ABSTRACT
One of the most important drivers of the emergence of antimicrobial resistance is the irrational usage of antibiotics. But alongside some indirect sources such as feeding Chinese corn stover silage could also transmit resistant genes to animals. This study was aimed at assessing the antibiotic resistance phenotypes, drug resistance genes and mediated drug resistance mechanisms. A total of 37 isolates were obtained by selective medium and identified by 16S rDNA sequencing including Lactobacillus acidophilus (n = 3), Lactobacillus amylovorus (n = 4), Weissella confusa (n = 3), Acetobacter pasteurianus (n = 9), Lactobacillus buchneri (n = 6), Enterococcus faecium (n = 8) and Lactobacillus reuteri (n = 4). Antimicrobial resistance of all isolates to 12 antibiotics was determined using the agar dilution method. Widespread resistance to ampicillin, erythromycin, clindamycin, kanamycin, streptomycin, levofloxacin and ciprofloxacin was observed. The presence of relevant resistance genes was examined by PCR, and the genes pbp5, blaZ, bla TEM, mecA, mexI, tetW, ermB, msrA, msrC, ermC, vatE, aacA-aphD, aphA1, aadA1, aadA2 and vanX were detected. All the isolated strains had multiple drug resistance, and harboured related drug-resistant genes; therefore, the use of probiotics in animal feed should be standardized to reduce the risks of horizontal transmission of drug resistance genes to animal products and human population.
Highlights
Fermentation bacteria isolated from the silage were identified.
Lactobacillus strains isolated from the silage exhibited antimicrobial resistance.
Feeding Chinese corn stover silage transmits resistant genes to animals.
Standardization of probiotics in animal feed is crucial to avoid horizontal drug resistance.
Introduction
Globally, silage is one of the most important major feeds for ruminants (Avila and Carvalho Citation2019). Silage is a kind of juicy feed which is soft, juicy, easy to digest, nutritious, palatable and storage-resistant after the fresh green fodder is properly processed and fermented by microorganisms (Markowiak and Slizewska Citation2018). Microorganisms play a vital role in the silage fermentation process. The main microorganisms involved in the silage fermentation process are lactic acid bacteria (Zielińska et al. Citation2015; Bao et al. Citation2016). Among the fermentation bacteria, lactobacilli are deemed to have long history of safe usage in feed industry as feed additives and have commonly been used as animal growth promoters, probiotics and forage ensiling as well. Furthermore, these bacteria are considered that improve quality and safety of the feed (Pararajasingam and Uwagwu Citation2017). Likewise, many of the lacotobacilli species works as resident microbiota in the animal’s body and exert beneficial effects on the animal’s heath (Jahan and Holley et al. Citation2016). The pathogenic bacteria causing food spoilage and foodborne diseases can also be inhibited by these lactobacilli.
With the deepening global research on probiotics, the popularity of probiotic products has been promoted. However, due to lack of strict safety standards for probiotics usage, a series of potential risks have been triggered (Pang et al. Citation2012). Incorporation of inferior probiotics supplements in the animals feed may lead to the transmission of antibiotic-resistant genes to common pathogenic bacteria and can induce drug resistance (Jahan and Holley Citation2016; Zielinska et al. Citation2017). In this regard, food chain is one of the most important ways for antibiotic-resistant bacteria to spread between animals and humans. A large number of microorganisms enter animals through silage without sterilization; it may transmit resistance genes through the food chain to other intestinal and respiratory pathogens, and as such poses a threat to the livestock industry and human population (Pang and Qin Citation2011).
Lactobacilli have been recognized as silage additives and gut microbiota stabilizers and have got great interest in the application in feed industry due to their growth-promoting abilities (Fanfan et al. Citation2019). Their effectiveness has been shown in poultry, piglets, fish and silage industries (Guo et al. Citation2017). Likewise, many strains of lactobacilli have been considered to produce substances like bacteriocines that inhibit pathogen activities (Jaimee and Halami Citation2016). Controversy remains for Enterococcus spp. strains as human probiotics but they are used as probiotics and silage additives in animals for stabilizing microbial communities of the gastrointestinal tracts (Li and Li Citation2015). The scientists have focused the search for new Lactobacillus strains having safety and beneficial health properties as probiotics. Most of the commonly used lactobacilli have been generally regarded as safe as per guidelines of the US Food and Drug Administration (FDA) and the European Food Safety Authorities (EFSA). The Panel of Biological Hazards have also considered these as safe for environment and allow their usage in animal consumption (Dincer and Kivanc Citation2019). Mostly lactobacilli have been regarded as safe to be used as feed additives but still few infections like pleuropneumonia (Ben Braïek and Smaoui Citation2019; Jiang et al. Citation2021), urinary tract infections (Florescu et al. Citation2008), endocarditis (Holmberg et al. Citation2017), meningitis (Chen et al. Citation2018) and bacteraemia (Groga-Bada et al. Citation2018) have been reported to be caused due to their usage. The main individuals exposed to these infections include immunocompromised, elderly person, premature newborn individuals and people with serious underlying illnesses (Datta et al. Citation2017; Biesiada et al. Citation2019). Limited literature is available regarding the role of lactobacilli in animal infections, although the genus Lactococcus can be associated with infections in fish and birds and bovine mastitis but up to date no reports have validated Lactobacillus infections in animals (Citla and Gourishankar Citation2013).
Despite of many beneficial effects of lactobacilli there is a serious concern of acquired resistance to antimicrobials (Plumed-Ferrer et al. Citation2015). This issue has become a research hotspot for the scientists because these lactobacilli work as reservoirs for resistance genes that can be easily transmitted to pathogenic bacteria, leading to development of antibiotic resistance among bacteria resulting in the treatment failure during disease outbreaks (Torriani et al. Citation2001). Keeping in view this safety issue, it is imperative to evaluate the probiotics while selecting as feed additives. These safety issues must be in line with the guidelines provided by different internationally recognized regulations on the feed additives (Ward and Timminis Citation1999; Klare et al. Citation2005). The Panel on Additives and Products or Substances used Animal Feed (FEEDAP) has restricted the use of feed additives of unknown genetic nature carrying antimicrobial resistance to avoid risks of horizontal transmission (Aminov et al. Citation2001).
The aim of this study was to analyse and evaluate the antibiotic resistance of probiotics isolated from Chinese corn stover silage, and to analyse their antibiotic resistance phenotypes, detect drug resistance genes and mediated drug resistance mechanisms, in order to provide a reference for the safety assessment of lactic acid bacteria and quality control of silage.
Materials and methods
Sample collection and isolation of bacterial strains
A total of 13 corn stover silages were collected from the Jilin province in China. Isolation of lactobacillus was performed as described by National Food Safety Standard-Food microbiological examination-lactobacillus test (GB 4789.35-2016). A 10 g each silage sample out of 13 collected samples was blended with 50 mL of sterile saline (0.9% NaCl) and a 10-fold dilution in saline was prepared after intensive shaking (101–108). Then 1 mL of each dilution was mixed with 20 mL of cabbage agar (CA) containing 3% CaCO3 and plated (Composition of CA: cabbage 200 g, glucose 20 g, peptone 10 g, agar 20 g, water 1000 mL). The plates were subjected to incubation at 37°C for 48 h. The bacterial colonies forming clear zones of CaCO3 hydrolysis were considered as putative LAB and were individually picked and streaked on MRS agar plates by dilution streaking. The plates were incubated at 37°C for 24 h in order to obtain a single colony and subsequently subjected to Gram staining and catalase test. The isolates were used either immediately or preserved at −80°C in 50%. Lactobacillus plantarum 8PA3 was as a reference strain. A total of 37 strains namely Lactobacillus acidophilus (n = 3), Lactobacillus amylovorus (n = 4), Weissella confusa (n = 3), Acetobacter pasteurianus (n = 9), Lactobacillus buchneri (n = 6), Enterococcus faecium (n = 8) and Lactobacillus reuteri (n = 4) were isolated for this study.
DNA extraction
The total DNA was extracted using Ezup Column Bacteria Genomic DNA Purification Kit (Sangon Biotech, Shanghai, China), and 16S rDNA was amplified by PCR. Thermal cycling consisted of an initial denaturation at 95°C for 5 min, followed by 30 cycles of denaturation at 95°C for 1 min, annealing at 60°C for 1 min and extension at 72°C for 1 min, with a final extension at 72°C for 10 min. The PCR components were also obtained from Sangon Biotech, Shanghai, China. The reaction was performed in 25 μL mixture with 16S rDNA primers as described previously (Nawaz et al. Citation2011).
Determination of antimicrobial resistance
Agar dilution method was used to determine the drug sensitivity of the isolated strains to 12 antibiotics on LSM agar according to CLSI, including ceftriaxone, cefuroxime, ampicillin, chloramphenicol, clindamycin, tetracycline, erythromycin, kanamycin, streptomycin, gentamicin, levofloxacin and ciprofloxacin. The microbiological breakpoints to antibiotics were based on the European Food Safety Authority (EFSA Citation2017), while for antibiotics not included in EFSA referred to the CLSI (Citation2016), as shown in . The reference strain E. faecium ATCC 29212 served as a quality control. The lowest concentration of antibiotics that inhibited visible bacterial growth at 37°C incubation was termed as minimum inhibitory concentration. The microbiological cut-off values defined by the EFSA Panel of Additives and Products or Substances used in Animal Feed were adopted for determination of strains susceptibility (EFSA). Parallel use of the reference strain E. faecium ATCC 29212 was monitored for accuracy of the antimicrobial susceptibility. Experimental procedures were performed in triplicate.
Table 1. Breakpoints of drug sensitivity of Lactobacillus.
Determination of resistant genes
PCR amplifications were performed for detection of resistance genes according to drug resistance phenotype, including β-lactam antibiotics (pbp5, blaZ, blaTEM and mecA), tetracycline (tetW, tet(38), mexI, tetE and tetT), MLSB (ermB, vatE, msrC, ermC, lnua01, msrA, vgaA and vgaB), aminoglycoside (aacA-aphD, aphA1, aadA1, aadD, aac(2’)-Ic and aadA2) and vancomycin (vanX and vantC). The PCR-specific primers and amplification conditions are listed in . The PCR products were separated by 1% agarose gel electrophoresis, and the positive products were sequenced by Sangon Biotech Co. Ltd. (Shanghai, China), and the sequencing was compared with NCBI BLAST database.
Table 2. Gene specific primers and conditions for polymerase chain reaction detection.
Statistical analysis
Graphs were plotted and appropriate statistically significant differences were assessed mostly by Chi-square tests using graph pad prism software 8.4.2 (679) version. The differences were significant statistically when p-value < .005, and p = ****, then the p-value < .0001, whereas ns indicates non-significant.
Results
A total of 37 bacterial strains were isolated from 13 corn stover silages in the Jilin Province, China. The isolated strains were categorized into seven species as follows: L. acidophilus (n = 3), L. amylovorus (n = 4), W. confusa (n = 3), A. pasteurianus (n = 9), L. buchneri (n = 6), E. faecium (n = 8) and L. reuteri (n = 4).
Distribution of antimicrobial resistance
The minimum inhibitory concentrations (MICs) of all 37 strains to 12 antibiotics, divided into sensitive and resistant strains according to the recommended breakpoints are shown in – and . All the isolated strains, except E. faecium, were susceptible to cefuroxime and ceftriaxone. All the L. acidophilus were sensitive to ampicillin, clindamycin and chloramphenicol, while resistant to kanamycin, streptomycin, levofloxacin and ciprofloxacin. Only AP13-2 strain was sensitive to tetracycline, erythromycin and gentamicin. Furthermore, all the four strains of L. amylovorus were resistant to tetracycline, streptomycin and levofloxacin, and highly resistant to erythromycin (≥512 μg/ml). Similarly, all the three strains of W. confusa were resistant to clindamycin, kanamycin streptomycin and ciprofloxacin. A total of seven out of nine strains of A. pasteurianus were resistant to erythromycin and chloramphenicol, both levofloxacin and ciprofloxacin shown resistance to all of the nine A. pasteuricum strains. All of the L. buchneri were resistant to ampicillin and ciprofloxacin. Likewise, all of the E. faecium showed high MICs to ceftriaxone, cefuroxime, kanamycin and streptomycin (MIC ≥ 64 μg/mL), while resistance with comparatively low MICs to ampicillin (MIC = 8 μg/mL) and gentamicin (MIC = 32 μg/mL). All of the four L. reuteri were resistant to ampicillin, chloramphenicol, tetracycline, erythromycin, streptomycin and gentamicin. The tested strains showed multidrug resistance. A total of 24.3% isolates showed resistance to three to five antibiotics, 72.9% isolates showed resistance to six to eight antibiotics and only L. amylovorus showed resistance to nine antibiotics.
Figure 1. Strains of L. acidophilus showing resistance and sensitivity against the selected antimicrobials. Data were analysed through Chi-Square test, statistically significant (P < .05), whereas ns indicates non-significant.
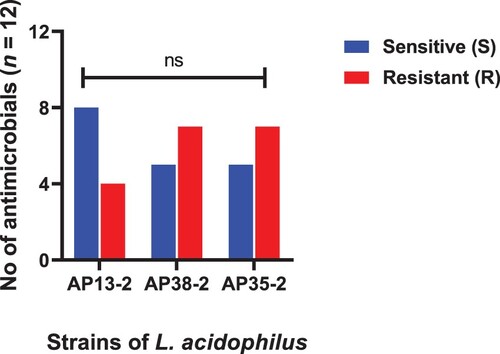
Figure 2. Strains of L. amylovorus showing resistance and sensitivity against the selected antimicrobials. Data were analysed through Chi-Square test, statistically significant (P < .05), whereas ns indicates non-significant.
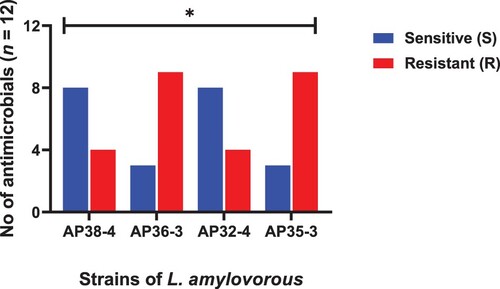
Figure 3. Strains of W. confuse showing resistance and sensitivity against the selected antimicrobials. Data were analysed through Chi-Square test, statistically significant (P < .05) whereas ns indicates non-significant.
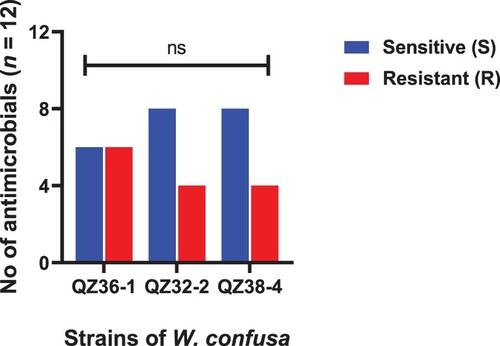
Figure 4. Strains of A. pasteurianus showing resistance and sensitivity against the selected antimicrobials. Data were analysed through Chi-Square test, statistically significant (P < .05), whereas ns indicates non-significant.
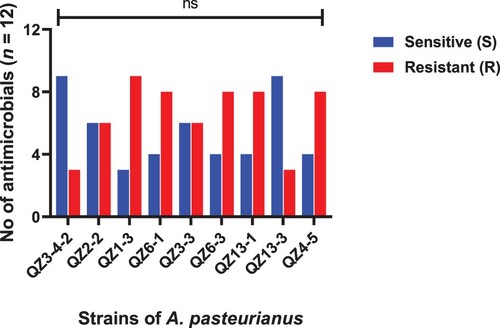
Figure 5. Strains of L. buchneri showing resistance and sensitivity against the selected antimicrobials. Data were analysed through Chi-Square test, statistically significant (P < .05), whereas ns indicates non-significant.
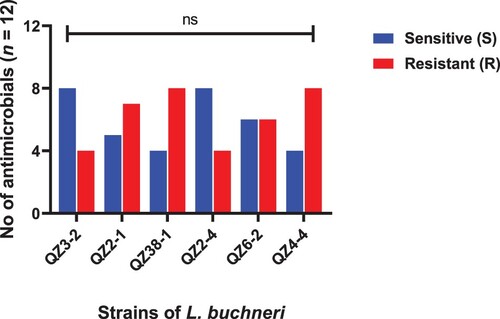
Figure 6. Strains of E. faecium showing resistance and sensitivity against the selected antimicrobials. Data were analysed through Chi-Square test, statistically significant (P < .05), whereas ns indicates non-significant.
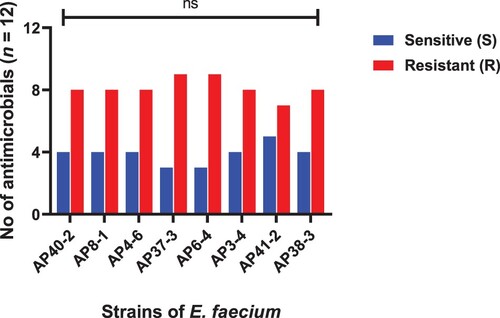
Figure 7. Strains of L. reuteri showing resistance and sensitivity against the selected antimicrobials. Data were analysed through Chi-Square test, statistically significant (P < .05, whereas ns indicates non-significant.
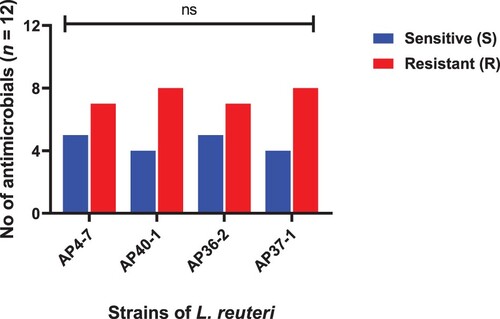
Figure 8. Comparisons of susceptibility (S) and resistance (R) of antimicrobial against MDR isolates (A) Ceftriaxone, (B) Cefuroxime, (C) Ampicillin, (D) Chloramphenicol, (E) Clindamycin, (F) Tetracycline, (G) Erythromycin, (H) Kanamycin, (I) Streptomycin, (J) Gentamicin, (K) Levofloxacin, (L) Ciprofloxacin. P-value was analysed through Chi-square test and was statistically significant, when p-value = **** = <.0001, whereas ns indicates non-significant.
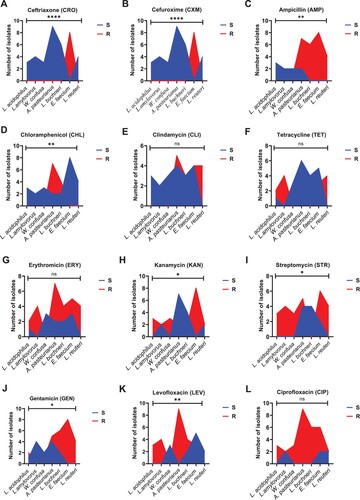
Table 3. L. acidophilus strains sensitivity and resistance against selected antibiotics.
Table 4. L. amylovorus strains sensitivity and resistance against selected antibiotics.
Table 5. W. confusa strains sensitivity and resistance against selected antibiotics.
Table 6. A. pasteurianus strains sensitivity and resistance against selected antibiotics.
Table 7. L. buchneri strains sensitivity and resistance against selected antibiotics.
Table 8. E. faecium strains sensitivity and resistance against selected antibiotics.
Table 9. L. reuteri strains sensitivity and resistance against selected antibiotics.
Distribution of antimicrobial resistance genes
All the isolates were tested for antimicrobial resistance genes by PCR amplifications based on the MICs. The antimicrobial resistance genes detected in the resistance phenotype for all strains are shown in . The gene tetW encode tetracycline ribosomal protective protein conferring resistance to tetracycline was detected in 12 out of 16 tetracycline-resistant, and 9 tetracycline-sensitive isolates. The mexI gene encode antibiotic efflux pump, conferring resistance to tetracycline and fluoroquinolone was detected in five isolates resistant to tetracycline or quinolone. The ermB produced antibiotic target alteration, which induced resistance to erythromycin, lincomycin and streptogramin B (MLSB) was found in 32 (86.5%) isolates. The genes msrC and msrA encoded target antibiotic action sites and conferred protection from antibiotic binding, were observed among 15 and 4 strains, respectively. Related resistance genes were present in all β-lactam-resistant strains, except four isolates. The aadA1, aacA-aphD and aadA2 genes, responsible for the aminoglycoside were found in most of the aminoglycoside resistant strains. The vancomycin resistance genes were detected in 17 (45.9%) strains. Other resistance genes (tetE, tetT, tet(38), ermC, luna01, vgaA, vgaB, aadD, aac(2’)-lc and vantC) were not identified in any of the resistant strains. Some strains had been detected for resistance genes but had no corresponding resistance phenotype, this may be because the gene was not expressed at the RNA level or a mutation in the regulatory region may have resulted in the open reading frame not being expressed.
Table 10. Characteristics of 37 multidrug-resistance isolates.
Discussion
Corn stover silage is an increasingly important ruminant feed in China and the microbes plays an important role in the silage fermentation. In our study, a total of 37 Lactobacillus strains were isolated from the corn stover silages including L. buchneri, L. acidophilus, W. confusa, A. pasteurianus, E. faecium, L. amylovorus and L. reuteri. Lactic acid bacteria are the main microorganisms in the fermentation process (Toomey et al. Citation2010). At present, there are two kinds of lactic acid bacteria developed and utilized: homo-type lactic acid fermenting bacteria and hetero-type lactic acid fermenting bacteria. Lactobacillus reuteri, Lactobacillus brucei and W. confusa belong to heteromorphic lactic acid fermenting bacteria. Although heteromorphic lactic acid fermenting bacteria consume more energy, and their metabolites and volatile fatty acids are beneficial to improve the aerobic stability of silage (Hummel et al. Citation2007). Lactobacillus buchneri is able to anaerobically convert sugars into acetic acid and 1,2-propanediol, and acetic acid, and can improve the aerobic stability of silage by inhibiting the growth of spoilage bacteria (Vakulenko et al. Citation2003; Ouoba et al. Citation2008). Weissella cibaria and W. confusa are mainly present on the corn surface. Weissella confusa plays an important role in the early fermentation of silage, but studies have shown that the spoilage had little effect on improving the quality of silage (Chang et al. Citation2011; Klose et al. Citation2014). Lactobacillus reuteri has tolerance to gastrointestinal environment, and has certain tolerance to artificial gastric juice, intestinal juice and bile salts. Lactobacillus acidophilus, Lactobacillus amylophilus and E. faecium are homo-type lactic acid fermenting bacteria (Dec et al. Citation2017). The homo-type fermentation can rapidly reduce pH, increase the number of lactic acid bacteria and lactic acid content, and maintain the quality and nutritional value of the silage raw materials (Dec et al. Citation2018). Acetobacter pasteurianus is an unconventional bacterium in silage which converts ethanol to acetic acid under aerobic conditions, consume oxygen at the early stage of fermentation which promotes the formation of anaerobic environment and reduces pH to provide conditions for the growth and reproduction of lactic acid bacteria (Aquilanti et al. Citation2007). All the strains isolated in this study have been reported.
One of the key criterions that must be met prior to intentional incorporation of lactic acid bacteria into food chain is antimicrobial susceptibility. A great variation in susceptibility to antimicrobial exists among lactic acid bacteria. The spread of bacterial resistance to antibiotics through the food chain is a serious public safety concern. Owing to the increasing use of lactic acid bacteria as probiotics food starter, the antibiotic resistance of these bacteria and the potential risks of horizontal transmission of antibiotic resistance genes have received increasing attention. Lactic acid bacteria are generally regarded as safe, but recent reports suggest that some of the lactic acid bacteria may be pathogenic. Therefore, the resistance of lactic acid bacteria should be characterized prior to use.
The resistance observed in our study for ceftriaxone and cefuroxime was comparatively low and shown a reasonable susceptibility towards all experimental strains except E. faecium. The resistance observed for both of these antibiotics was from total of 8 out of 37 experimental isolates. The results obtained for ceftriaxone and cefuroxime were highly significant. Our results are consistent with the previous reports provided for drug resistance of Lactobacillus species obtained from different sources (Dec et al. Citation2017, Citation2020; Anisimova et al. Citation2019). The experimental isolates also revealed a significant result in terms of sensitivity and resistance to Ampicillin. But the rate of significance was low as compared to ceftriaxone and cefuroxime. A number of previous data are in line with the current findings of our study indicating significant results for susceptibility and resistance of Lactobacillus species to ampicillin (Danielsen et al. Citation2003; Nawaz et al. Citation2011; Anisimova et al. Citation2019). Some of the researchers have obtained lactobacillus from poultry and other products and have stated comparatively higher resistance of Lactobacillus than our findings to ampicillin (Dec et al. Citation2017; Dec et al. Citation2018; Erginkaya et al. Citation2018). Likewise, majority of the experimental isolates shown susceptibility to chloramphenicol and revelled a low statistical significance. Similar results have been observed by other scientists in regard of susceptibility of Lactobacillus species to chloramphenicol which support our findings (Danielsen et al. Citation2003; Anisimova et al. Citation2019; Dec et al. Citation2017; Dec et al. Citation2020). In contrast, some reports validate resistance to chloramphenicol in Lactobacillus species (Sukmarini et al. Citation2014; Erginkaya et al. Citation2018). Some experimental isolates shown resistance to Clindamycin and tetracycline but as a whole the results were statistically non-significant for both of these antibiotics. Opposite to our findings, some reports have mentioned significant and higher rate of resistance among different Lactobacillus species to these antibiotics (Cauwerts et al. Citation2006; Sukmarini et al. Citation2014; Dec et al. Citation2017, Citation2018; Erginkaya et al. Citation2018). Numerous studies have shown that lactic acid bacteria are usually sensitive to antibiotics that inhibit protein synthesis, such as chloramphenicol, erythromycin, clindamycin and tetracycline, but these are sensitive to cell wall synthesis inhibitors such as b-lactam antibiotics as well (Cauwerts et al. Citation2006). Lactobacillus is generally resistant to aminoglycoside antibiotics such as kanamycin and streptomycin because of the membrane impermeability (Danielsen and Wind Citation2003; Flórez et al. Citation2006). In aminoglycosides group kanamycin and tetracycline shown a low significant resistance while gentamycin revealed a non-significant result. Persistent to our findings a low pattern of resistance to gentamicin has been reported for the Lactobacillus species obtained from turkeys (Dec et al. Citation2017, Citation2018) but reportedly higher and significant rate of resistance to gentamicin also exists (Erginkaya et al. Citation2018). Likewise, majority of the isolates exhibited resistance to erythromycin but a whole the statistical results were non-significant in our study. In contrast, some scientists have reported significant results for erythromycin in terms of resistance (Cauwerts et al. Citation2006; Dec et al. Citation2017, Citation2018). The results obtained for levofloxacin in terms of resistance were significant and higher than that of kanamycin and streptomycin. Ciprofloxacin also exhibited resistance to most of the isolates but as whole statistical analysis for ciprofloxacin was non-significant. We found that Lactobacillus had a high resistance rate to kanamycin (59.4%), streptomycin (72.9%) and gentamicin (64.8%). However, the isolates showed low concentration range as well, compared with streptomycin and kanamycin (2–64 μg/mL), probably because of better membrane penetration (Anisimova and Yarullina Citation2019). The incidence of resistance of the strains was 67% for levofloxacin and 81% for ciprofloxacin. Similarly, Lactobacillus species were resistant to ciprofloxacin as described by other researcher (Sukmarini et al. Citation2014). The main mechanism of bacterial resistance to quinolones is the mutation of DNA cyclotron and topoisomerase-IV genes, which causes the mutation of enzyme subunits and reduces the sensitivity of bacteria to quinolones (Campedelli et al. Citation2018).
In this study, the resistance rate to tetracycline was 40.5%, and tetW gene was detected in most of the tetracycline-resistant isolates. The resistance to tetracycline was due to the presence of tetW gene in 12 tetracycline-resistant isolates because it encode tetracycline ribosomal protective protein. A total of five isolates resistant to quinolone and tetracycline revealed mexI gene responsible for encoding antibiotic efflux pump and conferred resistance to ciprofloxacin, levofloxacin and tetracycline. Some recent studies have reported the presence of the gene tetW in L. helvetius, L. plantarum and E. faecium (Bansal et al. Citation2016; von Wintersdorff et al. Citation2016). Other genes such as tetK, tetQ, tetM, tetK, tetL, tetO and tetS have also been found in Lactobacillus (Zheng et al. Citation2020). The ermB gene was present in a total of 32 isolates responsible for producing antibiotic target alteration and thus conferred resistance to clindamycin, streptomycin and erythromycin. Some reports have given similar results and stated that 88% of the total isolates harboured the ermB gene (Mayrhofer et al. Citation2014). Some genes had conferred protection from antibiotic binding through encoding target antibiotic action sites including msrC gene found in 15 isolates and msrA gene founded in four isolates. Erythromycin and clindamycin share a common mechanism of resistance. The genes msrC and msrA encode target antibiotic action sites and confer protection from antibiotic binding. The genes aadA1, aadA2 and aphA1 produced aminoglycoside antibiotic inactivation. The genes responsible for aminoglycoside resistance including aadA1, aacA-aphD and aadA2 genes were found in all isolates resistant to aminoglycosides. Compared to kanamycin and streptomycin, gentamicin has shown very effective inhibition at the low-end concentration range (0.002–1 μg/mL), possibly because this aminoglycoside is able to cross the cell membrane better than other aminoglycosides, thus resulting in lower MICs of this antibiotic (Erginkaya et al. Citation2018). The emergence of aac(6’)Ie-aph(2’’)Ia, aph(3’)IIIa, aad6, aadA and aph(3’)IIIa among Lactobacillus isolated from farm animals also reminds people of the forthcoming hazard of food-based probiotics (Dec et al. Citation2020). The target gene mutation of quinolone action was not detected in this study. The vanX gene encodes the enzyme D-ala-D-ala dipeptidase endows lactic acid bacteria with intrinsic resistance to vancomycin (Fernández et al. Citation2012)
Conclusions
Multiple drug resistance was observed for all the tested isolates and the relevant resistance genes were detected. Therefore, based on our findings these strains cannot be considered as safe for animals and human usage and alternative measures should be brought into consideration. The obtained data can be used for subsequent experiments in order to provide a reference for the safety assessment of lactic acid bacteria and quality control of the silage. Through which the risks of horizontal transmission of drug resistance genes to animal products and human population can be reduced up to many extent. This study suggests that the first and utmost step while application of lactic acid bacteria is animal feed is the antimicrobial resistance assessment.
Disclosure statement
No potential conflict of interest was reported by the author(s).
Additional information
Funding
References
- Aminov RI, Garrigues-Jeanjean N, Mackie RI. 2001. Molecular ecology of tetracycline resistance: development and validation of primers for detection of tetracycline resistance genes encoding ribosomal protection proteins. Appl Environ Mic. 67:22–32.
- Anisimova EA, Yarullina DR. 2019. Antibiotic resistance of Lactobacillus strains. Curr Mic. 76:1407–1416. doi:10.1007/s00284-019-01769-7
- Aquilanti L, Garofalo C, Osimani A, Silvestri G, Vignaroli C, Clementi F. 2007. Isolation and molecular characterization of antibiotic-resistant lactic acid bacteria frompoultry and swine meat products. J Food Prot. 70:557–565.
- Avila CLS, Carvalho BF. 2019. Silage fermentation- updates focusing on the performance of micro-organisms. J App Mic. 128:966–984.
- Bansal S, Mangal M, Sharma SK, Gupta RK. 2016. Non-dairy based probiotics: healthy treat for intestine. Crit Rev Food Sci Nutr. 56:1856–1867.
- Bao W, Mi Z, Xu H. 2016. Assessing quality of Medicago sativa silage by monitoring bacterial composition with single molecule, real-time sequencing technology and various physiological parameters. J Sci Rep. 6:28358.
- Ben Braïek O, Smaoui S. 2019. Enterococci: between emerging pathogens and potential probiotics. Biomed Res Int. 23:5938210.
- Biesiada G, Krycińska R, Czepiel J, Stazyk K, Kedzierska J, Garlicki A. 2019. Meningoencephalitis caused by Lactobacillus plantarum – case report. Int J Neurosci. 129:715–718.
- Campedelli I, Mathur H, Salvetti E, Clarke S, Rea MC, Torriani S. 2018. Genuswide assessment of antibiotic resistance in Lactobacillus spp. Appl Environ Mic. 85:e01738–18.
- Cauwerts K, Pasmans F, Devriese LA, Haesebrouck F, Decostere A. 2006. Cloacal Lactobacillus isolates from broilers often display resistance toward tetracycline antibiotics. Microb Drug Resist. 12:284–288.
- Chang YC, Tsai CY, Lin CF, Wang YC, Wang IK, Chung TC. 2011. Characterization of tetracycline resistance lactobacilli isolated from swine intestines at western area of Taiwan. Anaerobe. 17:239–245.
- Chen F, Zhang Z, Chen J. 2018. Infective endocarditis caused by Lactococcus lactis subsp. lactis and Pediococcus pentosaceus: a case report and literature review. Medicine (Baltimore). 97:13658.
- Citla SD, Gourishankar A. 2013. Lactobacillus causing urinary tract infection in a neonate. J Med Cases. 4:682–685.
- Clinical and Laboratory Standards Institute (CLSI). 2016. Performance standards for antimicrobial susceptibility testing. 26th Edition CLSI Supplement M100S. CLSI, Wayne, PA, USA.
- Danielsen M, Wind A. 2003. Susceptibility of Lactobacillus ssp. to antimicrobial agents. Int J Food Microbiol. 82(1):1–11.
- Datta P, Gupta V, Mohi GK, Chander J, Janmeja AK. 2017. Lactobacillus coryniformis causing pulmonary infection in a patient with metastatic small cell carcinoma: case report and review of literature on Lactobacillus pleuro-pulmonary infections. J Clin Diagn Res. 11:DE01–DE05. doi:10.7860/JCDR/2017/22837.9391.
- Dec M, Nowaczek A, Stępień-Pyśniak D, Wawrzykowski J, Urban-Chmiel R. 2018. Identification and antibiotic susceptibility of lactobacilli isolated from turkeys. J BMC Mic. 18:168.
- Dec M, Stępień-Pyśniak D, Nowaczek A, Puchalski A, Urban-Chmiel R. 2020. Phenotypic and genotypic antimicrobial resistance profiles of fecal lactobacilli from domesticated pigeons in Poland. Anaerobe. 65:102251.
- Dec M, Urban-Chmiel R, Stępień-Pyśniak D, Wernicki A. 2017. Assessment of antibiotic susceptibility in Lactobacillus isolates from chickens. Gut Pathog. 9:54.
- Dincer E, Kivanc M. 2019. Characterization of Lactobacillus plantarum strains isolated from Turkish pastirma and possibility to use of food industry. Food Sci Technol. 40:498–507.
- EFSA. 2017. Scientific opinion on the update of the list of QPS-recommended biological agents intentionally added to food or feed as notified to EFSA. EFSA J. 9(1):1–16.
- Erginkaya Z, Turhan EU, Tatli D. 2018. Determination of antibiotic resistance of lactic acid bacteria isolated from traditional Turkish fermented dairy products. Iran J Vet Res. 19:53–56.
- Fanfan Z, Xuzhe W, Weihua L. 2019. Improved quality of corn silage when combining cellulose-decomposing bacteria and Lactobacillus buchneri during Silage Fermentation. BioMed Res Int. 11:4361358.
- Fernández M, Conde S, de la Torre J, Molina-Santiago C, Ramos JL, Duque E. 2012. Mechanisms of resistance to chloramphenicol in Pseudomonas putida KT2440. Antimicrob Agents Chemother. 56:1001–1009.
- Florescu D, Hill L, Sudan D, Iwen PC. 2008. Leuconostoc bacteremia in pediatric patients with short bowel syndrome: case series and review. Pediatr Infect Dis J. 27:1013–1019.
- Flórez AB, Ergevarn M, Danielsen M, Tosi L, Morelli L, Lingren S. 2006. Susceptibility of Lactobacillus plantarum strain to six antibiotics and definition of new susceptibility-resistance cutoff values. Microbial Drug Resist. 12:252–256.
- Groga-Bada P, Mueller I, Foschi F, Gawaz M, Eick C. 2018. Mitral valve endocarditis due to Lactobacillus. Case Rep Med. 8613948.
- Guo HL, Pan L, Li L. 2017. Characterization of antibiotic resistance genes from Lactobacillus isolated from traditional dairy products. J Food Sci. 82:724–730.
- Holmberg P, Hellmich T, Homme J. 2017. Pediatric sepsis secondary to an occult dental abscess: a case report. J Emerg Med. 52:744–748.
- Hummel A, Holzapfel WH, Franz CMAP. 2007. Characterisation and transfer of antibiotic resistance genes from enterococci isolated from food. Syst Appl Mic. 30:1–7.
- Jahan M, Holley RA. 2016. Transfer of antibiotic resistance from Enterococcus faecium of fermented meat origin to Listeria monocytogenes and Listeria innocua. Let App Mic. 62:304–310.
- Jaimee G, Halami PM. 2016. Emerging resistance to aminoglycosides in lactic acid bacteria of food origin – an impending menace. App Mic Bio. 100:1137–1151.
- Jiang S, Cai L, Lv L, Li L. 2021. Pediococcus pentosaceus, a future additive or probiotic candidate. Mic Cell Fact. 20:45.
- Klare I, Konstabel C, Muller-Bertlig S, Reissbrodt R, Huys G, Vancanneyt M. 2005. Evaluation of new broth media for microdilution antibiotic susceptibility testing of Lactobacilli, Pediococci. Lactococci Bifidobacteria Appl Env Mic. 71:8982–8986.
- Klose V, Bayer K, Kern C, Goelß F, Fibi S, Wegl G. 2014. Antibiotic resistances of intestinal lactobacilli isolated from wild boars. Vet Mic. 168:240–244.
- Li S, Li Z. 2015. Association of mutation patterns in gyra and parc genes with quinolone resistance levels in lactic acid bacteria. Antibiotics. 68:81–87.
- Markowiak P, Slizewska K. 2018. The role of probiotics, prebiotics and synbiotics in animal nutrition. Gut Pathog. 10:21.
- Mayrhofer S, Zitz U, Birru FH, Gollan D, Gołoś AK, Kneifel W. 2014. Comparison of the CLSI guideline and ISO/IDF standard for antimicrobial susceptibility testing of lactobacilli. Microb Drug Resist. 20:591–603.
- Nawaz M, Wang J, Zhou A, Ma C, Wu X, Moore JE. 2011. Characterization and transfer of antibiotic resistance in lactic acid bacteria from fermented food products. J Curr Mic. 62:1081–1089.
- Ouoba LII, Lei V, Jensen LB. 2008. Resistance of potential probiotic lactic acid bacteria and bifidobacteria of African and European origin to antimicrobials: determination and transferability of the resistance genes to other bacteria. Int J Food Mic. 121:217–224.
- Pang H, Qin G. 2011. Natural populations of lactic acid bacteria associated with silage fermentation as determined by phenotype, 16S ribosomal RNA and recA gene analysis. Syst App Mic. 34:235–241.
- Pang H, Tan Z, Qin G. 2012. Phenotypic and phylogenetic analysis of lactic acid bacteriaisolated from forage crops and grasses in the Tibetan Plateau. J Mic. 50:63–71.
- Pararajasingam A, Uwagwu J. 2017. Lactobacillus: the not so friendly bacteria. BMJ Case Rep. bcr-2016-218423:218423.
- Plumed-Ferrer C, Barberio A, Franklin-Guild R, Werner B, McDonough P, Bennett J. 2015. Antimicrobial susceptibilities and random amplified polymorphic DNA-PCR fingerprint characterization of Lactococcus lactis ssp. lactis and Lactococcus garvieae isolated from bovine intramammary infections. J Dairy Sci. 98:6216–6225.
- Sukmarini L, Mustopa AZ, Normawati M, Muzdalifah I. 2014. Identification of antibiotic-resistance genes from lactic acid bacteria in Indonesian fermented foods. HAYATI J Biosci. 21:144–150.
- Toomey N, Bolton D, Fanning S. 2010. Characterization and transferability of antibiotic resistance genes from lactic acid bacteria isolated from Irish pork and beef abattoirs. Res Mic. 161:27–35.
- Torriani S, Felis GE, Dellaglio F. 2001. Differentiation of Lactobacillus plantarum, L. pentosus, and L. paraplantarum by recA gene sequence analysis and multiplex PCR assay with recA gene-derived primers. Appl Environ Mic. 67:3450–3454.
- Vakulenko SB, Donabedian SM, Voskresenskiy AM, Zervos MJ, Lerner SA, Chow JW. 2003. Multiplex PCR for detection of aminoglycoside resistance genes in enterococci. Antimicrob Agents Chemother. 47:1423–1426.
- von Wintersdorff CJ, Penders J, van Niekerk JM, Mills ND, Majumder S, van Alphen LB. 2016. Dissemination of antimicrobial resistance in microbial ecosystems through horizontal gene transfer. Front Microbiol. 7:173.
- Ward LJ, Timminis MJ. 1999. Differentiation of Lactobacillus casei, Lactobacillus paracasei and Lactobacillus rhamnosus by polymerase chain reaction. Lett Appl Mic. 29:90–92.
- Zheng J, Wittouck S, Salvetti E, Franz CM, Harris HM, Mattarelli P. 2020. A taxonomic note on the genus Lactobacillus: description of 23 novel genera, emended description of the genus Lactobacillus Beijerinck 1901, and union of Lactobacillaceae and Leuconostocaceae. Int J Syst Evol Mic. 70:2782–2858.
- Zielińska K, Fabiszewska A, Stefańska I. 2015. Different aspects of Lactobacillus inoculants on the improvement of quality and safety of alfalfa silage. Chi J Agr Res. 75:298–306.
- Zielinska K, Fabiszewska A, Swiatek M. 2017. Evaluation of the ability to metabolize 1,2-propanediol by heterofermentative bacteria of the genus Lactobacillus. Elec J Biotech. 26:60–83.