Wina E, Muetzel S, Becker K. 2005. The impact of saponins or saponin-containing plant materials on ruminant production A review. J Agric Food Chem. 53(21):8093–8105. doi:10.1021/jf048053d.
- Xie KL, Wang ZF, Guo YR, Zhang C, Zhu WH, Hou FJ. 2022. Gentiana straminea supplementation improves feed intake, nitrogen and energy utilization, and methane emission of Simmental calves in northwest China. Anim Biosci. 35(6):838–846. doi:10.5713/ab.21.0263.
- Yu Z, Morrison M. 2004. Improved extraction of PCR-quality community DNA from digesta and fecal samples. Biotechniques. 36(5):808–812. doi:10.2144/04365ST04.