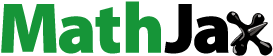
ABSTRACT
The present study was designed for biomonitoring of heavy metals such as lead (Pb), cadmium (Cd), chromium (Cr), zinc (Zn), nickel (Ni), manganese (Mn) and cobalt (Co) at three selected wildlife parks of Punjab. Soil, water and feed samples analysis revealed the high heavy metals contents in feed (21.59 ± 27.39 and 12.67 ± 23.13) followed by soil (11.80 ± 25.84 and 4.96 ± 10.95) and water (2.93 ± 4.48 and 1.11 ± 1.23) samples at Jallo Wildlife Park and Wildlife Park Bahawalnagar, respectively. At Wildlife Park Murree, heavy metals contamination was recorded highest in feed (10.45 ± 16.35), followed by water (6.26 ± 11.35) and soil samples (5.75 ± 9.54). Feathers samples at all sites showed the highest levels of heavy metals concentrations as 12.21 ± 25.69 at Jallo Wildlife Park, 11.50 ± 25.63 at Wildlife Park Bahawalnagar and 3.35 ± 4.81 at Wildlife Park Murree. Interspecific variation of heavy metals among samples and sites was recorded at P < 0.01, and bioaccumulation of heavy metals in the feathers of captive birds was found in high concentration, making them a good indicator of biomonitoring. In captivity, the birds feeding on homogenous diets and particular environmental conditions can better allow us to understand the bioaccumulation of polymetallic contamination, which can further be generalized to predict the risk of these toxic pollutants on public health.
Introduction
Natural biomonitors are used to evaluate the ecosystem integrity and effects of ecological variability on the human population. Organisms can be used as a bioindicator to predict the quality of the environment or as a biomonitor to estimate the degree of environmental contamination quantitatively in a particular area (Markert Citation2007; Parmar et al. Citation2016). Depending on the trophic level, nutrition, size and age, organisms that live in an ecosystem have varying levels of pollution contamination. Other than physiological factors of the organism, environmental factors such as temperature, latitude and physiochemical characteristics also influence the bioaccumulation of pollutants like heavy metals, which tend to accumulate instead of decomposition because of their non-biodegradable nature (Soliman et al. Citation2022).
Heavy metals are naturally occurring elements that are found throughout the earth’s crust but most of the environmental contamination and human exposure result from anthropogenic activities. Their excessive usage in everyday life and unrestrained discharge into the environment has reached a point where the negative impacts are certain. High levels of heavy metals distort the environmental quality and ecological integrity that are necessary to preserve biodiversity (Naz et al. Citation2021). Even the essential heavy metals, which are required for many biochemical reactions, could become toxic depending on the exposure dose, mode and oxidation state. Therefore, with the increasing public health concerns regarding heavy metals contamination, there is a critical need for surveillance and evaluation of the management and remediation of these inorganic xenobiotics (Arooj et al. Citation2023).
Avian species have widely been used as biomonitors of environmental contamination and are a proven invaluable source of ecotoxicological studies (Siddig et al. Citation2016). Unlike mammals, birds have a higher basal metabolic rate, which makes them sensitive to environmental changes and more effective antioxidant systems for scavenging free radicals either endogenous or exogenous which make them resistant to ecological variability and environmental pollution (Sanderfoot and Holloway Citation2017). Conventionally, in captivity, pollution-monitoring methods have mainly been relied on internal tissues and organs samples of birds, but lately, analysis of faeces, blood and feathers for the presence of heavy metals is thought to be a non-destructive method (Shehzad et al. Citation2022). These methods could provide critical information regarding chronic and acute toxic effects of heavy metals and captivity stress on birds, such as neurotoxicity, multiple organ failure and generation of free radical which stimulate oxidative stress impairing proteins, lipids and DNA (Borghesi et al. Citation2017; Naz et al. Citation2020).
Therefore, the aim of this study is to analyse the levels of heavy metals in Indian peafowl (Pavo cristatus) as a model animal at wildlife parks in urban areas. They could be an excellent model to study heavy metals bioaccumulation through diet. In captivity, they were usually fed with homogeneous diet mainly with poultry feed, which is also a limiting factor to their welfare and health, as compared to their wild counterparts with a diverse range of food (Woods et al. Citation2022). So, the analysis of diet and bird could give a more specific estimation of heavy metals as there is an association between heavy metals levels in diet and their detected levels in the body of birds as reported in the literature (Falkowska et al. Citation2013; Markowski et al. Citation2013; Squadrone et al. Citation2018). Assessment of blood gives information about the recent exposure of heavy metals directly linked to diet, while feathers provide long-term contamination. Feathers reflect endogenous heavy metals levels from diet during their growth period (heavy metals incorporated in the keratin structure) until moulting and long-term exogenous exposure from its environment (Borghesi et al. Citation2017; Furtado et al. Citation2019).
The concentration of heavy metals in the environment perseveres for a long time due to their non-biodegradable nature. Heavy metals from anthropogenic sources have a weak association with their natural concentration in soil/sediments, so the heavy metals from the anthropogenic sources are likely to be more bioavailable and bioaccumulative than those from natural sources (Migani et al. Citation2015; Borghesi et al. Citation2017; Rutkowska et al. Citation2018). Analysis of soil and water samples can indicate the alteration of the natural profile of heavy metals and the rate of contamination. Environmental biomonitoring can indicate how the natural profiles of heavy metals in the environment and animals are being affected. Therefore, the current study is a prerequisite to measure the exposure, uptake and bioaccumulation of heavy metals to develop enhanced management practices and mitigation of these toxicants.
Materials and methods
This present research was conducted with permission from was granted by office of Director General Wildlife and Parks, Punjab, for research sampling at three wildlife parks.
Sampling
Three different wildlife parks of Punjab i.e. Jallo Wildlife Park, Lahore, Wildlife Park Bahawalnagar and Wildlife Park Murree were taken into consideration for the research study (). Jallo Wildlife Park is located in Lahore, central Punjab, which is the second largest metropolitan city of Pakistan at an elevation level of 217 m above the sea level. Its climate is steppe hot with dry winter according to the BWhw-Köppen climate classification and been ranked as ‘the most polluted city in the world’ with the rising pollution levels every year, far above the recommended levels of WHO (Ali et al. Citation2022). Wildlife Park Bahawalnagar is located in Bahawalnagar, southern Punjab, at an elevation level of 163 m above the sea level with climate of desert hot with dry winter per the BWhw-Köppen climate classification. It is relatively underdeveloped and moderate levels of pollution. Murree Wildlife Park is situated in the most-visited hill station Murree in the northern most part of Punjab. It has an elevation of 2291 m from the sea level with warm temperate fully humid environment per the Cwb Köppen climate classification.
A total of 75 feathers samples were collected from three selected sites during March and April 2022. Fully grown tail feathers (n = 32/site) were collected from Indian peafowls at Jallo Wildlife Park, Wildlife Park Bahawalnagar and Wildlife Park Murree. To avoid flight impairment, plucking of feathers from wings is not recommended (Anbazhagan et al. Citation2021). Feathers samples were kept in plastic zip lock bags at 4°C until further analysis. Blood samples from individual birds (n = 25/site) were taken from brachial vein by a 3 ml syringe. Special care was taken while capturing the birds for blood sampling to avoid any injury and unnecessary stress. Wildlife Park staff, trained for bird handling, captured the birds and a veterinary officer took the blood samples. Blood samples were transferred to red top vacutainers immediately and were allowed to stand for blood clotting. The vacutainers were covered with a cloth towel and placed in a handling box at 4°C. Soil samples (n = 15/site) were collected from cage floors, while feed and water samples (n = 15/site) were collected from feeding trays of Indian peafowls in sterilized zip lock bags and bottles, respectively. All of the samples were kept in a handling pack at 4°C for safe transportation.
Sample preparation and analysis
Wide ranges of methods are used to remove external contaminations from the surface of feathers. In the present study, alternate washing technique with an organic solvent and distilled water was used. Feathers were washed three times with diluted acetone and distilled water alternatively ending with deionized water to remove exogenous contaminants (Ansara-Ross et al. Citation2013). The washed feather samples were placed in polypropylene vials individually and oven dried at 60°C for 48 h. Dry weight was determined, and each sample was cut into 1–2 mm pieces with a stainless steel scissor. For digestion, 1 g of feather sample was mixed with 1:1 mixture of nitric acid 65% (HNO3) and hydrogen peroxide 30% (HClO4), and a beaker was placed on a hot plate at 70°C to obtain clear solution. The final extract was increased to 25-ml portion with deionized water and filtered using Whatman filter paper (Anbazhagan et al. Citation2021). Samples were stored at room temperature until further analysis. All the reagents used for the preparation and analysis of samples were of analytical grade (Sigma-Aldrich, USA). Blood samples were allowed to clot in red top vacutainer. For serum extraction, the clotted samples were centrifuged at 2000 rpm for 10 min. Serum samples were stored for prior analysis. One millilitre of serum was diluted with 10 ml de-ionized water for heavy metals quantification (Akan et al. Citation2014). Soil and feed samples (n = 15/site) were oven dried, and 1 g dried sample of feed and soil was added to 15 ml of standard solution (concentrated HNO3 and HClO4; 3:1 ratio) in separate beakers. On the hot plate, samples were heated at 150 ˚C for 30 min and then at 180 ˚C until digestion was completed. The samples were then diluted by 20 ml distilled water and two drops of 30% of hydrogen peroxide (H2O2). The diluted solution was filtered by Whatman filter paper and stored in sterilized borosilicate glass vials till further analysis (Yasmeen et al. Citation2020). A total of 1 ml water sample (n = 15/site) was mixed with 15 ml of HNO3. The procedure was completed by heating the solution on the hot plate to obtain a colourless solution and the dilution with distilled water (20 ml) along with two drops of 30% H2O2. The diluted sample solution was filtered and stored until analysis (Gupta Citation2013). Aurora Al 1200 Atomic absorption spectrometer was used to analyse all of the samples.
Quality assurance and control
To ensure the stability of AAS, a quality control (QC) sample was prepared by mixing aliquots from each sample. After every 15 samples, the QC sample was injected to check the stability of instrument, and the variation of heavy metals concentrations in QC samples was less than 15%. A blank sample solution was used to set the instrument to zero for each sample analysis.
Precision, which is typically expressed as the relative standard deviation (RSD), with a range of 5–10% of the evaluated dataset, was measured less than 10% for each heavy metal in the present study. Different concentrations of standard solutions (Merck), 0.01, 0.1, 0.5 and 1 mg/l, were prepared from the stock solution of 1000 mg/l of each heavy metal separately for plotting the calibration curve.
The precision was evaluated by repeatability, which was determined by plotting at least five calibration curves of known concentration for heavy metal standard solution (1.0 µg/ml) with blank sample solution on the same day and the working solution were prepared on a daily basis. For limits of detection (LOD) and quantification (LOQ), a blank solution was measured 25 times to calculate the standard deviations for the noise levels generated for each heavy metal. Accuracy was determined by the standard addition method and expressed as percent recovery. A total of 100% recovery is preferable, but there is no established value. Percent recovery of each heavy metal in each sample was measured separately with a range of 87.3–106.1%, which was within the acceptable range recommended by experts. The concentration of heavy metal in each sample was determined as the average value of three replicates.
Statistical analysis
Statistical analysis was conducted using IBM’s SPSS (version 21) and OriginPro®2023. Normality and homogeneity of variance of data were checked by Shapiro–Wilk and Levene’s test. Results were significant at P < 0.05, indicating non-normal distribution of data and unequal variances (non-parametric data) for concentrations of heavy metals in all samples. Thus, the data were analysed by non-parametric (distribution free) statistics. The Kruskal–Wallis test was used to test the null hypothesis, whether the concentration levels of heavy metals in all selected samples were similar at the significance level of P < 0.05. It was followed by Dunn’s multiple comparison with Bonferroni adjustment to estimate the mean difference of heavy metals concentrations in each sample. Spearman’s rank correlation was applied to measure the positive or negative relationship between samples and the strength of association between internal heavy metal contents (blood) with feed and water being source of contamination. Also, the correlation between external heavy metals concentration, feather and its surrounding environment/habitat and soil was calculated.
The concentration levels detected by AAS were calculated by following the study by Yasmeen et al. (Citation2020), and the bioaccumulation factor of heavy metals in feathers of Indian peafowl was calculated (DeForest et al. Citation2007; Ali and Khan Citation2019) as follows:
Results
Regional variations of heavy metals concentrations
Heavy metals levels (mean concentration with standard deviation) in feed, water and soil were analysed to estimate the nutrition and environmental quality of the selected wildlife parks. The highest heavy metals content was found in feed samples at all sites as 21.59 ± 27.39 μg/g, 12.67 ± 23.13 μg/g and 10.45 ± 16.35 μg/g at Jallo Wildlife Park, Wildlife Park Bahawalnagar and Wildlife Park Murree, respectively. Water samples from Wildlife Park Murree showed the highest contamination with heavy metals (6.26 ± 11.35 μg/g) and the lowest contamination from water samples of Wildlife Park Bahawalnagar (1.11 ± 1.23 μg/g). In the case of soil, heavy metals concentration levels were found as follows: Jallo Wildlife Park > Wildlife Park Murree > Wildlife Park Bahawalnagar. Concentration levels of each detected heavy metal in soil, feed and water are presented in .
Table 1. Descriptive statistics of heavy metals concentrations (mean ± SD) in soil, feed and drinking water.
Heavy metals concentration levels were detected from blood and feathers of Indian peafowl as an indicator of environmental heavy metal levels. Feather samples showed high concentration of heavy metals compared to blood at each site (). Site-wise comparison revealed heavy metals concentration in feathers from highest to lowest levels as Jallo Wildlife Park > Wildlife Park Bahawalnagar > Wildlife Park Murree, while blood samples showed concentrations of heavy metals from highest to lowest levels as follows: Jallo Wildlife Park > Wildlife Park Murree > Wildlife Park Bahawalnagar. The total concentration levels of each metal from all samples collectively at each selected site are presented in . Overall, the highest prevalent heavy metal at all selected sites was Zn followed by Cr, while the lowest prevalent heavy metal was Co, except for Wildlife Park Bahawalnagar where it was the second lowest concentration level after Cd. The order of heavy metal concentration levels from highest to lowest was as Zn > Cr > Mn > Pb > Ni > Cd > Co at Jallo Wildlife Park and Zn > Cr > Mn > Ni > Pb > Co > Cd and Zn > Cr > Pb > Cd > Mn > Ni > Co at Wildlife Park Murree.
Table 2. Descriptive statistics of internal (blood) and external (feathers) heavy metals concentration (mean ± SD) levels in Indian peafowl.
Interspecific heavy metals concentration from selected sites was found significant at P < 0.05, indicating variation of heavy metals concentration level between samples and sites. Dunn’s test of multiple comparison using rank means with Bonferroni adjustments revealed statistically significant differences between independent samples i.e. feed, water, soil, blood and feathers from each site (online resource). Multiple comparison between samples showed highest variation of Cd concentration at Jallo Wildlife Park. At Wildlife Park Bahawalnagar, the highest variation of Cr and Co concentration was recorded between samples, while Pb and Ni concentration in samples at Wildlife Park Murree had the highest difference among them. Blood and feathers samples at each site showed a highly statistically significant difference at P < 0.001 between all heavy metal concentrations except for Ni levels between blood and feathers at Jallo Wildlife Park.
Relationship of heavy metals and Indian peafowl
A Spearman's rank correlation (rs) indicated a significant correlation between heavy metals concentration of blood with feed and water at all sites. The correlation was scaled (+ or −) as negligible (rs = 0–0.19), weak (rs = 0.20–0.39), moderate (rs = 0.4–0.59), strong (rs = 0.60–0.79) and very strong (rs = 0.80–1.00). A strong positive correlation of blood with feed samples at all sites was recorded, which indicates that with an increase in heavy metal content in feed, the heavy metals concentration in blood also increases. Heavy metals contents in water showed negligible to moderate correlation with blood samples at each site. Inter-specific heavy metals concentration correlation (positive and negative) in blood, feed and water is presented in online resource 2. Significant correlations of blood heavy metals levels at P < 0.05, which have a linear relation with feed and water are plotted () for all sites, show the increase in heavy metals levels in blood with an increase in feed and water.
Correlation between feathers and soil samples was also measured to find out whether Indian peafowl feathers acquire heavy metals from its habitat. For the present study, soil samples were used to compute the correlation with feather’s heavy metals contents. The results indicated negligible to strong positive association between feather and soil samples at all sites (Online resource 3). Significant correlations of feather heavy metals levels (P < 0.05) with soil are plotted below () indicating the increase in heavy metals levels in feathers with an increase in soil.
Bioaccumulation of heavy metals
To find the accumulation levels of heavy metals, feathers and soil heavy metals concentrations were used to calculate the bioaccumulation factor (BAF). BAF is the association between the organism and its surrounding environment and illustrates the site-specific contamination rate. BAF value of more than 1 indicates the accumulation of heavy metals in the organism more than its surrounding medium. High BAF values were recorded from all sites especially the non-essential heavy metals i.e. Pb and Cd. Most of the detected heavy metals at Jallo Wildlife Park had BAF values of more than one followed by Wildlife Park Murree and Wildlife Park Bahawalnagar ().
Discussion
An exponential rise in the human population and the development of new residential areas increase the anthropogenic-induced pressure and pollution on the wildlife parks. Improvement and developmental projects such as construction of enclosures for new captive animals also exert negative effects on captive species (Biaza Citation2006). Unlike mammals, birds have a higher basal metabolic rate and more effective antioxidant systems for scavenging metabolism-induced free radicals (Sanderfoot and Holloway Citation2017). The present study documents the distribution and accumulation of heavy metals in the environment and in the body of Indian peafowl at selected Wildlife Parks of urban areas. Moreover, this assessment also demonstrates the suitability of birds as a bioindicator for monitoring the effects of environmental pollution. A study conducted at Lahore reported the values as follows: Pb 14.89 mg/kg, Cd 22.5 mg/kg, Cr 2242.5 mg/kg, Zn 19.53 mg kg (Muhammad et al. Citation2022), Ni 40.89 mg/kg and Co 27.25 mg/kg in contaminated soil, which were much higher levels than the present study, while in groundwater, heavy metals were recorded as Pb 0.24 mg/kg, Cd 0.06 mg/kg, Cr 1.21 mg/kg, Zn 0.10 mg/kg, Ni 0.13 mg/kg and Co 0.13 mg/kg, which are lower than present study (Murtaza and Usman Citation2022). Results from the adjacent area of Murree were similar to the present study in water samples of Wildlife Park Murree: Pb 2.97 mg/l, Cr 8.03 mg/l, Mn 17.6 mg/l, Ni 5.18 mg/l and Co 8.09 mg/l (Muhammad et al. Citation2022), while Ali et al. (Citation2016) reported less concentration levels of heavy metals in water samples from Murree: Pb (0.08 ppm), Cd (0.07 ppm) and Cr (0.08 ppm). A study conducted in Himalayan foothill including Murree region reported all of the heavy metals detected from the present study in soil and dust samples along the roadside with concentration levels as follows: Zn, 54.6 and 26 μg/g; Pb, 26.7 and 28 μg/g; Mn, 16.4 and 92 μg/g; Cd, 4.6 and 0.7 μg/g; and Ni, 18 and 11.3 μg/g (Salim et al. Citation2020). High concentration levels detected in feed at all sites have also been reported by many other studies. Heavy metals concentrations in feed were determined by Bukar and Sa Citation2014 as follows: Cd, 0.53–3.19 mg/kg; Co, 0.13–3.33 mg/kg; Mn, 12.50–37.50 mg/kg; Ni, 1.03–2.06 mg/kg; Pb, 0.27–0.80 mg/kg; Cr, 0.47–2.38 mg/kg; and Zn, 16.30–38.04 mg/kg. Another feed analysis (Ukpe and Chokor Citation2018) also estimated similar concentration levels of heavy metals, i.e. Pb, 1.17 g/kg; Ni, 1.71 g/kg; Cr, 0.529 g/kg; Co, 0.39 g/kg; Cd, 0.031 g/kg. High levels of heavy metals in feed might be the result of feed production process, transportation, storage conditions, or contamination from soil or water (Jothi et al. Citation2017). A study conducted on blood samples of Mallards recorded heavy metal accumulation (Binkowski and Sawicka-Kapusta Citation2015) with concentrations of Pb of 0.69 µg/g, Zn of 24.470 µg/g, Cr of 4.77 µg/g and Ni of 3.7 µg/g, which are in accordance with the current study. Another study reported varying results from the present study, and concentration levels of Pb of 0.78 µg/g, Cd of 0.04 µg/g and Zn of 29 µg/g were found in blood samples of pied flycatchers by Berglund (Citation2018). All of the selected sites are situated in the suburban areas of cities in Central (Jallo Wildlife Park), South (Wildlife Park Bahawalnagar) and North (Wildlife Park Murree) Punjab, with different managerial settings and varying pollution levels. A study in the same regions was done by Nighat et al. (Citation2013) for the estimation of heavy metals in the feathers of three families of wild birds. In Northern Punjab, Cd concentration was found in a range of 0.82–4.04 μg/g, which is in line with the present study at Wildlife Park Murree: 0.83 μg/g, while Pb (6.09–32.89 μg/g), Zn (90.75–786.06 μg/g) and Ni (33.58–120 μg/g) were much higher than the concentration of Pb (1.43 μg/g), Zn (7.29 μg/g) and Ni (0.65 μg/g) in feathers of Indian Peafowl from the present study. The high levels of heavy metals residues in the reported study might be the result of the multiple sources of contamination from different regions to those free-ranging birds, while the bird species from the present study are confined in a specific area of Wildlife Park which reflects the heavy metals levels of its surroundings, which is clearly shown in the results (). Higher concentration levels of heavy metals than recorded from Jallo Wildlife Park, Lahore, were recorded in feathers of cattle egrets from highly contaminated areas of Lahore and Sialkot (Abdullah et al. Citation2015) as Pb, 297 and 286 µg/g; Cd, 41 and 37.6 µg/g; Cr, 21.1 and 19 µg/g; Zn, 529 and 226 µg/g; Ni, 41.6 and 84.8 µg/g; and Mn, 16 and 21.9 µg/g. Heavy metals levels in feathers of Laggar falcon in Bahawalnagar were reported previously (Movalli Citation2000) as follows: Cd, 0.12 ppm; Pb, 1.08 ppm; Co, 1.06 ppm; Zn, 104.13 ppm; and Cr, 1.72 ppm with comparison to the present study in which Cd (0.39 µg/g), Cr (6.45 µg/g) were higher, while Pb (0.75 µg/g), Co (0.56 µg/g) and Zn (69.42 µg/g) were found in lower concentrations in feathers of Indian peafowl. Bioaccumulation rates of detected heavy metals were also found to be highest at Jallo wildlife Park. Bioaccumulation rates of Pb 2.57 µg/g, Cd 6.49 µg/g, Cr 1.16 µg/g, Zn 4.29 µg/g, Ni 1.15 µg/g and Mn 0.10 µg/g were reported in polluted area of Lahore by Abdullah et al. (Citation2015), which relates to the results of the present study with BAF values of most heavy metals higher than one indicating high contamination levels. Squadrone et al. (Citation2018) reported bioaccumulation of heavy metals in the feathers of African penguin as follows: Pb, 0.56 ± 0.38; Cd, 0.33 ± 0.2; Zn, 98 ± 32; Cr, 22 ± 77; Ni, 58 ± 45; Mn, 15 ± 17; and Co, 0.16 ± 0.1, which correlates with the present study. The difference between the concentration levels of the present study and other studies is because of the birds having species-specific detoxification mechanisms (Lucia et al. Citation2012) and varying contamination levels of the selected sites.
Blood as indicator
Heavy metals levels in the blood indicate recent contamination directly linked to feeding. Nutrients are distributed to different organs and body parts by blood, and so there is more steady heavy metals concentration in blood than in other tissues (Carvalho et al. Citation2013). A homogeneous diet of birds living in the captivity results in chronic exposure to even low doses of heavy metals, and this is reflected by high concentrations of these heavy metals in blood (Falkowska et al. Citation2013). The concentrations of heavy metals in blood, feed and water were associated with the present study (linear relationship), signifying the value of measuring contaminant exposure in blood by feed and water samples in which high heavy metals concentrations were recorded. The variations between heavy metals concentrations in blood between selected sites are because of the difference between pollution levels, climatic conditions and management of the selected parks, while a difference within samples of each site showed the physiological variability of examined birds. A study on seabirds showed that birds feeding on cephalopods and krill had higher levels of Cd than the birds feeding on fish because the cephalopods and krill lived in Antarctic ecosystems having high levels of Cd associated with natural sources (Carvalho et al. Citation2013). In another study (Falkowska et al. Citation2013), a diet with different mercury (Hg) levels was used to analyse its accumulation and detoxification from the body of captive Penguins. The present study showed that blood could be used as a reliable biomarker for determining the recent exposure to heavy metals and its relation to dietary heavy metal intake. The heavy metals levels in blood not only reflect the dietary exposure but also reflect the redistribution and removal from internal organs/tissues (Berglund Citation2018).
Feather as indicator
Feathers have been extensively used in the ecotoxicological studies as a non-invasive source of biomonitoring. Heavy metals can accumulate in the feather from exogenous sources i.e. surrounding environment as well as from endogenous sources i.e. detoxification of these contaminants through feathers during their growth period. During the growth period, feathers are connected with blood vessels, and the heavy metals ingested with feed are released into bloodstream that became part of keratin structures of feathers (Squadrone et al. Citation2019). Therefore, the heavy metals concentrations of feathers represent both the internal and external exposure. The results of the present study recorded the highest levels of heavy metals in feathers among all samples at selected sites. Abdullah et al. Citation2015 used sediments from contaminated soil to estimate the heavy metals levels in the feathers, excrement and eggs of cattle egrets, which revealed high levels of accumulation than the present study. Another study by Pandiyan et al. (Citation2020) reported toxic metals concentration in three avian species of which Painted Stork was resident of the selected site and Curlew Sandpiper and Eurasian Curlew were migratory species. Analysis revealed that the selected site was highly contaminated (soil, water and benthic prey species), and higher metals levels in feathers were recorded in the local species than the migratory species. Therefore, the feathers could be used as an indicator of environmental pollution of a specific location by its resident species as recorded from the present study or they can be used to monitor environmental pollutants on a larger scale by migratory species as they represent the long-term contamination levels, reflecting the accumulation during the entire growth period of feathers (Borghesi et al. Citation2017).
Conclusion
The results of the present study recorded significant levels of toxic heavy metals at the selected wildlife parks. A high variation of concentration levels of heavy metals among wildlife parks and between samples was recorded. Highest heavy metals concentrations were recorded in feather samples reflecting both the endogenous and exogenous exposure, and Jallo Wildlife Park was the most contaminated site. Linear model of soil with feathers and blood with feed and water revealed the exposure, and an increase in heavy metal contents in bird’s body depends on its surrounding and feed. The present study showed that blood and feathers could be used as reliable bioindicators for environmental contamination of a specific location. Blood can be used to estimate the current exposure to contamination, while feathers can represent long-term, exogenous and endogenous contamination levels. As the selected sites were in different climatic zones, further evaluation of climatic conditions like temperature, physiochemical factors and air quality is required to investigate the accumulation patterns and adverse health effects on animals in captivity or residing in a polluted area.
Ethical approval
The Committee on Animal Rights and Welfare, GC University Faisalabad, Pakistan approved this study (DZ/125/2019).
Consent to participate
All authors are agreed to submit the article to this journal.
Consent to publish
All authors are agreed to publish in this journal.
Authors contributions
Sajida Arooj: research methodology, sampling; Shabana Naz: conceptualization; Aniqa Qaisar: sampling and analysis; Sania Satti: sampling and analysis; Alina Razzaq: methodology; Mehwish Durrani: editing and reviewing; and Rifat Ullah Khan: editing and revising.
Acknowledgements
We extend our appreciation to the Researchers Supporting Project (No. RSP2023R218), King Saud University, Riyadh, Saudi Arabia
Disclosure statement
No potential conflict of interest was reported by the author(s).
Data availability statement
On request.
References
- Abdullah M, Fasola M, Muhammad A, Malik SA, Bostan N, Bokhari H, Kamran MA, Shafqat MN, Alamdar A, Khan M, et al. 2015. Avian feathers as a non-destructive bio-monitoring tool of trace metals signatures: a case study from severely contaminated areas. Chemosphere. 119:553–561. doi:10.1016/j.chemosphere.2014.06.068.
- Akan JC, Sodipo OA, Liman y, Chellube ZM 2014. Determination of heavy metals in blood, urine and water samples by inductively coupled plasma atomic emission spectrophotometer and fluoride using Ion-selective electrode. J Anal Bioanal Tech. 5(9):1–7.
- Ali H, Khan E. 2019. Trophic transfer, bioaccumulation, and biomagnification of non-essential hazardous heavy metals and metalloids in food chains/webs—concepts and implications for wildlife and human health. Hum Ecol Risk Assess An Int J. 25:1353–1376. doi:10.1080/10807039.2018.1469398.
- Ali M, Siddique I, Abbas S. 2022. Characterizing air pollution and its association with emission sources in Lahore: a guide to adaptation action plans to control pollution and smog. Appl Sci. 12(10):5102. doi: 10.3390/app12105102
- Ali Z, Khan K, Abbas S. 2016. Physio-chemical and biological physiognomies of drinking water and its impact on human health, Murree-foothills Himalayas. Pakistan Sci Int (lahore). 28(6):5263–5266.
- Anbazhagan V, Partheeban EC, Arumugam G, et al. 2021. Avian feathers as a biomonitoring tool to assess heavy metal pollution in a wildlife and bird sanctuary from a tropical coastal ecosystem. Environ Sci Pollut Res. 28:38263–38273. doi:10.1007/s11356-021-13371-1.
- Ansara-Ross TM, Ross MJ, Wepener V. 2013. The use of feathers in monitoring bioaccumulation of metals and metalloids in the South African endangered African grass-owl (Tyto capensis). Ecotoxicology. 22:1072–1083. doi:10.1007/s10646-013-1095-4.
- Arooj S, Naz S, Jabeen F, Sultana T. 2023. Biomonitoring of heavy metals and their association with DNA damage in Indian peafowl (Pavo cristatus) under captivity. Environ Sci Pollut Res. 30(13):38306–38318. doi: 10.1007/s11356-022-24898-2
- Berglund ÅMM. 2018. Evaluating blood and excrement as bioindicators for metal accumulation in birds. Environ Pollut. 233:1198–1206. doi:10.1016/j.envpol.2017.10.031.
- Biaza. 2006. Zoo research guidelines. Statistics (Ber). 1–46.
- Binkowski ŁJ, Sawicka-Kapusta K. 2015. Lead poisoning and its in vivo biomarkers in Mallard and Coot from two hunting activity areas in Poland. Chemosphere. 127:101–108. doi:10.1016/j.chemosphere.2015.01.003.
- Borghesi F, Dinelli E, Migani F, et al. 2017. Assessing environmental pollution in birds: a new methodological approach for interpreting bioaccumulation of trace elements in feather shafts using geochemical sediment data. Methods Ecol Evol. 8:96–108. doi:10.1111/2041-210X.12644.
- Bukar H, Sa MD. 2014. Determination of some heavy metals in selected poultry feeds available in Kano Metropolis, Nigeria. ChemSearch J. 5:8–11.
- Carvalho PC, Bugoni L, Mcgill RAR, Bianchini A. 2013. Metal and selenium concentrations in blood and feathers of petrels of the genus procellaria. Environ Toxicol Chem. 32:1641–1648. doi:10.1002/etc.2204.
- DeForest DK, Brix KV, Adams WJ. 2007. Assessing metal bioaccumulation in aquatic environments: the inverse relationship between bioaccumulation factors, trophic transfer factors and exposure concentration. Aquat Toxicol. 84:236–246. doi:10.1016/j.aquatox.2007.02.022.
- Falkowska L, Szumiło E, Hajdryh J, et al. 2013. Effect of diet on the capacity to remove mercury from the body of a penguin (Spheniscus demersus) living in the ZOO. E3S Web Conf. 1:2–4. doi:10.1051/e3sconf/20130112002.
- Furtado R, Pereira ME, Granadeiro JP, Catry P. 2019. Body feather mercury and arsenic concentrations in five species of seabirds from the Falkland Islands. Mar Pollut Bull. 149:110574. doi:10.1016/j.marpolbul.2019.110574.
- Gupta V. 2013. Feces of captive wild mammal use as bio-indicator of heavy metal pollution in urban air. Int J Innov Res Sci Eng Technol. 2:2404–2411.
- Jothi JS, Yeasmin N, Anka IZ, Hashem S. 2017. Chromium and lead contamination in commercial poultry feeds of Bangladesh. Int J Agric Res Innov Technol. 6:57–60. doi:10.3329/ijarit.v6i2.31705.
- Lucia M, Bocher P, Cosson RP, et al. 2012. Evidence of species-specific detoxification processes for trace elements in shorebirds. Ecotoxicology. 21:2349–2362. doi:10.1007/s10646-012-0991-3.
- Markert B. 2007. Definitions and principles for bioindication and biomonitoring of trace metals in the environment. J Trace Elem Med Biol. 21:77–82. doi:10.1016/j.jtemb.2007.09.015.
- Markowski M, Kaliński A, Skwarska J, et al. 2013. Avian feathers as bioindicators of the exposure to heavy metal contamination of food. Bull Environ Contam Toxicol. 91:302–305. doi:10.1007/s00128-013-1065-9.
- Migani F, Borghesi F, Dinelli E. 2015. Geochemical characterization of surface sediments from the northern adriatic wetlands around the Po river delta. Part I: bulk composition and relation to local background. J Geochemical Explor. 156:72–88. doi:10.1016/j.gexplo.2015.05.003.
- Movalli PA. 2000. Heavy metal and other residues in feathers of laggar falcon Falco biarmicus jugger from six districts of Pakistan. Environ Pollut. 109:267–275. doi:10.1016/S0269-7491(99)00258-4.
- Muhammad S, Ullah S, Ali W, et al. 2022. Spatial distribution of heavy metal and risk indices of water and sediments in the Kunhar river and its tributaries. Geocarto Int. 37:5985–6003. doi:10.1080/10106049.2021.1926557.
- Murtaza G, Usman M. 2022. Desalination and Water Treatment Assessment of various heavy metals level in groundwater and soil at tannery manufacturing areas of three mega cities (Sialkot, Lahore and Karachi) of Pakistan. Desalin Water Treat. 266:121–130. doi:10.5004/dwt.2022.28640.
- Naz S, Arooj S, Ali Z, Farooq Z. 2021. Potential consequences of captivity and environmental pollution in endoparasitic prevalence in different antelopes kept at wildlife parks. Environ Sci Pollut Res Int. 28:16308–16313. doi:10.1007/s11356-020-11561-x.
- Naz S, Muazzam S, Sagheer A, Tanveer A, Khan NA, Ali Z, Chand N, Khan RU. 2020. Captivity stress influences the DNA damage of Pavo cristatus under environmental conditions of Faisalabad, Pakistan. Environmental Science and Pollution Research. 27(5):5636–5639. http://dx.doi.org/10.1007/s11356-019-07307-z.
- Nighat S, Iqbal S, Nadeem MS, et al. 2013. Estimation of heavy metal residues from the feathers of Falconidae, Accipitridae, and Strigidae in Punjab, Pakistan. Turkish J Zool. 37:488–500. doi:10.3906/zoo-1112-1.
- Pandiyan J, Mahboob S, Jagadheesan R, et al. 2020. A novel approach to assess the heavy metal content in the feathers of shorebirds: A perspective of environmental research. J King Saud Univ - Sci. 32:3065–3071. doi:10.1016/j.jksus.2020.08.014.
- Parmar TK, Rawtani D, Agrawal YK. 2016. Bioindicators: the natural indicator of environmental pollution. Front Life Sci. 9:110–118. doi:10.1080/21553769.2016.1162753.
- Rutkowska M, Płotka-Wasylka J, Lubinska-Szczygeł M, et al. 2018. Birds’ feathers – suitable samples for determination of environmental pollutants. TrAC - Trends Anal Chem. 109:97–115. doi:10.1016/j.trac.2018.09.022.
- Salim Z, Khan MU, Malik RN. 2020. Concentration, distribution and association of heavy metals in multi-matrix samples of Himalayan foothill along elevation gradients. Environ Earth Sci. 79:479. doi:10.1007/s12665-020-09218-6.
- Sanderfoot OV, Holloway T. 2017. Air pollution impacts on avian species via inhalation exposure and associated outcomes. Environ Res Lett. 12. doi:10.1088/1748-9326/aa8051.
- Shehzad A, Anjum KM, Yaqub A, et al. 2022. Estimation of metal content in avian fauna of Sialkot, Pakistan using feathers as bio-indicator. J Anim Plant Sci. 32:1460–1468. doi:10.36899/JAPS.2022.5.0552.
- Siddig AAH, Ellison AM, Ochs A, et al. 2016. How do ecologists select and use indicator species to monitor ecological change? Insights from 14 years of publication in ecological indicators. Ecol Indic. 60:223–230. doi:10.1016/j.ecolind.2015.06.036.
- Soliman MM, Hesselberg T, Mohamed AA, Renault D. 2022. Trophic transfer of heavy metals along a pollution gradient in a terrestrial agro-industrial food web. Geoderma. 413. doi:10.1016/j.geoderma.2022.115748.
- Squadrone S, Abete MC, Brizio P, et al. 2018. Metals in feathers of African penguins (Spheniscus demersus): considerations for the welfare and management of seabirds under human care. Bull Environ Contam Toxicol. 100:465–471. doi:10.1007/s00128-018-2293-9.
- Squadrone S, Brizio P, Favaro L, et al. 2019. Humboldt penguins’ feathers as bioindicators of metal exposure. Sci Total Environ. 650:1734–1739. doi:10.1016/j.scitotenv.2018.09.326.
- Ukpe RA, Chokor AA. 2018. Correlation between concentrations of some heavy metal in poultry feed and waste. Open Access Journal of Toxicology. 3(2):555609. doi: 10.19080/OAJT.2018.03.555609
- Woods JM, Eyer A, Miller LJ. 2022. Bird welfare in zoos and aquariums: general insights across industries. J Zool Bot Gard. 3:198–222. doi:10.3390/jzbg3020017.
- Yasmeen R, Shaheen S, Khan BN, et al. 2020. Faecal matter of spotted deer (Axis axis) acts as bioindicator of heavy metals contamination in the air. Pak J Zool. 52:813–816. doi:10.17582/journal.pjz/20181214041244.