ABSTRACT
Subclinical mastitis is an important problem in terms of the dairy economy all over the world and due to the increasing antibiotic resistance day by day, there is a great need for new antibiotic alternatives and natural products. The aim of this study is to examine the effectiveness of bee venom against bacterial mastitis agents. Methods, such as well diffusion, disc diffusion, microdilution and time-kill analyses, were applied to 12 bacteria species isolated from milk samples. Effective results were obtained in microdilution and time-kill analysis. MIC values were determined in the range of 12.5–50 µg/ml and time-kill times were observed in the range of 1–24 h. In addition, bacterial suspensions from 12 species were prepared and there were effective against mixed infection in vitro. As a result, bee venom, as an antibiotic alternative, can give satisfactory results in the treatment of subclinical mastitis and mixed infections. Field studies and treatment trials should be emphasized.
Introduction
Mastitis, which is one of the costliest problems for the dairy industry, is defined as inflammation of the mammary gland (Kurt and Eşki Citation2021). The disease can be seen in clinical, subclinical and chronic types which may vary depending on the udder, the causative organisms, immunity, age and lactation stage of the animal (Romero et al. Citation2018; Krishnamoorthy et al. Citation2021). In clinical mastitis, heat, swelling and pain in the affected udder and changes in the appearance of milk are clearly observed (Krishnamoorthy et al. Citation2021; Kurt and Eşki Citation2021). Subclinical mastitis does not produce visible changes on the udder and in the milk, and because it progresses without showing clinical symptoms, it is overlooked, can spread easily and continues for a long time. Economically, 70–80% of milk yield losses are due to subclinical mastitis (Demir and Eşki Citation2019). Chronic mastitis is a rare form of the disease. However, it causes consistent inflammation of the mammary gland in dairy cows (Krishnamoorthy et al. Citation2021). Chronic mastitis is a rare form of the disease. However, it causes consistent inflammation of the mammary gland in dairy cows. In clinical mastitis, heat, swelling and pain in the affected udder and changes in the appearance of milk are clearly observed (Krishnamoorthy et al. Citation2021; Kurt and Eşki Citation2021). Subclinical mastitis does not produce visible changes on udder and change in the milk. Since subclinical mastitis progresses without showing clinical symptoms, it is overlooked, can spread easily and continues for a long time. Economically, 70–80% of milk yield losses are due to subclinical mastitis (Demir and Eşki Citation2019). Mastitis has various aetiologies and is most commonly caused by bacteria and results in serious economic losses such as a decrease in milk production and quality, cost of antibiotic treatment or extra labour, milk disposal, decrease in the sale price of milk and the culling of animals (Kurt and Eşki Citation2021; Dalanezi et al. Citation2020). There are also losses due to a situation that has a significant impact on animal welfare and public health (Libera et al. Citation2021).
Overuse or misuse of antibiotics in the treatment of mastitis results in a marked increase in bacterial strains resistant to these antibiotics. Therefore, antimicrobial-resistant microorganisms have been of great concern because of the extensive clinical use of antibiotics. The World Health Organization (WHO) has concerns that infections may no longer be treatable with antibiotics available soon, due to the continued significant increase in antibiotic resistance in the world. WHO (13 January 2020) reported that these infections could pose a great threat to human life unless new antibiotics are developed against drug-resistant infections (Yacoub et al. Citation2020). The increase in drug-resistant bacterial strains indicates that new antibiotics or novel natural antimicrobial agents that can be used in treatment should be developed (Neubauer et al. Citation2017; Xie et al. Citation2017). For this reason, the development of new compounds as an alternative treatment to mastitis is needed. Bees are of great economic and medicinal importance, especially as they play a critical role in the pollination of many fruits, vegetables and seed crops. Bees also produce compounds such as honey, resin, beeswax, propolis, royal jelly, pollen and venom (apitoxin) (Leandro et al. Citation2015). Among these compounds, bee venom produced by bees as a defensive mechanism is one of the most important bioproducts and has recently attracted the attention of researchers. Honeybee venom is viscous, transparent and colourless in the liquid state and is a white or yellowish-white powder in the dry form. The composition of bee venom can vary depending on the honey bee species, strain, the place of origin and venom collection season (Kokot et al. Citation2009). The composition of bee venom includes peptides (Melittin, apamin, secapin, procamine, protease inhibitor, tertiapine, mast cell degranulation peptide and other small peptides), enzymes (phospholipase A2, phospholipase B, hyaluronidase, phosphatase, Α-glucosidase, acid phosphomonoesterase and lysophospholipase), sugars (Glucose and fructose), phospholipids and volatile compounds. It also contains amines (histamine, dopamine and noradrenaline) and amino acids (aminobutyric acid and α-amino acids) (Park et al. Citation2010; Pascoal et al. Citation2019). Bee venom is traditionally used in the treatment of many diseases such as arthritis, rheumatism, skin rash, itching, pain and inflammation (Curicio-Vonlanthen et al. Citation1997; Yin et al. Citation2007; Ratcliffe et al. Citation2014). It is also known to have anti-carcino-genetic activity against prostate, liver and breast cancer. In many recent studies, it has been reported that bee venom exhibits numerous biological activities, including anti-fungal, anti-viral, metabolic, inflammatory, anti-articular and anticancer effects (Son et al. Citation2007; Zolfagharian et al. Citation2016; Memariani et al. Citation2019). Bee venom also has effects on the immune systems, central and peripheral nervous systems and cardiovascular systems (Fennell et al. Citation1968; Carpena et al. Citation2020; Tanuğur-Samancı and Kekeçoğlu Citation2021). Some investigations have shown the antibacterial effect of bee venom against several pathogenic bacteria (Harwig et al. Citation1995; Koduri et al. Citation2002; Boutrin et al. Citation2008; Park et al. Citation2013).
The aim of this study is to examine the antibacterial activity of bee venom on various bacteria isolated from cattle with subclinical mastitis and mixed infections that are difficult to treat.
Materials and methods
Ethical statement
The present study was approved on 04/04/2022 by the Ethics Committee of Adana Veterinary Control Institute, Adana, Turkey (Approval no: 04/04/2022-1/227).
Chemicals
Standards, Apamin (Sigma-A1289), Phospholipase A2 (Sigma-P9279) and Melittin (Sigma M2272) were purchased from Sigma-Aldrich (St. Louis, MO, USA) to determine the bee venom (BV) content.
Sample collection and preparation
Bee venom samples were collected in the citrus honey season between May and June 2021 from Apis mellifera anatoliaca (Muğla ecotype) hives located in the Menteşe provinces of Turkey. A bee venom collector (BeeSas beekeeping, Turkey) was used for the sample collection.
Bee venom is obtained by scraping with a sharp scalpel after being collected on glass plates and kept in air and shade for 8 h at room conditions. BV was then freeze-dried and placed in a −18°C freezer until analysis.
Determination of bee venom content
Apamin, phospholipase-A2 and melittin components in bee venom were analyzed by high-performance liquid chromatography (HPLC) – a variable wavelength detector (VWD) (Agilent 1260 series). The Agilent 1260 series HPLC configuration consists of a quad gradient pump (G1311B), a column oven (G1316A), an autosampler (G1329B) and VWD (G1314F).
It was segregated by an InfinityLab Poroshell C18 EC-C18 (4.6 × 50 mm, 2.7 microns) column. The optimum separation temperature was 20°C and the column flow rate was 1 ml/min. Standard mixture solutions, contained apamin, phospholipase A2 and melittin were prepared at concentrations of 25, 50,100 and 125 μl/ml. The gradient programme is optimized for peak retention times. Absorbance was measured at 220 nm.
Animal and management
This study was carried out on 80 multiparous Holstein dairy cows at a medium-scale commercial farm in Adana, Turkey. The animals were aged (3 and 4) with a milk yield (of 35 and 40 l/day) and lactation times (2 and 3). They were managed in free stall barns under the same conditions, milked twice a day using an automatic milking system, fed with a total mix ratio and had free access to water. Pre- and post-milking udder disinfection procedures and mastitis screenings were routinely performed on the farm.
California mastitis test
California mastitis test (CMT) was performed for the confirmation of the examination applied by inspection and palpation. The test process and the interpretation of CMT results were made according to a previous study (Baştan et al. Citation2008). No treatment was performed before and during the study. Subclinical mastitis was formed according to CMT and udder examination. The CMT was performed according to the manufacturer’s instructions (California mastitis test kit, ImmuCell). The CMT reaction of each quarter was recorded as negative (0), trace, 1+, 2+ and 3+. If one of the 1+, 2+ and 3+ values was obtained, it was considered positive. All tests were performed for four teats of each cow. A total of 320 teats were examined.
Milk sample collection
Milk samples were taken before morning milking and feeding. Before collecting the milk, the udder and the teat ends were washed, cleaned and dried, and samples were taken after the foremilk was discarded. Milk samples were collected from individual quarters with subclinical mastitis (n = 20) to sterile falcon tubes (Isolab®, Germany) under asepsis and antisepsis conditions. After the milk samples were collected, they were immediately transported to the laboratory under the cold chain (+4°C) for microbiological analyses.
Isolation and identification of subclinical bacterial mastitis agents
The bacteria were isolated from milk samples and transported to the laboratory under the cold chain (+4°C). Samples (5 ml) from each sample were poured directly into the sterile stomacher bags with a filter (Fisher Scientific, Nepean, ON, Canada) aseptically and 45 ml of sterile ringer solution was added and homogenized. The decimal dilution was pipetted and spread out the surface of Blood agar, Endo Agar and Saboraud Dextrose Agar (SDA) plates (Sigma-Aldrich, St. Louis, MO, USA) before incubation at 37°C for 24–48 h. The isolates were first evaluated by Gram staining to examine the morphology of colonies and biochemical test characteristics. Then, the bacterial species were identified by using the VITEK-2 automated identification system (Biomerieux, Basingstoke, UK).
Determination of antibiotic susceptibility for subclinical mastitis agents
Antibiotic susceptibility was evaluated by the Kirby-Bauer disk diffusion method (Bauer et al. Citation1966). Amoxicillin (30 µg), Amikasin (30 µg), Trimethoprim-sulfamethoxazole (1.25/23.75 µg), Neomycin-Bacitracin-Tetracycline (30 µg/10IU/30 µg), Gamithromycin (15 µg), Rifaximin (40 µg), Framycin-Penicillin G (100 µg/10IU), Cephalexin-kanamycin (15 µg/30 µg), Cefapirin (30 µg) and Enrofloxacin (5 µg) (Mast Diagnostics, Merseyside, UK) discs were used. Results were interpreted according to the guidelines of the Clinical and Laboratory Standards Institute (CLSI).
Antibacterial effect of bee venom on subclinical mastitis agent
Following antibiotic susceptibility tests, disc diffusion, well diffusion and microdilution tests were applied to the isolates. In addition, due to time and antibiotic concentration, the time-killing method, which is a method of dynamically revealing the bactericidal effect of the antibiotic, was applied. In addition, due to mixed infections, bee venom antimicrobial activity was tested on a mixture of isolated bacteria. Mc Farland 0.5 turbidity value of all isolated species was taken after the suspension was made. The concentration of this bacterial mixture was set to 2 × 106 cfu/ml, and the bee venom activity in this mixture was examined in all tests.
Disc diffusion method
In this method, firstly, bacterial suspensions, adjusted to 0.5 Mcfarland turbidity, were spread on the Muller Hinton Agar plates (MHA). Then 6.25, 12.5, 25, 50, 100, 200, 400 and 800 μg of bee venom were adsorbed onto blank discs and placed on the agar. Plates were incubated at 37°C for 24 h and the inhibition zones formed around the disc were measured with a calliper.
Agar well diffusion method
Antibacterial effects of bee venom were determined using the agar well diffusion method according to a previous study with minor modifications against tested bacteria (Yazgan et al. Citation2021). Briefly, after standardizing the overnight bacteria isolates to 0.5 McFarland turbidity (1.5 × 108 cfu/ml), 0.1 ml of each bacterial suspension was spread on sterile Muller Hinton Agar (MHA) plates. Agar wells of 5 mm in diameter were made in agar plates using a sterile plastic cylinder. Each well was filled with 100 µl of 6.25, 12.5, 25, 50, 100, 200, 400 and 800 μg/ml concentrations of bee venom. MHB and distilled water were also used as control. Then, all plates were incubated at 37°C for 24 h. After incubation, the inhibition zones formed around the well were measured with a calliper. The results were expressed in mm by the arithmetic mean of the diameter of the inhibition zone around each well. All tests were performed in triplicate for each sample.
Microdilution method
Minimal inhibition concentration (MIC) and minimal bactericidal concentration (MBC) of bee venom were determined by the broth dilution method (CLSI Citation2015). The stock solution was prepared by dissolving in 1 ml distilled water as 6.25, 12.5, 25, 50, 100, 200, 400 and 800 μg. Serial dilution from each stock concentration was performed on microplates (96-well microplates) by the microdilution method. Twenty microlitres of bee venom at each concentration was added to each well ELISA microplate. Then, 200 μl of each bacterial culture (2 × 106 cfu/ml) was inoculated on each well microplate containing bee venom and Muller Hinton Broth (MHB). The well including MHB and the bacterial suspension was used as the positive control. The well without MHB was also used as the negative control. The microplates were incubated overnight at 37°C and were read absorbance at 560 and 620 nm. MIC value was determined for each bacterium according to absorbance values on microplates. Then, the MBC values were determined by inoculating the samples in the microplates on the Müller Hinton Agar. All tests were performed in triplicate.
Time-kill assay
The time-kill assay of bee venom, against all tested bacteria, was evaluated at their MIC according to the previous method with minor modifications (Chuesiang et al. Citation2019). Each grew without formulation. Different time intervals (0, 1, 3, 6, 12 and 24 h) were applied in the assay. Briefly, 1 ml of overnight growth of each bacterial suspension at 0.5 McFarland turbidity (1.5 × 108 cfu/ml) was inoculated to each tube containing bee venom at MIC concentration. The tube was incubated for 0, 1, 3, 6, 12 and 24 h at 37°C. 0.1 ml of each bacterial suspension was spread on the MHA agar plate at each time point and incubated at 37°C for 24 h. After incubation bacterial colonies were counted for the time-kill assay curve.
Results
Chemical compositions of bee venom
The major compounds detected in the bee venom were mellitin and phospholipase A with 75.39%, and 13.82%, respectively, whereas other compounds in a low concentration of 4.97% apamine (). The chromatogram for the chemical composition of bee venom is displayed in .
Figure 1. HPLC-VWD chromatogram and calibration curves of Apamin, Phospholipase-A2 and Melittin components in bee venom.
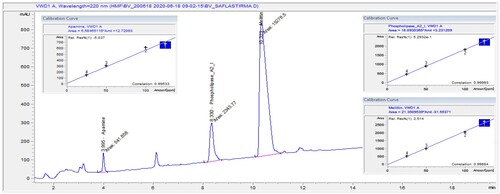
Table 1. The concentrations of components of bee venom (± SD standard deviation).
Identification of subclinical bacterial mastitis agent
Bacteria isolated from milk samples were analysed with the VITEK-2 identification system and various biochemical tests, and 12 species of bacteria were identified. These isolates are Staphylococcus aureus, Staphylococcus epidermidis, Staphylococcus xylosus, Streptococcus uberis, Streptococcus dysagalactiae, Enterococcus casei, Kocuria varians, Aerococcus viridans, Pseudomonas oryzohabitans, Sphingomonas paucimobilis, Serratia marcescens and Acinetobacter lwoffi. While mixed infections and more than one agent were seen in some cattle, a single agent was isolated in others.
Determination of antibiotic susceptibility for subclinical mastitis agents
To determine the antibiotic susceptibility profiles, the sensitivity of the isolated mastitis agents to the antibiotics used in the treatment of mastitis was investigated. Twelve isolates were found to be sensitive to Amoxicillin, Amikasin, Trimethoprim-Sulfamethoxazole, Neomycin-Bacitracin-Tetracycline, Gamithromycin, Rifaximin, Framycin-Penicillin G, Cephalexin-Kanamycin, Cefapirin and Enrofloxacin. Amoxicillin was not evaluated for Serratia marcescens because of its intrinsic resistance.
Antibacterial effect of bee venom on subclinical mastitis agents
Disk diffusion method
In the disk diffusion test, no inhibition zones were observed in any isolate. The diameter of the inhibition zone widened as the concentration increased.
Agar well diffusion method
Subsequently, the well diffusion test was also performed on all isolates at the same concentrations as the microdilution test. The zone diameters were measured for each concentration and ranged from 0.5 to 21 mm, sometimes no zone diameter was detected (ND).
Determination of MIC and MBC values
Firstly, MIC and MBC values were determined by the microdilution method to examine the interactions of mastitis agents with bee venom. The microdilution method was applied to the microplates using 6.25, 12.5, 25, 50, 100, 200, 400, 800 and 1600 µg/ml concentrations of bee venom. Absorbances at 560 and 620 nm wavelengths were measured with an ELISA reader. The MIC values of the agents were between 12.5 and 50 µg/ml, and the MBC values were between 12.5 and 50 µg/ml.
Time-kill assay
In the time-kill analysis, it was determined at what concentration and for how long mastitis agents were inhibited. The effects of 12.5, 25, 50, 100, 1000 and 2500 µg/ml concentrations of bee venom were examined at 0, 1, 3, 6, 12 and 24 h, respectively. The concentration and time that inhibited the growth of bacteria on agar were evaluated as effective. The time-kill times varied between 1 and 24 h, and the concentration varied between 25 and 2500 µg/ml depending on the isolate. In all concentrations, a decrease in 4–6 logs was detected in the growth curve of the isolates (, ).
Table 2. Microdilution, time-killing and well diffusion tests through which bee venom is tested at various concentrations.
For gram-positive bacteria such as S. aureus, S. epidermidis, S. xylosus, S. uberis and K. varians, the shortest inhibition timepoint was 1 h at 2.5 mg/ml of bee poison concentration. The shortest inhibition timepoint for E. casei is 3 h at 2.5 mg/ml. In non-fermentative gram-negative isolates such as P. oryzihabitans, S. paucimobilis and S. marcescens, inhibition timepoint was 24 h for almost all concentrations. In 12 h, a significant decrease was detected in the growth curve of S. paucimobilis isolate. The shortest inhibition timepoint was 3 h at 2.5 mg/ml for A. lwoffii and A. viridans. When time-kill is analyzed according to MIC values, inhibition was observed for S. aureus, S. epidermidis and K.varians at a concentration of 12.5 µg/ml in 3 h, for S. uberis at a concentration of 25 µg/ml in 3 h, for S. dysagalactiae, P. oryzihabitans, S. paucimobilis and S. marcescens at a concentration of 12.5 µg/ml 24 h, for S. xylosus and A. viridans at a concentration of 12.5 µg/ml in 6 h, for E. hirae at a concentration of 12.5 µg/ml in 12 h and for A. lwoffii at a concentration of 50ug/ml in 24 h. The bacterial mixture was inhibited in 6 h at both MIC and all bee venom concentrations.
Discussion
Subclinical mastitis is a type of mammary infection that can be recognized by the isolation of pathogenic agents and determination of some biochemical changes in milk, which do not show local and systemic symptoms and cause an increase in the amount of leucocytes in milk. In this study, animals that do not show local and systemic symptoms with a decreased milk yield and a positive CMT test, were evaluated as subclinical mastitis. Although the use of antibiotics is the main treatment strategy in subclinical mastitis, natural alternatives to antibiotic treatment from plant and animal products are sought due to the increasing number of antibiotic-resistant pathogens, the high incidence of mixed infections and the impact on the dairy industry in terms of drug residues (Cheng and Han Citation2020).
Toxins and venoms of animal origin are potential antimicrobials with no previous clinical application. They have evolved as part of the defence strategies of animals. Raw venoms contain different peptide toxins that are generally small, easy to synthesize, structurally stable and have high biodiversity (Lewis and Garcia Citation2003).
Apis mellifera venom is one of the best toxins . The antimicrobial activity of bee venom is provided particularly by melittin and phospholipase A (PLA), as well as adolapin, apamin, mast cell degranulating peptides, enzymes, biologically active amines and various peptides. Therefore, the determination of the qualitative and quantitative content of bee venom is significant as the effect of its antimicrobial activity. The content of melittin, which causes pore formation in the membrane, varies between 50% and 60%, and the total protein content varies between 47.2% and 77.8% (Samancı and Kekeçoğlu Citation2019; Sonmez et al. Citation2022). In our study, the melittin value of the bee venom was 70.49%. On the other hand, the bactericidal effect of PLA is the destruction of phospholipids in the membrane. While the PLA ratio in bee venom is approximately 10–12%, this ratio is 9.27–20.95% in Apis mellifera anatoliaca venom (Tanuğur-Samancı and Kekeçoğlu Citation2021; Bogdanov Citation2016). The PLA ratio of the bee venom we obtained was 13.51%. The melittin and PLA content of the bee venom we obtained was of very high quality.
We isolated 12 species of bacteria from 20 animals with subclinical mastitis, and primarily a disc diffusion test was performed with antibiotics commonly used in the treatment of mastitis. All isolates were susceptible to all antibiotics. Subsequently, we used bee venom to find a natural antibacterial alternative to these antibiotics that could develop resistance and inhibit mixed infections, which is an important problem of subclinical mastitis, with a single preparation. Disc diffusion, well diffusion, microdilution and time-kill tests were applied to examine the antibacterial activity of bee venom.
Although zones of inhibition have been reported in various studies, no zone of inhibition was observed for any bacteria in the disk diffusion test in our study (Zolfagharian et al. Citation2016; Fadl Citation2018; Nassar et al. Citation2018). The absence of an inhibition zone made us think that although its molecular weight is 2840 Da, a sufficient amount of active substance could not diffuse from the disc and it may not be a good alternative in bee venom susceptibility studies (Kolayli and Keskin Citation2020).
In our study, inhibition zones were determined for S. aureus, S. uberis, S. epidermidis, S. xylosus, P. oryzihabitans and S. paucimobilis at all concentrations in the well diffusion tests. For K. varians, A. lwoffi, S. marcescens and E. casei, zone diameters could not be determined at some concentrations. No zones were obtained in S. dysagalactiae, A. viridans and bacterial mixture. In two well diffusion studies for S. aureus, an inhibition zone of 2.5 mg/ml wasp venom 24 mm and 12.5 µg/ml honeybee venom 11 mm was detected (Al-Shammery and Hozzein Citation2022; Sonmez et al. Citation2022). In our study, a 17 mm zone was detected for S. aureus at a concentration of 12.5 µg/ml. The reason for the large diameter of our zone may be the high melittin ratio. Well diffusion studies on other bacteria were not found in the literature.
In both the well diffusion test and the disc diffusion test, clear and consistent results could not be obtained as in the microdilution test. In these tests, active substances may not show the same diffusion rate in the agar due to polarity, solubility, size, clogging and the like (Didaras et al. Citation2020).
By the microdilution test, MIC and MBC values of all bacteria were determined. While our study was planned, concentrations of 6.25–800 μg/ml were tested on mastitis isolates following all these studies. Bee venom MIC values of mastitis isolates were between 12.5 and 50 μg/ml. The sensitivity of all tested pathogens to bee venom was achieved at 12.5–25 µg/ml and 12.5–50 µg/ml for gram-positive and gram-negative bacteria, respectively. In various studies, this value is shown as 10–100 μg/ml for gram-positive bacteria, 30–500 μg/ml for gram-negative bacteria, 20–40 µg/ml for oral pathogens and 256–1024 µg/ml for Salmonella species isolated from poultry (Al-Ani et al. Citation2015; Leandro et al. Citation2015; Arteaga et al. Citation2019).
S. paucimobilis has the highest MIC value (25 μg/ml) for bee venom. Sfingomonas spp. has glycosphingolipids (GSL) instead of lipopolysaccharide (LPS) and may develop in various environments that cannot be tolerated by most other bacteria. Even after disinfection processes in milk production facilities, its presence has been determined. This resistant state also causes it to appear as the causative agent of hospital infection (Kuehn et al. Citation2013). These properties of S. paucimobilis may be the reason for the high MIC value of bee venom.
S. uberis has the second MIC value (25 μg/ml) for bee venom. S. uberis is a source of intimidation in mastitis treatments. It causes recurrent infections and cannot be controlled by traditional mastitis control programmes. It easily adapts to the environment and forms a biofilm. These properties may have affected MIC values.
Mixed infections are one of the biggest problems in mastitis treatments. One or two pathogen species cause mixed infections. However, we mixed 12 types of pathogens to know the effect and potential of bee venom more clearly although it is generally found in the literature that 2–3 agents cause mixed infections. These 12 species are pathogens that we frequently encounter in routine laboratory practices of our faculty. The MIC value of the bacteria mixture is 25 μg/ml.
Previous studies have suggested that gram-positive bacteria are more sensitive to melittin than gram-negative bacteria. Interestingly, no significant difference was observed in the MIC values of gram-positive and gram-negative bacteria in our study. It is known that the mechanical action of bee venom is a pore in the cell membrane in many studies. Previous studies on gram-positive bacteria suggest that the effect of BV on cell membrane pore formation and permeability is associated with melittin. They observed the effect of melittin on S.aureus as damage in the cell membrane, pore formation and cell permeability (Marques Pereira et al. Citation2020). However, the external membrane of gram-negative bacteria containing the LPS layer prevents melittin penetration from the cytoplasmic membrane (Shai Citation2002; Al-Ani et al. Citation2015). As we know, phospholipase A2, the second main compound of bee venom, indirectly disrupts the cell membrane of gram-negative bacteria at low speed for long periods (Haktanir et al. Citation2021). In summary, melittin has antibacterial effects on gram-positives, while phospholipase A2 affects gram-negatives. Thus, bee venom shows antimicrobial activity regardless of gram-positive and -negative bacteria in mastitis pathogens.
In the time-kill test, all isolates were prepared as MIC values and upper concentrations. In addition, concentrations of 1 and 2.5 mg/ml were added. S. xylosus was inhibited for 1 h at 25 μg/ml. This is the lowest concentration and shortest-term inhibition of the time-kill assay. All gram-negative bacterial species in our study were inhibited in 24 h at all concentrations (except A. lwoffi, in which 2.5 mg/ml was inhibited in 3 h). The reason for this long time (24 h) may be that phospholipase A2 affects the LPS layer and the GSL layer at a low speed for a long time (Haktanir et al. Citation2021).
In our antibacterial activity tests, various concentrations of bee venom were used at the micrograms of level. In addition, 1 mg/ml and 2,5 mg/ml concentrations were added to contribute to clinical studies in the future. Because the pharmacokinetic activity of bee venom is unknown. The concentration level to be used in treatment may be well above the MIC value. Therefore, studies should be carried out to evaluate bee venom in terms of pharmacokinetics and pharmacodynamics. Furthermore, in addition to pharymacokinetic and pharmacodynamic studies, the age of the animal, the weight of the animal and the dry or lactation period should be taken into consideration. However, the clinical stage is still planned. Our MIC values are lower than those in many antibacterial activity studies. This aroused the idea that bee venom could provide high efficiency in low doses. Of course, only clinical practices will show this.
There are fewer studies on the antibacterial effect of bee venom against mastitis agents (Han et al. Citation2007; Park et al. Citation2010; Fadl Citation2018). Bee venom has been applied subcutaneously in various treatment studies (Bava et al. Citation2023). In mastitis treatment studies, subcutaneous applications were made to the area of the supra-mammary lymph nodes. However, the number of bacterial species remained very limited (Han et al. Citation2009). The most significant difference of our study from other studies is that it was tested on many bacterial agents, three methods were used to detect susceptibility to bee venom, and a time-kill was determined for each species. Additionally, it was determined that bee venom is effective against mixed infections, which is a crucial problem in mastitis and where antibiotics are insufficient. However, in our study planned for the future, the treatment will be in the form of application of the preparation into the breast (teat canal). The reason why we prefer a local application in this way is that it is not an invasive procedure and the anaphylactic risk is low. In addition, bee venom pharmaceutical formulation must be suitable for local tolerance of intramammary preparations in cows given by some institutions and agents.
In conclusion, bee venom is a bioproduct with high potential and efficacy as an antibiotic alternative. The effectiveness of bee venom on mixed infections, which complicates the treatment of mastitis and is very common in the field, was demonstrated in our study. Although it is difficult to be preferred in systemic treatments due to its allergic efficacy, it can be considered to be applied as an ointment, pomade, spray or intramammary preparation in local treatments. To reflect these practices in the field, it is recommended to support them with animal experiments and to try them in treatment with ethical permissions.
Acknowledgements
The authors gratefully acknowledge Adana Veterinary Control Institute for technical assistance during study preparation and data collection. They thank the Scientific Research Project Department for the support.
Disclosure statement
No potential conflict of interest was reported by the author(s).
Additional information
Funding
References
- Al-Ani I, Zimmermann S, Reichling J, Wink M. 2015. Pharmacological synergism of bee venom and melittin with antibiotics and plant secondary metabolites against multi-drug resistant microbial pathogens. Phytomedicine. 22:245–255.
- Al-Shammery KA, Hozzein WN. 2022. Antibacterial activities of two potential peptides extracted from Polistes wattii Cameron 1900 (Vespidae: Polistinae) wasp venom collected at Eastern Province Saudi Arabia. PLoS ONE. 17(3):e0264035.
- Arteaga V, Lamas A, Regal P, Vázquez B, Manuel J, Cepeda A, Manuel C. 2019. Antimicrobial activity of apitoxin from Apis mellifera in Salmonella enterica strains isolated from poultry and its effects on motility biofilm formation and gene expression. Microb Pathog. 137:103771–103776.
- Baştan A, Kaçar C, Acar DB, Şahin M, Cengiz M. 2008. Investigation of the incidence and diagnosis of subclinical mastitis in early lactation period cows. Turk J Vet Anim Sci. 32:119–121.
- Bauer AW, Kirby WMM, Sherris JC, Turck M. 1966. Antibiotic susceptibility testing by a standardized single disk method. Am J Clin Pathol. 36:493–496.
- Bava R, Castagna F, Musella V, Lupia C, Palma E, Britti D. 2023. Therapeutic Use of Bee venom and potential applications in veterinary medicine. Vet Sci. 10(2):119.
- Bogdanov S. 2016. Bee venom: Production, composition, quality. In: The bee venom book, Chapter 1, Bee product science. Muehlethurnen. Retrieved from May 2017, http://www.bee-hexagon.net/venom/production-composition-quality/.
- Boutrin MC, Foster HA, Pentreath VW. 2008. The effects of bee (Apis mellifera) venom phospholipase A2 on Trypanosoma brucei brucei and Enterobacteria. Exp Parasitol. 119(2):246–251.
- Carpena M, Nuñez-Estevez B, Soria-Lopez A, Simal-Gandara J. 2020. Bee venom: an updating review of its bioactive molecules and its health applications. Nutrients. 12(11):3360.
- Cheng WN, Han SG. 2020. Bovine mastitis: risk factors therapeutic strategies and alternative treatments - a review, Asian-Australas. J Anim Sci. 33(11):1699–1713.
- Chuesiang P, Siripatrawan U, Sanguandeekul R, McClements DJ, McLandsborough L. 2019. Antimicrobial activity of PIT-fabricated cinnamon oil nanoemulsions: effect of surfactant concentration on morphology of foodborne pathogens. Food Control. 98:405–411.
- Clinical and Laboratory Standards Institute. 2015. Method for dilution antimicrobial susceptibility tests for bacteria that grow aerobically; approved standard—10th ed, CLSI document M07-A10. Wayne (PA): Clinical and Laboratory Standards Institute.
- Curicio-Vonlanthen V, Schneider CH, Frutig K, Blaser K, Kalabacher H. 1997. Molecular parameters in melittin immunogenicity. J Pept Sci. 3(4):267–276.
- Dalanezi FM, Joaquim SF, Guimarães FF, Guerra ST, Lopes BC, Schmidt EMS, Cerri RLA, Langoni H. 2020. Influence of pathogens causing clinical mastitis on reproductive variables of dairy cows. J Dairy Sci. 103:3648–3655.
- Demir P, Eşki F. 2019. Estimate by quantitative methods of the effect on some milk yield traits with CMT score of subclinic mastitis in cows: pilot study. Van Vet J. 30:177–182.
- Didaras NA, Karatasou K, Dimitriou TG, Amoutzias GD, Mossialos D. 2020. Antimicrobial activity of bee-collected pollen and beebread: state of the art and future perspectives. Antibiotics. 9(11):811.
- Fadl A. 2018. Antibacterial and antibiofilm effects of bee venom from (Apis Mellifera) on multidrug-resistant bacteria (MDRB). Al-Azhar J Pharm Sci. 58(2):60–80.
- Fennell JF, Shipman WH, Cole LJ. 1968. Antibacterial action of melittin a polypeptide from bee venom. Proc Soc Exp Biol Med. 127(3):707–710.
- Haktanir I, Masoura M, Mantzouridou FT, Gkatzionis K. 2021. Mechanism of antimicrobial activity of honeybee (Apis mellifera) venom on Gram-negative bacteria: Escherichia coli and Pseudomonas spp. AMB Express. 11:54.
- Han SM, Lee K, Yeo J, Hwang S, Chenoweth PJ, Pak SC. 2009. Somatic cell count in milk of bee venom treated dairy cows with mastitis. J ApiProduct ApiMed Sci. 1:104–109.
- Han SM, Lee K, Yeo J, Kweon H, Kim B, Kim J, Baek H, Kim S. 2007. Antibacterial activity of the honey Bee venom against bacterial mastitis pathogens infecting dairy cows. Int J Ind Entomol. 14:137–142.
- Harwig SS, Tan L, Qu XD, Cho YOON, Eisenhauer PB, Lehrer RI. 1995. Bactericidal properties of murine intestinal phospholipase A2. J Clin Invest. 95(2):603–610.
- Koduri RS, Grönroos JO, Laine VJ, Le Calvez C, Lambeau G, Nevalainen TJ, Gelb MH. 2002. Bactericidal properties of human and murine groups I,II,V,X and XII secreted phospholipases A2. J Biol Chem. 277(8):5849–5857.
- Kokot ZJ, Matysiak J, Kłs J, Kędzia B, Hołderna-Kędzia E. 2009. Application of principal component analysis for evaluation of chemical and antimicrobial properties of honey bee (Apis mellifera) venom. J Apic Res. 48(3):168–175.
- Kolayli S, Keskin M. 2020. Natural bee products and their apitherapeutic applications. Stud Nat Prod Chem. 66:175–196.
- Krishnamoorthy P, Goudar AL, Suresh KP, Roy P. 2021. Global and countrywide prevalence of subclinical and clinical mastitis in dairy cattle and buffaloes by systematic review and meta-analysis. Res Vet Sci. 136:561–586.
- Kuehn JS, Gorden PJ, Munro D, Rong R, Dong Q, Plummer PJ, Wang C, Phillips GJ. 2013. Bacterial community profiling of milk samples as a means to understand culture-negative bovine clinical mastitis. PLoS One. 8(4):e61959.
- Kurt S, Eşki F. 2021. Pathogen isolation and antibiogram analysis in dairy cows with clinical mastitis in Adana region Turkey. Etlik Vet Mikrobiyol Derg. 32(1):20–26.
- Leandro LF, Mendes CA, Casemiro LA, Vinholis AH, Cunha WR, Almeida RD, Martins CH. 2015. Antimicrobial activity of apitoxin melittin and phospholipase A2 of honey bee (Apis mellifera) venom against oral pathogens. An Acad Bras Ciênc. 87:147–155.
- Lewis RJ, Garcia ML. 2003. Therapeutic potential of venom peptides. Nat Rev Drug Discov. 2(10):790–802.
- Libera K, Konieczny K, Witkowska K, Żurek K, Szumacher-Strabel M, Cieslak A, Smulski S. 2330. The association between selected dietary minerals and mastitis in dairy cows—a review. Animals. 1(8):2021.
- Marques Pereira AF, Albano M, Bérgamo Alves FC, Murbach Teles Andrade BF, Furlanetto A, Mores Rall VL, Delazari Dos Santos L, de Oliveira Orsi R, Fernandes Júnior A. 2020. Influence of apitoxin and melittin from Apis mellifera bee on Staphylococcus aureus strains. Microb Pathog.141:104011.
- Memariani H, Memariani M, Shahidi-Dadras M, Nasiri S, Akhavan MM, Moravvej H. 2019. Melittin: from honeybees to superbugs. Appl Microbiol Biotechnol. 103(8):3265–3276.
- Nassar M, Mawgoud Y, Dawoud M, Helal S, Fathy HM. 2018. Effect of honey bee venom against intensive care units environmental pathogens, Egypt. J Exp Biol. 14(2):237–244.
- Neubauer D, Jaśkiewicz M, Migoń D, Bauer M, Sikora K, Sikorska E, Kamysz W. 2017. Retro analog concept: comparative study on physico-chemical and biological properties of selected antimicrobial peptides. Amino Acids. 49(10):1755–1771.
- Park JH, Kim KH, Kim SJ, Lee WR, Lee KG, Park KK. 2010. Bee venom protects hepatocytes from tumor necrosis factor-α and actinomycin D. Arch Pharmacal Res. 33(2):215–223.
- Park S, Park BD, Yun S, Kang HJ, So B. 2013. Antimicrobial activities of honey bee venom against pathogens isolated from clinical bovine mastitis in Korea. Planta Med. 79(13):PL16.
- Pascoal A, Estevinho MM, Choupina A, Sousa-Pimenta M, Estevinho LM. 2019. An overview of the bioactive compounds therapeutic properties and toxic effects of apitoxin. Food Chem Toxicol. 134:1–11.
- Ratcliffe N, Azambuja P, Mello CB. 2014. Recent advances in developing insect natural products as potential modern day medicines. Evid Based Complement Alternat Med. 2014:904958.
- Romero J, Benavides E, Meza C. 2018. Assessing financial impacts of subclinical mastitis on Colombian dairy farms. Front Vet Sci. 5:273.
- Samancı T, Kekeçoğlu M. 2019. Comparison of commercial and anatolian bee venom in terms of chemical composition. Uludağ Arıcılık Derg. 19(1):61–68.
- Shai Y. 2002. Mode of action of membrane active antimicrobial peptides. Biopolymers. 66:236–248.
- Son DJ, Lee JW, Lee YH, Song HS, Lee CK, Hong JT. 2007. Therapeutic application of anti-arthritis pain-releasing and anti-cancer effects of bee venom and its constituent compounds. Pharmacol Ther. 115(2):246–270.
- Sonmez E, Kekecoglu M, Bozdeveci A, Karaoglu SA. 2022. Chemical profiling and antimicrobial effect of Anatolian honey bee venom. Toxicon. 213:1–6.
- Tanuğur-Samancı AE, Kekeçoğlu M. 2021. An evaluation of the chemical content and microbiological contamination of Anatolian bee venom. PloS One. 16(7):e0255161.
- Xie J, Zhao Q, Li S, Yan Z, Li J, Li Y, Wang R. 2017. Novel antimicrobial peptide CPF-C1 analogs with superior stabilities and activities against multidrug-resistant bacteria. Chem Biol Drug Des. 90(5):690–702.
- Yacoub T, Rima M, Karam M, Sabatier J M, Fajloun Z. 2020. Antimicrobials from venomous animals: An overview. Molecules. 25(10):2402.
- Yazgan H, Kuley E, Güven Gökmen T, Regenstein J M, Özogul F. 2021. The antimicrobial properties and biogenic amine production of lactic acid bacteria isolated from various fermented food products. J Food Process Preserv. 45(1):e15085.
- Yin C, Park HJ, Chae Y, Ha E, Park HK, Lee HS, Lee H. 2007. Korean acupuncture: the individualized and practical acupuncture. Neurol Res. 29(1):10–15.
- Zolfagharian H, Mohajeri M, Babaie M. 2016. Bee venom (Apis Mellifera) an effective potential alternative to gentamicin for specific bacteria strains: Bee venom an effective potential for bacteria. J Pharmacopuncture. 19(3):225–230.