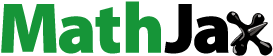
ABSTRACT
This study explored the growth parameters and nutritional composition of Black soldier fly larvae (BSFL) reared on some selected alternative organic waste materials. The substrates comprised wheat bran (WB) as the control, millet waste (MW), restaurant leftovers (RL) and fruits waste (FW) and were replicated 8 times in a mini-insectary set-up. Proximate nutrient and mineral composition of the larvae were analyzed. RL had the highest (p < 0.0001) total weight of larvae, number of larvae, efficiency of conversion and substrate reduction index compared to the other substrates. Crude protein (%) content of the BSFL was superior (p < 0.000) in the WB and MW substrate groups at 41.1% and 41.4%, respectively. Crude protein and dry matter yield (g) of the BSFL was higher (p < 0.0001) in the RL than in the other substrates. Metabolizable energy and dry matter were superior (p < 0.0001) in MW and RL groups respectively to all the other substrate groups. RL recorded the lowest (p < 0.0001) phosphorus and calcium levels relative to the other substrates. Conclusively, the crude protein content of the BSFL irrespective of the substrates was adequate to be employed in the livestock feed industry.
Introduction
The global demand for meat from 2005/07 to –2050 will increase by 76%. However, this increased demand in this period will be mainly from developing countries (113%) and less from developed countries (27%) (Alexandratos and Bruinsma Citation2012; Van Huis and Dunkel Citation2017; Greenhalgh et al. Citation2020). In the light of global climate change which possess a significant threat to food crop production, animal production stands tall as the anchor of future food security, but it is also bedeviled by a scope of challenges including the ever-soaring cost of production (Gomez-Zavaglia et al. Citation2020). The situation as described is because the animal production industry heavily relies on the production of staple food crops like grains which at the same time is threatened by climate change, as well as the scarce or limited protein sources which augment the grains to help arrive at a nutritionally balanced diet for the animals.
Over decades, scientists have been focused on finding alternative protein sources suitable for livestock production, since conventional protein ingredients over the years are becoming less available and more expensive, thereby skyrocketing the cost of production of livestock in general (Makinde and Makinde Citation2015; Salter and Lopez-Viso Citation2021). Even though energy-supplying ingredients in the feed composition claim the highest percentage of inclusion, their cost, alternatives and availability remain comparatively cheaper, diversified and available than the protein sources (Agyekum and Nyachoti Citation2017). Notable amongst the conventional protein sources/ingredients used in animal feed production include those of plant origin (Soybean meal, palm kernel cake, seeds and the like) and those of animal origin (Fish meal, meat and bone meal, blood meal, slaughter waste etc). Owing to the diversity in the amino acid composition and quality of the notable protein ingredients, it is almost impossible for the animal nutritionist to resort to a single protein ingredient to meet the nutritional requirement of the category of animals for which the feed is to be prepared (MacArthur et al. Citation2021; Ma et al. Citation2022). Hence, nutritionists adopt a combination of various natural and synthetic sources of protein to arrive at a balanced composition for the animals.
To encourage the growth of the animal production industry, scientists have identified and proposed a myriad of alternative protein sources capable of helping us address the challenges associated with protein sources in animal feed production (Shah and Muir Citation2014; Montesano et al. Citation2020). Some of these proposed alternatives include agro-industrial by-products (AIBPs) such as seed cakes, slaughterhouse waste, insects and insect larvae, and other organic and synthetic materials through scientific experiments have either proven viable or less useful (Pomalégni et al. Citation2017; Schreven et al. Citation2021; Isibika et al. Citation2023). Nonetheless, there is still more to be scientifically established as useful and fit for purpose. One of the proposed alternatives which are fast gaining attention globally is that of insects and insect larvae.
Black Soldier Fly (Hermetia illucens) larvae production is gaining popularity as a sustainable alternative to traditional animal feed sources (Rodrigues et al. Citation2022; Makokha et al. Citation2023). They are a significant source of protein and fat, making them a good alternative for animal feed, particularly for aquaculture and poultry production (Rodrigues et al. Citation2022). Furthermore, the larvae have been demonstrated to be successful in converting some selected organic waste into high-quality protein, making them an environmentally friendly waste management alternative depending on the region of the world and its regulations (Amrul et al. Citation2022; Salam et al. Citation2022). For instance, it is legal to convert waste into protein for use as aquaculture and poultry feed in the United States (AAFCO Citation2016), the European Union and other regions of the world (Cutrignelli et al. Citation2018; Pang et al. Citation2020; Alagappan et al. Citation2022). This is especially important in developing countries where waste management is a major concern.
Additionally, black soldier fly larvae can be reared on a variety of organic materials, including agricultural and food waste, such as fruit and vegetable waste, brewery waste, and manure (Scala et al. Citation2020; Scieuzo et al. Citation2022). The larvae can transform these waste items into high-quality protein, making them a great solution for waste management and sustainable animal feed production (Salam et al. Citation2022). It is hypothesized that employing organic waste as a substrate for black soldier fly larvae will result in high larvae yields and protein content, providing a sustainable and eco-friendly option for waste management and animal feed production. Thus, the purpose of this research was to explore the feasibility of using alternative organic waste as a substrate for the continuous production of black soldier fly larvae for small-scale farmers. The study aimed to evaluate the impact of substrate composition on larval yield, proximate and mineral composition, as well as the possibility for waste reduction and resource recovery.
Materials and methods
Study location and duration
A mini insectary was set up at the Department of Animal Science, Faculty of Agriculture of the Kwame Nkrumah University of Science and Technology (KNUST), Kumasi to undertake the production experiment of the black soldier fly larvae. As much as possible, the research resorted to the use of locally available inputs and resources with the best technological improvisation to undertake the production, harvesting and processing of the black soldier fly larvae. This was to ensure the easy replication of the processes by any local/rural smallholder farmer with the interest of doing so and also to ensure the least cost of production. The experiment lasted for six (6) weeks.
Source (s) of black soldier fly larvae (BSFL)
The black soldier fly pupae were obtained from a BSF production and processing facility at the Animal Research Institute in Accra, Ghana. The colony was kept under constant conditions in a climate-controlled environment at 28°C, 60% relative humidity, and a photoperiod of 14L: D10 cycles. They were then kept under these favourable conditions to hatch into flies. The flies were then well managed to produce eggs which were carefully collected to initiate and sustain the cycle of larvae production in the insectary.
Experimental phases
Phase 1: Protocols for setting up the BSF colony/insectary
A 2 m x 2 m square fibre net cage with a zip opening was created to house the flies that emerged from the pupae obtained from the Animal Research Institute in Accra. To provide the flies access to sunshine and fresh air, the net cage was stretched outside beneath a shed. In order to provide the flies with access to water, a knapsack sprayer was regularly used (3–4 times per day) to spray fresh water into the net cage. Two days after fly emergence from the pupal case, an attractant made from fermented brewers’ spent grains was used to stimulate egg laying following mating. Special wooden pallets stacked together with a rubber band were placed on the attractant to collect the eggs. Eggs were collected every 48 h and weighed. They were then kept in small plastic bowls with a tissue lining for three days, after which they were inoculated into the hatching substrate which is a moist mixture (moisture of 60%) of wheat bran and water. The set-up was left for seven days during which the eggs will hatch. Hatchlings fall on the substrate and begin to feed immediately. After seven days, the one-week-old hatchlings were separated from the standard substrate and weighed and distributed on the various experimental substrates.
Phase 2: Substrates and procedure
A total of four (4) substrates were used in the larvae production phase of the experiment. One kilogram (1 kg) of each substrate was weighed into the bowls used for the rearing of the larvae and inoculated 10 g (600-620 larvae/g) of the one-week-old hatchlings of the black soldier fly larvae. The content of the bowls was subsequently topped up with a known amount of the same substrate according to the rate of waste reduction by the growing larvae in the substrate. The substrates comprised wheat bran, WB (control), millet waste (MW), restaurant leftover (RL) and fruits waste (FW) (). Each experimental substrate had eight (8) replicated bowls of the set up. Feeding of the hatchlings lasted 7 days and harvesting was done on day 15. Substrates in which the performance of the larvae was seen as poor were left for an additional seven days to allow the larvae enough time to feed. Among the parameters measured was the total weight of larvae, survival rate, substrate reduction index and waste reduction index as calculated per the formulae:
Figure 1. Organic wastes used as substrates. (a) WB – wheat bran. (b) MW – millet waste. (c) RL – restaurant leftovers. (d) FW – fruit waste.
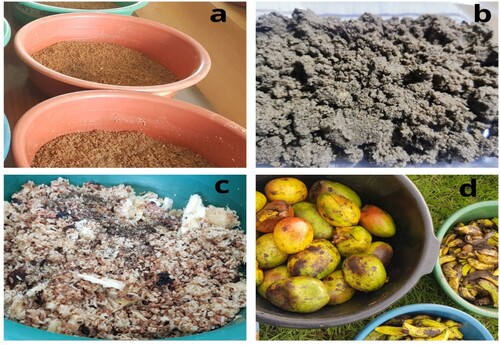
Total weight of larvae (TW), (Leong et al. Citation2016) readapted for this research substituting prepupal body weight (g) with larva body weight (g)
(1)
(1)
Substrate reduction Index (SRi) (Tschirner and Simon Citation2015)
(2)
(2)
(3)
(3) Where, B = total larval + pupal biomass (g); W = total amount of substrate provided; R = residual of the substrate
Survival rate of larvae (SR)
(4)
(4)
Phase 3: Harvesting and processing
Manually, the larvae were separated from the substrate by vigorously shaking the content of the experimental bowl in a 2 mm wire meshed container. After the separation, the samples of the resulting larvae were kept for 24 h to allow for gut emptying by the larvae and then oven dried at a temperature of 60°C for 48 h.
Product quality
The proximate composition and mineral composition of the dried larvae were estimated based on the pooled samples from replicates of each treatment in the laboratory.
Proximate analyses
According to the methods of Greenfield and Southgate (Citation2003), the analytical determinations of water (moisture), dry matter, crude protein, crude fat (ether extract), crude ash, and crude fibre constituted the proximate analysis. Differences after freeze-drying were used to calculate moisture content. The amount of dry matter was determined using the weight differential before and after the sample had been dried for 24 h at 100°C in the oven. By burning samples at 550°C for 6 h in a combustion oven, crude ash was ascertained. Using the nitrogen-to-protein conversion factor of 6.25, the crude protein concentration was calculated using the method of Dumas-Gaudot et al. (Citation2002). After diethyl ether extraction, ether extract, a gauge of crude fat, was evaluated gravimetrically with a Soxhlet apparatus. Using the Driemeyer (Citation2016) approach, the crude fibre content was calculated. The Megazyme total dietary fibre test technique k-tdfr 05/12.27 was employed to determine the content of soluble. These analyses were carried out twice and followed the AOAC (Citation2012) reported methodology. These formulae were employed:
(5)
(5) Where:
A = weight of dry and empty crucible (g)
B = weight of air-dried test sample (g)
C = weight of crucible and moisture-free test sample (g)
(6)
(6)
(7)
(7)
(8)
(8)
(9)
(9)
(10)
(10)
(11)
(11)
Total weight of nutrients (TWN) was also determined using the formula:
(12)
(12) Where:
A = mass of residue in crucible after drying (g)
B = mass of residue in crucible after ashing (g)
NFE = Nitrogen-free-extract.
ME = Metabolizable energy.
Mineral composition
Using inductively coupled plasma optical emission spectrometry (ICP-OES), the mineral composition of the BSFL was identified (British Standard Institution Citation2001). The materials were digested in diluted nitric acid (7 mol/L) (British Standard Institution Citation2001) after being burned till the ash grey turned red-brown. Aqua regia was used to remove iron independently (International Organization for Standardization Citation2002).
Statistical analysis
Analysis of variance was conducted on the data collected using the procedure of the General Linear Model of Minitab, Version 19 (Minitab, LLC, NY, US, 2019) following the model: yij = μ + τj + ϵij, where μ = general mean, τ = treatment effect and ϵ = random error. All comparisons between means were tested using the Tukey method at a p < 0.05 significance level of acceptance.
Results
Black soldier fly larvae (BSFL) production
The results of the BSFL production performance on the various substrates are presented in . The total weight of the larvae per day fed on the restaurant leftovers (RL) was significantly superior (p < 0.0001) compared to the other substrates (). The wheat bran and fruit waste produced the highest (p < 0.0001) individual larval weight compared to the other substrates (). Although RL had the maximum total weight of the larvae, the individual larval weight was light. In , the total nutrient weight of the larvae reared on the different substrates is presented. The weight of ash was superior (p < 0.0001) in the restaurant leftovers (RL) relative to the other substrates. Crude protein weight was significantly higher (p < 0.0001) in the restaurant leftovers (RL) than in the wheat bran and fruit waste substrates. Again, the restaurant leftover substrate had an increased (p < 0.0001) dry matter and nitrogen-free extract weight compared to the other substrates. The fat weight of the larvae was higher (p < 0.0001) in the millet waste (MW) than in the wheat bran and fruit waste substrates. As for the crude fibre weight of the larvae, the millet waste (MW) was superior (P = 0.002) compared to the other substrates. Similarly, the efficiency of conversion of the ingested food was higher (p < 0.0001) in the BSFL group that was fed restaurant leftovers than that in the other groups (). Following a similar trend, the BSFL in the restaurant leftovers substrate had a significantly higher (p = 0.001) substrate reduction index and was more efficient in utilizing these substrates as compared to those in the other substrates (). The number of larvae harvested from the substrates was significantly higher (p < 0.0001) in the restaurant leftovers group than in the other substrate groups () but the fruit waste substrate produced more larvae (p = 0.023) than the wheat bran substrate. Additionally, the survival rate of the larvae in the restaurant leftover substrate was superior (p < 0.0001) to the larvae in the other substrates but fruit waste larvae survived better (p = 0.023) than the wheat bran larvae ().
Figure 2. Total weight of BSFL reared on different substrates. Different alphabets indicate significance at p < 0.05. WB – wheat bran. MW – millet waste. RL – restaurant leftovers. FW – fruit waste
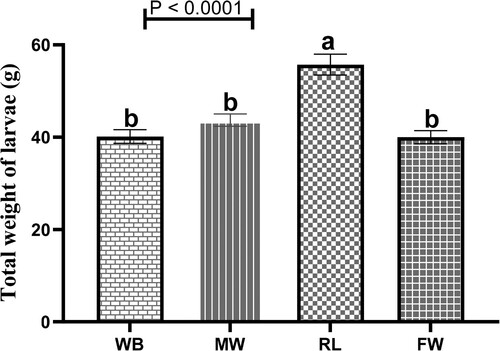
Figure 3. Individual larval weight reared on different substrates. Different alphabets indicate significance at p < 0.05. WB – wheat bran. MW – millet waste. RL – restaurant leftovers. FW – fruit waste.
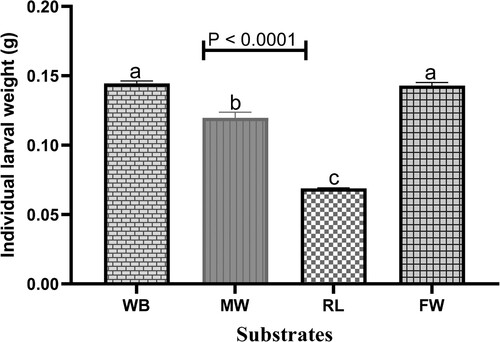
Figure 4. Total nutrient weight of BSFL reared on different substrates. Different alphabets indicate significance at p < 0.05. WB – wheat bran. MW – millet waste. RL – restaurant leftovers. FW – fruit waste.
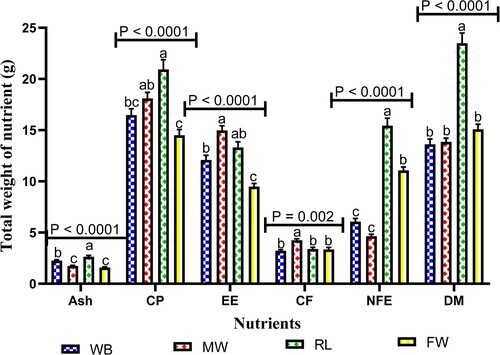
Figure 5. Efficiency of conversion of BSFL reared on different substrates. Different alphabets indicate significance at p < 0.05. WB – wheat bran. MW – millet waste. RL – restaurant leftovers. FW – fruit waste.
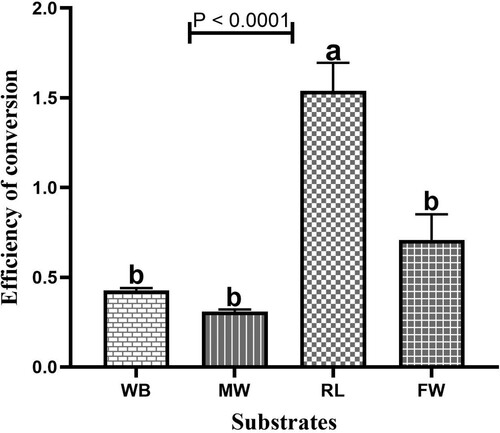
Figure 6. Substrate reduction index of BSFL reared on different substrates. Different alphabets indicate significance at p < 0.05. WB – wheat bran. MW – millet waste. RL – restaurant leftovers. FW – fruit waste.
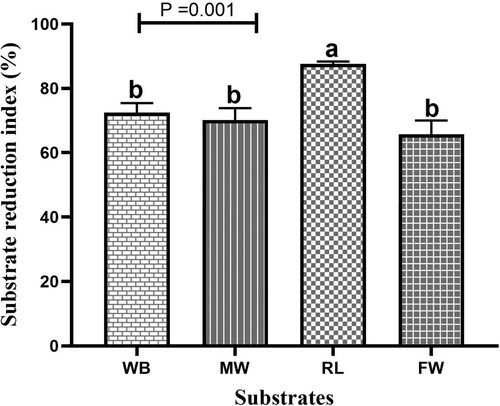
Proximate chemical composition of BSFL
The chemical composition of the BSFL reared on different substrates is shown in . The crude protein content of the larvae was significantly higher (p = 0.005) in the wheat bran and millet waste substrate groups compared to the fruit waste substrate group. The larvae reared on the millet waste and fruit waste substrates had an increased (p < 0.0001) crude fibre content compared to that reared on the restaurant leftovers substrate. The fat and metabolizable energy followed a similar trend. The fat and energy content of the larvae reared on the millet waste substrate was statistically higher (p < 0.0001) than those reared in the other substrates but the larvae harvested from the restaurant leftovers and fruits waste substrates had similar (p > 0.05) fat and energy contents. The ash content of the larvae harvested from the wheat bran substrate was significantly superior (p < 0.0001) compared to that harvested from the other substrates. The nitrogen-free extract was higher (p < 0.0001) in the larvae harvested from the restaurant leftovers and fruit waste substrates than that harvested from the other substrates. The moisture and dry matter contents of the larvae harvested from the substrates groups were significantly different (p < 0.0001) from each other with millet waste and restaurant leftovers obtaining the highest moisture and dry matter contents, respectively.
Table 1. Proximate composition of Black soldier fly larvae reared on different substrates.
Mineral composition of BSFL
The mineral composition of BSFL harvested from the various substrates is presented in . The potassium, magnesium, iron and zinc content of the larvae harvested from the wheat bran substrate were higher (p < 0.0001) than that obtained in the other substrates. The phosphorus content of the larvae harvested from the substrates groups was significantly different (p < 0.0001) from each other with fruit waste obtaining the highest phosphorus content. The calcium content of the larvae harvested from the substrates groups was significantly different (p < 0.0001) from each other with millet waste obtaining the highest calcium content. The larvae harvested from the restaurant's leftovers substrate recorded the lowest content (p < 0.0001) of manganese. A higher level (p < 0.0001) of copper was recorded in the larvae harvested from the wheat bran and restaurant leftover substrates than those harvested from the other substrates. However, total nitrogen did not differ (P > 0.05) across all the substrate groups.
Table 2. Mineral composition of Black soldier fly larvae reared on different substrates.
Discussion
The livestock industry today is challenged by the increasing cost of feed protein coupled with the unsustainable approach of increasing the production of soybean due to land deforestation (Dortmans et al. Citation2017). Insect protein seems to be a leading way to augment industry players’ efforts to find alternatives to conventional protein sources for animal production. The black soldier fly larvae present a nutritional profile that can replicate that of plant protein for livestock production. Thus, this study highlights the larvae production and proximate chemical and mineral composition of BSFL reared on different organic wastes for subsistent small-scale farming. The total weight of the larvae reared on the restaurant leftovers was superior to those reared on the other substrates with better efficiency of conversion of food ingested. This implies that in this present study, the larvae reared on the restaurant's leftover substrate were able to convert a greater proportion of the energy in the food waste into insect biomass (Scala et al. Citation2020). It can also be inferred that the quality of the restaurant leftovers was better than the other forms of waste since the quality of feed affects its efficiency of utilization (Reynolds et al. Citation2019). The quality of the feed was reflected in the productivity of the BSFL as more larvae were harvested from the restaurant's leftover substrate affirming the survival rate which was better than the other substrates. In this present study, the total yield of crude protein and dry matter was greater for the larvae reared on the restaurant's leftover substrate. Comparably, it is suggested that the food waste from the restaurant has a better nutrient composition than millet waste and fruit waste. Hence, small-scale farmers can use food waste from restaurants as a substrate for BSFL to increase yield and productivity. The substrate reduction index was higher for the restaurant's leftover substrate relative to the other substrates. This is because the larvae reared on the restaurant leftovers used higher proportions of the feed for growth and productivity, unlike the others that required extra feed for an extended period to reach maturity due to poor growth rate and efficiency of conversion of ingested food and thereby increasing the byproducts. A high substrate reduction index is associated with the insect's ability to make maximum use of the substrate for growth and proliferation (Surendra et al. Citation2020; Seyedalmoosavi et al. Citation2022). It is established that larvae or pupae reared on food wastes have a high substrate reduction index compared to wastes with much fibre composition (Bava et al. Citation2019; Meneguz et al. Citation2018). However, the present study revealed that rearing BSFL on restaurant leftovers yielded lighter individual larval weight, which suggests that there is a need to find nutritional interventions that can enrich the composition of the substrates for an enhanced larval mass. The supplementation of waste material with additives improves its nutrient availability and efficiency in supporting BSFL growth (Pamintuan et al. Citation2020; Cohn et al. Citation2022). It was indicated that BSFL that was reared on duck manure and a mixture of duck manure and rice straw improved individual larval weight, the efficiency of conversion and survival rate (Pamintuan et al. Citation2020). Heussler et al. (Citation2023) observed that wheat bran pre-treated with 0.15% potassium sorbate increased larval and pupae biomass yield compared to the control (wheat bran). Furthermore, Yakti et al. (Citation2023) reported a superior larval growth rate when chicken feed was supplemented with cellulose compared to chicken feed with either straw or lignin.
The crude protein content in the BSFL from various substrates generally mirrors the nutritional content of the substrates. The analyzed crude protein content of the BSFL reared on the substrates ranged from 36.2 to 41.1%, which is slightly superior to that (33–41%) reported by Shumo et al. (Citation2019) but within the range (32.97–48.93%) reported by Adebayo et al. (Citation2021). This comparison is possible because similar methods and substrates were tested in these studies with slight modifications. The values obtained are satisfactory to endorse the use of BSFL as an alternative source of protein in the animal industry and can partly or fully replace fishmeal and soybean meal in conventional feed for livestock (Liu et al. Citation2008; Bosch et al. Citation2019). The discrepancies in the protein levels of the BSFL suggest that one can selectively choose substrates (diet) that will yield the required biomass and nutrient content for specific animals (St-Hilaire et al. Citation2007). The fat content of the present study for food remains and fruit waste was low (34.26 and 34.05, respectively), however, higher fibre amounts were recorded in this present study relative to that reported by Adebayo et al. (Citation2021). The high energy levels of the BSFL reared on these substrates suggests that the larvae are efficient at converting low-nutrient substrates into appreciable biomass with adequate nutrients. High fibre content of substrate and moisture content have been listed as variables that can affect larval survivability and mineral composition of the larvae (Yakti et al. Citation2023). Palma et al. (Citation2018) evaluated the impact of almond hulls and shells on the growth of BSFL with an emphasis on aeration and moisture content and indicated that low levels of oxygen and moisture positively improved larval growth and survival.
Appreciating the impact of minerals as they play essential roles in the physiological function of animals is quite revealing because they can affect the overall quality of animal production. The Ca:P ratio of the BSFL in the present study was quite low compared to that of fish species which has a recommended ratio of 1:2 and 1:5:1 and this may lead to deficiencies (Tschirner and Simon Citation2015). This means that BSFL meal might not be able to match the superior content of Phosphorus available in fishmeal (18 g/kg) (Tschirner and Simon Citation2015). Since calcium makes up more than 90% of the mineral matrix, it plays a critical function in the development of bone and eggs in layer chickens. Therefore, bone loss, slowed growth and incorrect posture can be caused by calcium and phosphorus deficiency (Hafeez et al. Citation2015). Notwithstanding, concerns can arise from high calcium content in feed formulations for young animals, such as piglets because it may cause the pH of the stomach to rise, which might lead to bacterial infection (Lawlor et al. Citation2005). The level of calcium in this present study is very low compared to that of Shumo et al. (Citation2019) and Adebayo et al. (Citation2021) who found a range of 1.7–3.2% and 5.21–12.85%, respectively. Considering the other minerals, the control substrate (WB) appears to have higher levels compared to the other substrates. This suggests that the impact of the substrate used to rear this prepupae could have certain drawbacks, which could account for the variation in the range of values of the mineral content of the BSF larvae. Additionally, the mineral content of the black soldier fly larvae may increase if fat is removed from them. It was reported that 17% chicken feed mixed with straw of different particle sizes influenced the mineral composition of the larvae such that there were differences in the outcome of 26.78%, 12.04% and 35.34% for calcium, magnesium and phosphorus concentrations, respectively (Yakti et al. Citation2023).
Conclusion
Black soldier fly larvae can be used to produce high-quality protein from organic waste streams, lessen pollution, and supplement costly sources of protein in animal feeds. Black soldier fly larval feeding is influenced by the substrate being utilized for rearing. Regardless of the substrate type provided, the crude protein level is appreciable, making it a suitable resource for the animal feed industry due to its biomass, reproductive potential, and nutritional profile. Thus, it is imperative to assess the nutritional qualities of BSFL reared on various types of industrial wastes, with a particular emphasis on the assessment of the fatty acid and amino acid content to produce economically viable inclusion levels in animal feeds and build a database of substrates that are suitable for the production of BSFL.
Acknowledgements
The Authors acknowledge the contribution and assistance of Animal Research Institute (ARI) and the Department of Animal Science, KNUST.
Disclosure statement
No potential conflict of interest was reported by the author(s).
Data availability statement
All data used is available and can be provided upon reasonable request.
References
- Adebayo HA, Kemabonta KA, Ogbogu SS, Elechi MC, Obe MT. 2021. Comparative assessment of developmental parameters, proximate analysis and mineral compositions of black soldier fly (Hermetia illucens) prepupae reared on organic waste substrates. Int J Trop Insect Sci. 41:1953–1959. doi:10.1007/s42690-020-00404-4.
- Agyekum AK, Nyachoti CM. 2017. Nutritional and metabolic consequences of feeding high-fiber diets to swine: a review. Engineering. 3(5):716–725. doi:10.1016/J.ENG.2017.03.010.
- Alagappan S, Rowland D, Barwell R, Mantilla SMO, Mikkelsen D, James P, Yarger O, Hoffman LC. 2022. Legislative landscape of black soldier fly (Hermetia illucens) as feed. J Insects Food Feed. 8(4):343–355. doi:10.3920/JIFF2021.0111.
- Alexandratos N, Bruinsma J. 2012. World agriculture towards 2030/2050. In: The 2012 Revision. ESA Working Paper No. 12-03. Rome: Food and Agriculture Organization of the United Nations.
- Amrul NF, Kabir Ahmad I, Ahmad Basri NE, Suja F, Abdul Jalil NA, Azman NA. 2022. A review of organic waste treatment using black soldier fly (Hermetia illucens). Sustainability. 14(8):4565. doi:10.3390/su14084565.
- Association of American Feed Control Officials. 2016. Model bill and regulations. In: 2016 Official Publication. Ch. 4. Champagne, IL: Association of American Feed Control Officials; p. 155.
- Association of Official Analytical Chemists (AOAC) International. 2012. Official methods of analysis of AOAC international, 17th ed. Arlington: AOAC International; p. 25–42.
- Bava L, Jucker C, Gislon G, Lupi D, Savoldelli S, Zucali M, Colombini S. 2019. Rearing of Hermetia illucens on different organic by-products: Influence on growth, waste reduction, and environmental impact. Animals (Basel). 9(6):289. doi:10.3390/ani9060289.
- Bosch G, van Zanten HH, Zamprogna A, Veenenbos M, Meijer NP, van der Fels-Klerx HJ, van Loon JJA. 2019. Conversion of organic resources by black soldier fly larvae: legislation, efficiency and environmental impact. J Clean Prod. 222:355–363. doi:10.1016/j.jclepro.2019.02.270.
- British Standards Institution (BSI). 2001. EN 13652:2001, Soil improvers and growing media. Extraction of water-soluble nutrients and elements. London: BSI; p. 12–24.
- Cohn Z, Latty T, Abbas A. 2022. Understanding dietary carbohydrates in black soldier fly larvae treatment of organic waste in the circular economy. Waste Manag. 137:9–19. doi:10.1016/j.wasman.2021.10.013.
- Cutrignelli MI, Messina M, Tulli F, Randazzo B, Olivotto I, Gasco L, Loponte R, Bovera F. 2018. Evaluation of an insect meal of the Black Soldier Fly (Hermetia illucens) as soybean substitute: Intestinal morphometry, enzymatic and microbial activity in laying hens. Res Vet Sci. 117:209–215. doi:10.1016/j.rvsc.2017.12.020.
- Dortmans B, Diener S, Bart V, Zurbrügg C. 2017. Black soldier fly biowaste processing: a step-by-step guide. eawag.
- Driemeyer H. 2016. Evaluation of black soldier fly (Hermetia illucens) larvae as an alternative protein source in pig creep diets in relation to production, blood and manure microbiology parameters [dissertation]. University of Stellenbosch.
- Dumas-Gaudot E, Pozo MJ, Cordier C, Gianinazzi S, Barea JM, Azcón-Aguilar C. 2002. Localized versus systemic effect of arbuscular mycorrhizal fungi on defence responses to Phytophthora infection in tomato plants. J Exp Bot. 53(368):525–534. doi:10.1093/jexbot/53.368.525.
- Gomez-Zavaglia A, Mejuto JC, Simal-Gandara J. 2020. Mitigation of emerging implications of climate change on food production systems. Food Res Int. 134:109256. doi:10.1016/j.foodres.2020.109256.
- Greenfield H, Southgate DAT. 2003. Food composition data. In: Production management and use. 2nd ed. Vol. 2. Boston, MA: Springer; p. 17–19.
- Greenhalgh S, Chrystal PV, Selle PH, Liu SY. 2020. Reduced-crude protein diets in chicken-meat production: justification for an imperative. World's Poult Sci J. 76(3):537–548. doi:10.1080/00439339.2020.1789024.
- Hafeez A, Mader A, Ruhnke I, Röhe I, Boroojeni FG, Yousaf MS, Männer K, Zentek J. 2015. Implication of milling methods, thermal treatment, and particle size of feed in layers on mineral digestibility and retention of minerals in egg contents. Poult Sci. 94(2):240–248. doi:10.3382/ps/peu070.
- Heussler CD, Insam H, Walter A, Steiner BS, Steiner FM, Klammsteiner T. 2023. Life-history traits of black soldier fly reared on agro-industrial by-products subjected to three pre-treatments: a pilot-scale study. J Insects Food Feed. 9(5):545–556. doi:10.3920/JIFF2022.0044.
- International Organization for Standardization. 2002. ISO 15587–1: 2002, 2002 Water quality – digestion for the determination of selected elements in water – Part 1: aqua regia digestion. Geneva: ISO; p. 56–72.
- Isibika A, Simha P, Vinnerås B, Zurbrügg C, Kibazohi O, Lalander C. 2023. Food industry waste-an opportunity for black soldier fly larvae protein production in Tanzania. Sci Total Environ. 858:159985. doi:10.1016/j.scitotenv.2022.159985.
- Lawlor P, Lynch P, Caffrey P, et al. 2005. Measurements of the acid binding capacity of ingredients used in pig diets. Ir Vet J. 58:447–452. doi:10.1186/2046-0481-58-8-447.
- Leong SY, Kutty SRM, Malakahmad A, Tan CK. 2016. Feasibility study of biodiesel production using lipids of Hermetia illucens larva fed with organic waste. Waste Manag. 47:84–90. doi:10.1016/j.wasman.2015.03.030.
- Liu Q, Tomberlin JK, Brady JA, Sanford MR, Yu Z. 2008. Black soldier Fly (Diptera: Stratiomyidae) larvae reduce Escherichia coli in dairy manure. Environ Entomol. 37:1525–1530. doi:10.1603/0046-225X-37.6.1525.
- Ma C, Mirth CK, Hall MD, Piper MD. 2022. Amino acid quality modifies the quantitative availability of protein for reproduction in Drosophila melanogaster. J Insect Physiol. 139:104050. doi:10.1016/j.jinsphys.2020.104050.
- MacArthur MR, Mitchell SJ, Treviño-Villarreal JH, Grondin Y, Reynolds JS, Kip P, … Mitchell JR. 2021. Total protein, not amino acid composition, differs in plant-based versus omnivorous dietary patterns and determines metabolic health effects in mice. Cell Metab. 33(9):1808–1819.e2. doi:10.1016/j.cmet.2021.06.011.
- Makinde OJ, Makinde J. 2015. Maggot meal: a sustainable protein source for livestock production-a review. Adv Life Sci Technol. 31:35–42. https://citeseerx.ist.psu.edu/document?repid=rep1&type=pdf&doi=3a969b922507cb43ed93ddb2b92c3cf81e216a5b.
- Makokha MP, Muliro PS, Ngoda PN, Ghemoh CJ, Xavier C, Tanga CM. 2023. Nutritional quality of meat from hen fed diet with full-fat black soldier fly (Hermetia illucens) larvae meal as a substitute to fish meal. J Funct Foods. 101:105430. doi:10.1016/j.jff.2023.105430.
- Meneguz M, Schiavone A, Gai F, Dama A, Lussiana C, Renna M, Gasco L. 2018. Effect of rearing substrate on growth performance, waste reduction efficiency and chemical composition of black soldier fly (Hermetia illucens) larvae. J Sci Food Agric. 98(15):5776–5784. doi:10.1002/jsfa.9127.
- Montesano D, Gallo M, Blasi F, Cossignani L. 2020. Biopeptides from vegetable proteins: new scientific evidences. Curr Opin Food Sci. 31:31–37. doi:10.1016/j.cofs.2019.10.008.
- Palma L, Ceballos SJ, Johnson PC, Niemeier D, Pitesky M, VanderGheynst JS. 2018. Cultivation of black soldier fly larvae on almond byproducts: impacts of aeration and moisture on larvae growth and composition. J Sci Food Agric. 98(15):5893–5900. doi:10.1002/jsfa.9252.
- Pamintuan KRS, Agustin HAT, Deocareza ED. 2020. Growth and nutritional performance of black soldier fly (Hermetia illucens) larvae reared in fermented rice straw and duck manure. IOP Conf Ser: Earth Environ Sci. 505(1):012030. doi:10.1088/1755-1315/505/1/012030.
- Pang W, Hou D, Chen J, Nowar EE, Li Z, Hu R, Tomberlin JK, Yu Z, Li Q, Wang S. (2020). Reducing greenhouse gas emissions and enhancing carbon and nitrogen conversion in food wastes by the black soldier fly. J Environ Manag, 260:110066. doi:10.1016/j.jenvman.2020.110066.
- Pomalégni SCB, Gbemavo DSJC, Kpadé CP, Kenis M, Mensah GA. 2017. Traditional use of fly larvae by small poultry farmers in Benin. J Insects Food Feed. 3(3):187–192. doi:10.3920/JIFF2016.0061.
- Reynolds C, Goucher L, Quested T, Bromley S, Gillick S, Wells VK, Evans D, Koh L, Kanyama AC, Katzeff C, Svenfelt Å. 2019. Review: consumption-stage food waste reduction interventions – what works and how to design better interventions. Food Policy. 83:7–27. doi:10.1016/j.foodpol.2019.01.009.
- Rodrigues DP, Ameixa OM, Vázquez JA, Calado R. 2022. Improving the lipid profile of black soldier fly (Hermetia illucens) larvae for marine aquafeeds: current state of knowledge. Sustainability. 14(11):6472. doi:10.3390/su14116472.
- Salam M, Shahzadi A, Zheng H, Alam F, Nabi G, Dezhi S, … Bilal M. 2022. Effect of different environmental conditions on the growth and development of black soldier fly larvae and its utilization in solid waste management and pollution mitigation. Environ Technol Innov. 28:102649. doi:10.1016/j.eti.2022.102649.
- Salter AM, Lopez-Viso C. 2021. Role of novel protein sources in sustainably meeting future global requirements. Proc Nutr Soc. 80(2):186–194. doi:10.1017/S0029665121000513.
- Scala A, Cammack JA, Salvia R, Scieuzo C, Franco A, Bufo SA, Tomberlin JK, Falabella P. 2020. Rearing substrate impacts growth and macronutrient composition of Hermetia illucens (L.) (Diptera: Stratiomyidae) larvae produced at an industrial scale. Sci Rep. 10(1):19448. doi:10.1038/s41598-020-76571-8.
- Schreven SJJ, Yener S, Van Valenberg HJF, Dicke M, Van Loon JJA. 2021. Life on a piece of cake: performance and fatty acid profiles of black soldier fly larvae fed oilseed by-products. J Insects Food Feed. 7(1):35–49. doi:10.3920/JIFF2020.0004.
- Scieuzo C, Franco A, Salvia R, Triunfo M, Addeo NF, Vozzo S, Piccolo G, Bovera F, Ritieni A, Francia AD, Laginestra A. 2022. Enhancement of fruit byproducts through bioconversion by Hermetia illucens (Diptera: Stratiomyidae). Insect Sci. doi:10.1111/1744-7917.13155.
- Seyedalmoosavi MM, Mielenz M, Veldkamp T, Daş G, Metges CC. 2022. Growth efficiency, intestinal biology, and nutrient utilization and requirements of black soldier fly (Hermetia illucens) larvae compared to monogastric livestock species: a review. J Anim Sci Biotechnol. 13(1):31. doi:10.1186/s40104-022-00682-7.
- Shah NH, Muir TW. 2014. Inteins: nature's gift to protein chemists. Chem Sci. 5(2):446–461. doi:10.1039/C3SC52951G.
- Shumo M, Osuga IM, Khamis FM, Tanga CM, Fiaboe KKM, Subramanian S, Ekesi S, van Huis A, Borgemeister C. 2019. The nutritive value of black soldier fly larvae reared on common organic waste streams in Kenya. Sci Rep. 9:10110. doi:10.1038/s41598-019-46603-z.
- St-Hilaire S, Sheppard C, Tomberlin JK, Irving S, Newton L, McGuire MA, Mosley EE, Hardy RW, Sealey W. 2007. Fly prepupae as a feedstuff for rainbow trout, oncorhynchus mykiss. J World Aquac Soc. 38:59–67. doi:10.1111/j.1749-7345.2006.00073.x.
- Surendra KC, Tomberlin JK, van Huis A, Cammack JA, Heckmann LHL, Khanal SK. 2020. Rethinking organic wastes bioconversion: Evaluating the potential of the black soldier fly (Hermetia illucens (L.)) (Diptera: Stratiomyidae) (BSF). Waste Manag. 117:58–80. doi:10.1016/j.wasman.2020.07.050.
- Tschirner M, Simon A. 2015. Influence of different growing substrates and processing on the nutrient composition of black soldier fly larvae destined for animal feed. J Insects Food Feed. 1(4):249–259. doi:10.3920/JIFF2014.0008.
- Van Huis A, Dunkel FV. 2017. Edible insects: a neglected and promising food source. In: Nadathur SR, Wanasundara JPD, Scanlin L, editors. Sustainable protein sources. Wageningen: Academic Press; p. 341–355.
- Yakti W, Müller M, Klost M, Mewis I, Dannehl D, Ulrichs C. 2023. Physical properties of substrates as a driver for Hermetia illucens (L.) (Diptera: Stratiomyidae) larvae growth. Insects. 14(3):266. doi:10.3390/insects14030266.