Abstract
The study investigated the effects of FMD vaccination on acute phase response and milk composition in the HF crossbred cows (n = 12) using a cross-over experimental design. In trial 1, one group (n = 6) received inactivated trivalent oil-adjuvanted FMD vaccine [day post-vaccination, (dpv) 0], while the mock-vaccinated group received saline (n = 6). After a cooling period of 15 days, the trial was repeated by swapping groups. The body temperature, dry matter intake (DMI) and milk yield were recorded. Haemato-biochemical parameters, haptoglobin and cortisol concentrations were also assessed. A significant (p < 0.05) increase in the neutralizing antibody response to FMDV serotype O and Asia1 indicated a positive immune response. A significant (p < 0.05) increase of 0.4° to 0.6°C in the body temperature, decrease of 0.7–0.8 kg in DMI along with an increase in the serum haptoglobin, total leukocyte count and neutrophils within dpv 2 indicated acute phase response in the FMD vaccinated group. Among the milk constituents, only milk fat (p = 0.053) was significant. It was concluded that FMD vaccination induced acute phase response within dpv 3 in the crossbred cows and caused a non-significant reduction in the milk yield on the day of vaccination.
1. Introduction
Foot-and-mouth disease (FMD) is an economically important transboundary disease affecting the cloven-footed livestock and wild animals. Epidemiological investigations from the endemic countries demonstrate that FMD infection adversely affects the lifetime productivity of the cows. For instance, the FMD outbreak caused a reduction of about 7 kg milk yield/day/cow for about 8 weeks in Kenya (Lyons et al. Citation2015). In a Holstein herd in Iran, FMD outbreak resulted in a reduction of 4.7–8% in the mean daily milk production per cow during their first and second lactation, a decrease in the fat content of the milk and weight loss of 7.1 kg in the heifers (Ansari-Lari et al. Citation2017). A retrospective cohort study involving a dairy herd of 328 cows [purebred Jersey, Holstein-Friesian (HF) or crossbred cows] revealed that FMD outbreak increased the median age of first calving and decreased the odds of conception in Kenya (Chaters et al. Citation2018). In India, the estimated economic loss due to FMD is INR 120,000–140,000 million rupees (Singh et al. Citation2013). India launched biannual vaccination campaign to control FMD in 2003–2004 using inactivated oil-adjuvanted trivalent FMD vaccine (covering the serotypes O, A, and Asia1) and by 2018, entire India was brought under the umbrella through a flagship program of National animal disease control program that aims to control FMD by 2025 (Annual report 2017–18 Department of Animal Husbandry, Citation2018).
The handling of animals during vaccination and inflammatory response evoked in response to injection can itself be a stressful management procedure that may have short-term adverse effects on the production. It is reported that FMD vaccination in cows (Aftovax with O1, A22, and Asia1 serotypes adjuvanted in aluminium hydroxide gel and saponin) resulted in a drop in milk yield from day 8 post-vaccination (dpv) and loss persisted for seven days (Yeruham et al. Citation2001). Milk yield reduced ∼ 2 kg on dpv 1 with a significant increase in body temperature lasting for 48 h after the administration of a killed vaccine (Triangle 9 Ayerst Veterinary Laboratories, Ontario, Canada) in the high yield HF cows (Scott et al. Citation2001). Vaccination induced increased concentrations of TNFα for 2 days post-vaccination and transiently led to the increase of plasma cortisol, insulin, leptin, and haptoglobin levels (Klasing and Korver Citation1997; Houseknecht et al. Citation1998). Hay and total dry matter intake (DMI) reduced on dpv 1 and 2 in the beef heifers vaccinated against respiratory pathogens (Rodrigues et al. Citation2015). A significant decrease in average daily weight gain and DMI was recorded after the administration of inactivated oil adjuvanted FMD vaccine (Decivac FMD DOE; Intervet/Schering-Plough Animal Health, Boxmeer, Netherlands) in Korean goat (Jo et al. Citation2014).
Despite the long-term benefits of the FMD control program, there is a growing concern among the milk producers, vaccinators and bovine practitioners on the adverse effects of FMD vaccination on the production and reproduction in India and other countries (Ferreira et al. Citation2016; Marqués et al. Citation2019) that can adversely affect the voluntary participation of the cattle owners and may eventually lower the herd immunity to prevent the outbreak of FMD. Based on the retrospective analysis of milk yield for 5 years, we found that FMD vaccination caused a transient non-significant drop in the milk yield on the first two days post-vaccination in Deoni and crossbred cows (Krishnaswamy et al. Citation2021). As milk yield and milk fat are inversely related, it is likely that FMD vaccination may affect the milk composition. The varying degree of inimical effects of different types of FMD vaccine on the production is due to the type of adjuvant used in the inactivated vaccine that induces febrile response (Ferreira et al. Citation2016). Therefore, we investigated the short-term effects of inactivated oil adjuvanted FMD vaccine on the health and production parameters in the lactating crossbred cow.
2. Materials and methods
2.1. Farm location and management
The study was conducted on lactating crossbred cows (Holstein-Friesian × Bos indicus) maintained at an organized government farm. The detailed information about the farm practices is presented elsewhere (Krishnaswamy et al. Citation2021).
2.2. Experimental animals and design
Crossbred milch cows (n = 12) were selected based on the age, parity and days in milk and were divided into two groups (n = 6/group). The age ranged from 35.1 to 101.2 months with a mean of 67.6 months and the parity ranged from one to three. Days in milk ranged from 64 to 180 days. Before the commencement of the experiment, a general health checkup was done by a qualified veterinarian and subclinical mastitis was ruled out for the selected cows. A crossover experimental design was adopted. One group received vaccination with commercially available trivalent (covering serotype O, A, and Asia1) oil-adjuvanted FMD vaccine (Raksha Ovac-Trivalent,), while the other group was mock-vaccinated with 2 mL saline intramuscular (Trial #1). After a cooling period of two weeks to overcome the residual effects of FMD vaccination on the dependent variables, the same trial was repeated such that the unvaccinated group in the first trial was administered the FMD vaccine, while the other group served as mock injected control (Trial #2). The cross-over design eliminated the variations due to the cow as each experimental unit was exposed to both the treatment (FMD vaccination or mock vaccination). Further, the experimental design accounted for the day-to-day variation in the farm management that influence the milk yield and other dependent variables of the study. The experimental cows were stall-fed during the whole period of the experiment for recording the dry matter intake (DMI), rectal temperature and collecting blood. An acclimatization period of 7 days was given for stall-feeding before the actual commencement of trial as the cows were maintained under a semi-intensive system of management. Cows were observed for 1 d before FMD vaccination and 3 days post-vaccination (dpv −1 to +3). Day of FMD vaccination was considered dpv 0. A booster dose of the FMD vaccine was administered on dpv 28. Approximately 10 mL of blood was collected into a vacutainer (Becton Dickinson Vacutainer Systems, Plymouth, UK) from dpv −1 to +3. Immediately after collection, about 0.5 mL was withdrawn to estimate blood glucose using glucometer strips (Accu-check Active, Roche). In parallel, 1 mL of blood was collected in an anticoagulant coated vacutainer for a complete blood count. In addition, sera samples were collected on dpv 28 and 42 for estimation of FMD specific neutralizing antibodies. Sera were stored at −20°C until assay.
2.3. Virus neutralization test (VNT)
The sera collected on dpv 0, 28, and 42 were assessed for the immune response to FMD vaccination in terms of neutralizing antibody titre using VNT (OIE Citation2018). Briefly, heat-inactivated sera samples were serially diluted two-fold from 23 to 210 in GMEM (each sample in duplicate) in 96 well cell culture plates to which 100 TCID50 of FMDV (O, A and Asia1) was added and incubated for 1 h at 37°C; after 48 h, the cytopathic effect was assessed and titre of antibody was calculated as log10 of the highest dilution of serum that neutralized 50% of the infected wells (log10VN50). Back titration of 100 TCID50 virus was included in each run to decide on the acceptability of the results (Sperman Citation1908;Kärber Citation1931).
2.4. Body temperature and dry matter intake (DMI)
The rectal temperature of the experimental cows was recorded using clinical thermometer from dpv −1 to +3. Daily voluntary feed intake of each crossbred cow was recorded from dpv-1 to dpv + 3. Weighed quantity of feed was offered daily twice at 09:30 and 16:00 h. The residues were collected and weighed individually at 09:00 h on the next day. Representative samples of concentrate, green and dry fodder were submitted for proximate analysis at ICAR-National Institute of Animal Nutrition and Physiology, Bengaluru. Dry matter intake/cow/day was expressed in percent.
2.5. Complete blood count and serum biochemistry
Haematological parameters such as red blood cell (RBC) count, Haemoglobin (Hb), packed cell volume (PCV), total leukocyte count (TLC), and the differential count was determined using a haematology autoanalyzer (Mindray BC-2800) from dpv −1 to dpv +3. Estimation of total protein, albumin, blood urea nitrogen, creatinine, triglycerides and total cholesterol was done by semi-autochemistry analyzer (Mindray, BA-88A) from the sera collected on dpv −1 to +3.
2.6. Assay of bovine haptoglobin and cortisol
Sera collected from dpv −1 to +3 were used for the assay of bovine haptoglobin and cortisol. Haptoglobin was assayed using a commercial ELISA kit (Cat #PG-0022Bo, Genetix Biotech Asia Private Limited, India) as per the instructions of the manufacturer. The intra and inter-assay coefficient of variation (CV) were <8% and <10%, respectively. Cortisol was assayed using a radioimmunoassay kit (Cat # IM1841, Immunotech, France) at ICAR-National institute of animal nutrition and physiology, Bengaluru as per the instructions of the manufacturer. The intra and inter-assay coefficient of variation (CV) were <5.8% and <9.2%, respectively.
2.7. Milk production and composition
An aliquot of 20 mL of milk sample was collected from each cow after morning milking for the estimation of milk constituents such as fat, solids not fat (SNF), protein and lactose was done using milk analyzer (Vector, India). In addition, milk yield (kg/cow/day) was recorded in an electronic weighing balance with a precision of 100 g.
2.8. Statistical analysis
For VNT, the endpoint titre based on the serial dilutions of the sera was log10 transformed to make it linear and the log10VN50 antibody titre of each FMDV serotype and was analyzed by one-way repeat measures ANOVA with orthogonal contrast with dpv 0 using Bonferroni test. Data on milk yield and its constituents were corrected for confounding factors such as age, parity and stage of lactation using least-squares analysis of variance (Harvey Citation1990). The dependent variables such as body temperature, DMI, serum haptoglobin, cortisol haematological parameters, milk yield and milk constituents were analyzed by mixed-model ANOVA by fitting the treatment (FMD or mock vaccination) and dpv (−1 to +3) as fixed effects and cow as a random effect. Data are presented as mean ± standard error (n). Data were analyzed using Statistical Package for the Social Sciences (SPSS) version 22 and charts were prepared using GraphPad Prism 5.0.
3. Results and discussion
A significant increase in the neutralizing antibody response (log10VN50) was recorded for FMDV serotype O (p < 0.001) and Asia1 (p < 0.027) on dpv 28 as compared with dpv 0; however, it was non-significant for serotype A (p < 0.228). Following booster on dpv 28, the antibody titre did not increase on dpv 42 for all three serotypes. Among all the three serotypes, the basal antibody titre showed a maximum value of 2.6 log10VN50on dpv 0 for FMDV serotype O, while it was about 1.4–1.6 log10VN50 for the other two serotypes (Supplementary Figure 1). Higher antibody titre for FMDV serotype O than that of other serotypes on dpv 28 is in accordance with the earlier report in crossbred cows that were vaccinated with inactivated trivalent oil adjuvanted FMD vaccine (Gowane et al. Citation2013). Higher antibody titre on dpv 0 might be due to regular and repeated vaccination of the pluriparous animals or inapparent infection with FMDV (Mohan et al. Citation2009) as inactivated FMD vaccine do not mount sterile immunity.
No interaction was observed between vaccination and dpv on the rectal temperature; however, the main effect of vaccination showed an extremely significant (p < 0.000) increase of 0.4° to 0.6°C increase in the body temperature in the FMD vaccinated crossbred milch cows as compared with the mock vaccinated group. A lack of interaction between the vaccination group and dpv suggested a lack of simple effect of temperature on dpv ((A)). The results of FMD vaccination on the body temperature is in agreement with previous studies (Ferreira et al. Citation2016; Kim et al. Citation2021). Immunization of Hanwoo (Bos taurus coreanae) beef cows with FMD vaccine (Bioaftogen, FMDV vaccine O1 Campos, A24 Cruzeiro and A2001 Argentina; Biogenesis Bago, Buenos Aire) increased the ruminal temperature for >20 h post-vaccination (Kim et al. Citation2021). A significant rise in the temperature was observed (OurovacAftosa; OurofinoSaude Animal, Cravinhos, Brazil, 5 mL subcutaneous) in Nelore beef cows (Bos indicus) maintained in Brazil at 24 h post-FMD vaccination (Ferreira et al. Citation2016). The febrile response to FMD vaccination is mediated through the adjuvant that is intended to stimulate the innate immune response. In India, there are three commercial firms that produce FMD vaccine with common FMDV strains but unique adjuvant, that is proprietary in nature.
Figure 1. Effect of FMD vaccination on the acute phase response in the crossbred cow. From dpv −1 to +3, (A) rectal temperature and (B) dry matter intake (DMI) were recorded. Further, (C) serum haptoblobin and (F) cortisol were assayed using commercial kits. Complete blood count revealed a significant increase in the (D) total leukocyte count and (E) neutrophils till 48 h in the FMD vaccinated cows. Data was analyzed by linear mixed model ANOVA. A significant increase in the rectal temperature, DMI and haptoglobin on dpv 1 in the FMD vaccinated group was suggestive of acute phase response. Cortisol concentration was significant on dpv 2 in the FMD vaccinated group.
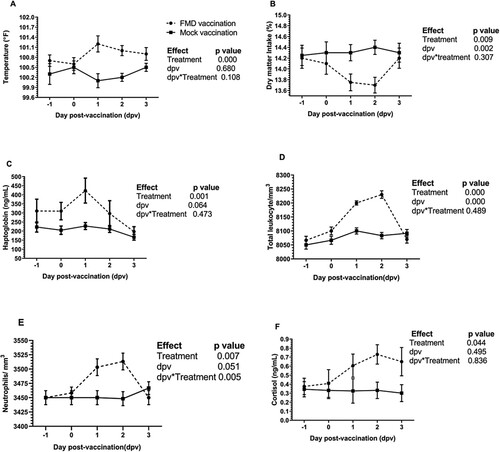
Administration of FMD vaccine significantly reduced the DMI by ∼ 0.7–0.8 kg till 48 h post-vaccination (p = 0.009; (B)). In support of our findings, a reduction in the forage intake and total nutrient intake has been reported following FMD vaccination in the Korean goats (Jo et al. Citation2014). The reduction in the feed intake following vaccination is likely due to acute phase response as evidenced by increased plasma haptoglobin levels in the feedlot cattle (Ferreira et al. Citation2016).
Serum haptoglobin, which is a well-recognized marker of acute phase response in the cow (Eckersall and Bell Citation2010) showed a significant increase in the FMD vaccinated group (p = 0.001; (C)). A comparable observation was made in the Nelore beef cows following vaccination with bivalent oil adjuvanted FMD vaccine (Ferreira et al. Citation2016) was elevated from dpv 3 to 5 in Nelore beef cows vaccinated with bivalent oil adjuvanted FMD vaccine.
Of all the haematological variables, only the total leukocyte count (p = 0.000) and neutrophil count (p = 0.007) showed a significant rise in the crossbred cows that received the FMD vaccine as compared with mock-vaccinated group ((D,E)). Further, a significant increase in the neutrophil count in the FMD vaccinated group on dpv 1 and 2 indicated an interaction effect. The main effect of vaccination on serum cortisol showed a significant increase in the FMD vaccinated crossbred cows (p = 0.04). The serum cortisol concentration was significant on dpv2 in the FMD vaccinated group ((F)) suggesting a stress response to the vaccination. However, the changes in the other haematological and basic serum biochemistry variables were comparable between the two groups (Supplementary Table 1 and 2; p > 0.05). There was no significant difference between the groups. Collectively, the results suggested that the FMD vaccination stimulated the innate immune response as evidenced by the febrile response, increased concentration of serum haptoglobin, mild neutrophilia, and leukocytosis. An increase in the total leukocyte count and neutrophil count in the FMD vaccinated group on dpv 1 and 2 is in agreement with the results reported in feedlot cattle (Seo et al. Citation2019) and Korean goat (Jo et al. Citation2014) and could be due to the effect of adjuvant (Carroll and Forsberg Citation2007).
In parallel, a stress response to vaccination was also recorded as evidenced by increased cortisol. Vaccinating beef cattle against bovine respiratory disease pathogens using a modified live vaccine (2 mL subcutaneous, Bovi-Shield Gold One Shot; Zoetis) increased the concentration of plasma cortisol from 2 to 16 h post-vaccination (Rodrigues et al. Citation2015). Vaccination is known to be one of the management factors responsible for increasing cortisol concentration (Kim et al. Citation2011).
As the interaction effect between the treatment and dpv was non-significant for the milk yield and its composition ((A–E)), the main effect was considered for further analysis. The effect of FMD vaccination was non-significant for all the milk constituents except milk fat (p = 0.053). The effect of dpv showed a significant influence on the milk yield (p < 0.0001), but no effect on any of the milk constituents. Though a decline in the milk yield was observed in both the groups, the FMD vaccinated group showed a steep negative slope from dpv 0 to dpv1 as compared with the mock vaccinated group, indicating an acute reduction of about 1 kg in the milk yield ((A)). In the mock vaccinated group, the decline was not as steep as in the FMD-vaccinated group. Though the treatment effect was non-significant (p = 0.408), the reduction in the milk yield within 24 h post FMD vaccination is of economic importance, especially for the small and marginal milk producers. In the absence of vaccination effect, a significant influence of dpv on the milk yield could not be explained convincingly. Our results are consistent with the previous study (Krishnaswamy et al. Citation2021), where a mild non-significant decline in milk yield in crossbred was observed after administration of FMD vaccine (Raksha-Ovac trivalent vaccine, Indian Immunologicals Limited, 2 mL intramuscular). A mild, but transitory drop-in milk yield was recorded after vaccination of HF dairy cows with a killed vaccine containing BHV1, BVDV, PI-3 V, BRSV, and 5-way leptospiral bacterin (Sentry 9; Boehringer Ingelheim Burlington, Ontario, Canada) (Scott et al. Citation2001).
Figure 2. Effect of FMD vaccination on the least squares mean (LSM) of milk yield and its composition in the crossbred cow. Milk yield and composition data was corrected for the stage of lactation, age and parity using Harvey’s least squares analysis. Within 24 h post-vaccination, the corrected milk yield showed a decline of ∼1 kg in the vaccinated group. Of all the constituents tested, only corrected milk fat showed a significant, but slight decrease suggesting a negative effect of FMD vaccination.
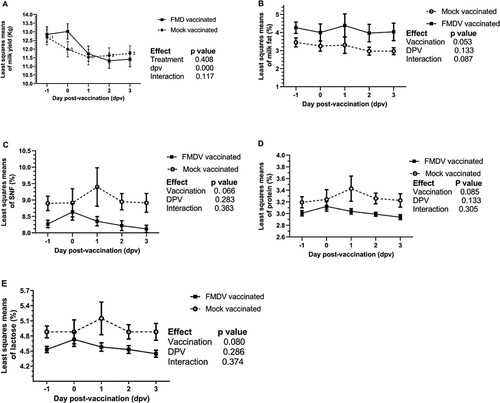
It was concluded that increased body temperature and decreased DMI to FMD vaccination within dpv 2 was mediated through acute phase response; however, it did not affect the milk composition and milk yield significantly.
Supp_Tables_06Dec2022
Download MS Word (228.5 KB)Disclosure statement
No potential conflict of interest was reported by the author(s).
Data availability statement
The data will be shared upon reasonable request.
Additional information
Funding
References
- Annual report 2017-18 Department of Animal Husbandry, D. and F.M. of A. and F.W.G. of I. 2018. Chapter 3: Animal Husbandry. Published by Department of Animal Husbandry, Dairying and Fisheries, Ministry of Agriculture and Farmers Welfare, Government of India. p. 21–52.
- Ansari-Lari M, Mohebbi-Fani M, Lyons NA, Azizi N. 2017. Impact of FMD outbreak on milk production and heifers’ growth on a dairy herd in southern Iran. Prev Vet Med. doi:10.1016/j.prevetmed.2017.05.022.
- Carroll JA, Forsberg NE. 2007. Influence of Stress and Nutrition on Cattle Immunity. Vet Clin North Am Food Anim Pract. 23:105–149. doi:10.1016/j.cvfa.2007.01.003.
- Chaters G, Rushton J, Dulu TD, Lyons NA. 2018. Impact of foot-and-mouth disease on fertility performance in a large dairy herd in Kenya. Prev Vet Med. doi:10.1016/j.prevetmed.2018.08.006.
- Eckersall PD, Bell R. 2010. Acute phase proteins: biomarkers of infection and inflammation in veterinary medicine. Vet J. doi:10.1016/j.tvjl.2010.04.009.
- Ferreira LCL, Cooke RF, Marques RS, Fernandes HJ, Fernandes CE, Stelato R, Franco GL, Lemos RAA. 2016. Effects of vaccination against foot-and-mouth disease virus on reproductive performance of Bos indicus beef cows. J Anim Sci. doi:10.2527/jas.2015-9537.
- Gowane GR, Sharma AK, Sankar M, Thirumurugan P, Narayanan K, Subramaniam S, Pattnaik B. 2013. Evaluation of genetic and environmental parameters determining antibody response induced by vaccination against foot and mouth disease. Agric Res. doi:10.1007/s40003-013-0063-9.
- Harvey WR. 1990. User’s guide for LSMLWMand MIXMDL PC-2 version. Mixed ModelLeast-Squares and Maximum Likelihood Computer Programme.
- Houseknecht KL, Baile CA, Matteri RL, Spurlock ME. 1998. The biology of leptin: a review. J Anim Sci. 76:1405–1420. doi:10.2527/1998.7651405x.
- Jo NC, Jung J, Kim JN, Lee J, Jeong SY, Kim W, Sung HG, Seo S. 2014. Effect of vaccination against foot-and-mouth disease on growth performance of Korean native goat (Capra hircus coreanae). J Anim Sci. doi:10.2527/jas.2014-7190.
- Kärber G. 1931. Beitrag zur kollektiven behandlung pharmakologischer reihenversuche. Naunyn Schm Arch Exp Pathol Pharm. 162:480–483. doi:10.1007/BF01863914.
- Kim D, Moon J, Ha J, Kim D, Yi J. 2021. Effect of foot-and-mouth disease vaccination on acute phase immune response and anovulation in Hanwoo (Bos Taurus coreanae). Vaccines. 9:1–9. doi:10.3390/vaccines9050419.
- Kim M-H, Yang J-Y, Upadhaya SD, Lee H-J, Yun C-H, Ha JK. 2011. The stress of weaning influences serum levels of acute-phase proteins, iron-binding proteins, inflammatory cytokines, cortisol, and leukocyte subsets in Holstein calves. J Vet Sci. 12:151–157. doi:10.4142/jvs.2011.12.2.151.
- Klasing KC, Korver DR. 1997. Leukocytic cytokines regulate growth rate and composition following activation of the immune system. J Anim Sci. 75:58–67. doi:10.2527/animalsci1997.75Supplement_258x.
- Krishnaswamy N, Jeyakumar S, Selvan RPT, Gowane GR, Mahadappa P, Vijayapillai U, Dechamma HJ, Patel BHM, Saravanan P, Ramesha KP, Sanyal A. 2021. Short-term effect of foot-and-mouth disease (FMD) vaccination on the milk yield in the Deoni and crossbred cows. Trop Anim Health Prod. 53:217. doi:10.1007/s11250-021-02653-y.
- Lyons NA, Alexander N, Stärk KDC, Dulu TD, Sumption KJ, James AD, Rushton J, Fine PEM. 2015. Impact of foot-and-mouth disease on milk production on a large-scale dairy farm in Kenya. Prev Vet Med. 120:177–186. doi:10.1016/j.prevetmed.2015.04.004.
- Marqués FJ, Battistessa EI, Peek SF, Raabis SM, Darien BJ. 2019. The effect of foot-and-mouth disease vaccination on early pregnancy loss in beef heifers in Argentina. Prev Vet Med. 170:104716. doi:10.1016/j.prevetmed.2019.104716.
- Mohan MS, Gajendragad MR, Kishore S, Gopalakrishna S, Singh N. 2009. Kinetics of immune response to foot-and-mouth disease virus (type Asia 1) in experimental cattle. Vet Res Commun. 33:103–109. doi:10.1007/s11259-008-9076-4.
- OIE. 2018. Foot and mouth disease chapter 3.1.8.
- Rodrigues MC, Cooke RF, Marques RS, Cappellozza BI, Arispe SA, Keisler DH, Bohnert DW. 2015. Effects of vaccination against respiratory pathogens on feed intake, metabolic, and inflammatory responses in beef heifers1. J Anim Sci. 93:4443–4452. doi:10.2527/jas.2015-9277.
- Scott HM, Atkins G, Willows B, McGregor R. 2001. Effects of 2 commercially-available 9-way killed vaccines on milk production and rectal temperature in Holstein-Friesian dairy cows. Can Vet J. 42(10):793–798.
- Seo J, Song M, Jo N, Kim W, Jeong S, Kim J, Lee S, Seo S. 2019. The co-injection of antioxidants with foot-and-mouth disease vaccination altered growth performance and blood parameters of finishing Holstein steers. Asian Australas J Anim Sci. doi:10.5713/ajas.18.0609.
- Singh B, Prasad S, Sinha DK, Verma MR. 2013. Estimation of economic losses due to foot and mouth disease in India. Indian J Anim Sci. 83(9):964–970.
- Sperman C. 1908. The method of ‘right and wrong cases’ (‘constant stimuli’) without Gauss’s formulae. Br J Psychol. 2:227–242. doi:10.1111/j.2044-8295.1908.tb00176.x.
- Yeruham I, Yadin H, Haymovich M, Perl S. 2001. Adverse reactions to FMD vaccine. Vet Dermatol. doi:10.1046/j.0959-4493.2001.00221.x.