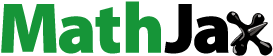
ABSTRACT
This study examined Copper (Cu), Iron (Fe), and Zinc (Zn) levels in Limnothrissa miodon fish from Lake Kariba in Zambia and their potential impact on human health. Two-gram samples from each stratum underwent a 12-hour digestion, and concentrations were determined using Microwave Plasma Atomic Emission Spectrometer. Results indicated that Cu, Fe, and Zn concentrations fell within Food and Agricultural Organization and World Health Organization safety limits. Estimated Daily Intake (EDI) and Hazard Quotient (THQ) values for males and females remained under recommended thresholds, suggesting minimal health risks from consumption. Element concentrations followed the order Fe > Zn > Cu, all below Recommended Daily Allowances (RDAs). THQ values, with Zn posing the highest potential risk followed by Fe and Cu, were under one. Overall, the Hazard Index (HI) was 0.02, signifying a low non-carcinogenic risk from fish consumption. Despite safety, ongoing monitoring of heavy metal accumulation in the ecosystem is advisable for long-term safety. In conclusion, trace element levels in Limnothrissa miodon from Lake Kariba are safe for human consumption with low associated health risks. Nonetheless, continued monitoring of heavy metal levels is vital.
Introduction
Heavy metal pollution, resulting from the release of industrial, agricultural, and household waste into the natural environment, is a significant global environmental concern (Rahmani et al. Citation2018; Hasimuna et al. Citation2022; Mohiuddin et al. Citation2022). Naturally occurring elements like copper (Cu), zinc (Zn), and iron (Fe) are vital components of the Earth's crust and play crucial roles in biological systems (Yahya et al. Citation2018; Hasimuna et al. Citation2021; Simukoko et al. Citation2021). These metals, known as essential elements, have critical functions in cellular organelles and various components like lysosomes, endoplasmic reticulum, cell membranes, nuclei, mitochondria, and certain metabolic enzymes (Wang and Shi Citation2001; Yahya et al. Citation2018).
Despite their importance in biological systems, elevated concentrations of heavy metals can have detrimental effects on ecosystems and human health (Hasimuna et al. Citation2022). Human activities have increased heavy metal levels in both terrestrial and aquatic environments, impacting ecosystem health and product quality (Ustaoglu and Islam Citation2020; Yang et al. Citation2020).
Heavy metals have been investigated in several compartments of aquatic ecosystems in various parts of the globe. For instance, Mishra and Tripathi (Citation2008) studied the capacity of three macrophytes namely Eichhornia crassipes, Spirodela polyrrhiza W. Koch (duckweed) and Pistia stratiotes L. (water lettuce) to effectively remove heavy metals from water. In addition, levels of heavy metals were studied to specify heavy metals in Enteromorpha intestinalis a macrophytic algae and concluded that aquatic algae could be used as an indicator of heavy metal contamination (Yozukmaz et al. Citation2018). To add on, freshwater fish species and or their organs (liver, gills, muscle, brain, and blood plasma) have been investigated for heavy metals contamination (Can et al. Citation2012; Mela et al. Citation2013; Paschoalini and Bazzoli Citation2021; Simukoko et al. Citation2021; Hasimuna et al. Citation2022). Moreover, freshwater mussels have also shown that heavy metal contamination occurs in aquatic environments and has a negative impact on the genes of these species (Aksu et al. Citation2012; Khan et al. Citation2019).
Copper, zinc, and iron are essential trace elements for various biological processes, including those in humans (Yunusa et al. Citation2023). However, excessive levels of these metals in aquatic environments due to pollution can lead to adverse health effects for aquatic organisms and human consumers (Zhang et al. Citation2022; Yunusa et al. Citation2023).
Copper, essential for enzymatic functions and physiological processes, can cause gastrointestinal issues, liver and kidney damage, and neurotoxicity at elevated levels (Alloway Citation2013; Hefnawy and El-Khaiat Citation2015; Rajeshkumar and Li Citation2018; Renu et al. Citation2023). Zinc, vital for immune system regulation, wound healing, enzymatic activities, and metabolic processes, can cause gastrointestinal distress, nausea, vomiting, and potentially impact the immune system and other organs when exposed excessively (Brown et al. Citation2001; Goyer and Clarkson Citation2001; Bredesen Citation2015; Traven et al. Citation2023). Iron, important for oxygen transport, DNA synthesis, and electron transport in the body, can be harmful in high concentrations, leading to gastrointestinal problems and organ damage (Satarug et al. Citation2010; Nazanin et al. Citation2014; Bharti et al. Citation2022; Jomova et al. Citation2022).
Limnothrissa miodon, commonly known as Lake Tanganyika sardine or Kapenta, is a commercially significant fish species native to Lake Tanganyika, providing essential protein for local consumption and export (Muvengwi et al. Citation2012; Hasimuna et al. Citation2019, Citation2023a). This species primarily feeds on planktonic organisms, such as zooplankton and phytoplankton, which are abundant in the open waters they inhabit (Muvengwi et al. Citation2012; Mandima et al. Citation2016). L. miodon was introduced into Lake Kariba from Lake Tanganyika in 1967 and 1969, subsequently supporting the fishing industry in the area within three years (Chali et al. Citation2014; Hasimuna et al. Citation2019, Citation2023a). Lake Kariba, situated on the Zambia-Zimbabwe border, serves as a vital resource for the surrounding communities, providing water for hydropower generation, domestic use, irrigation, and fish as a protein source (Tumbare Citation2008; Hasimuna et al. Citation2019, Citation2023b).
However, the increasing human population and associated human activities around Lake Kariba pose a risk of heavy metal contamination to the fishery. This study aimed to investigate the levels of heavy metal contamination in Limnothrissa miodon in Lake Kariba and assess the potential risks to human health. The objectives of this study were as follows:
To determine the levels of contamination of the trace elements (Cu, Fe, and Zn) in L. miodon in Lake Kariba;
To assess the potential health risks associated with the consumption of L. miodon contaminated with trace elements;
To provide recommendations to mitigate the impact of trace element contamination on L. miodon and the lake ecosystem.
Materials and methods
Sampling sites
The L. miodon samples used in this study were obtained from Lake Kariba which is located at Latitude 16.95 S and Longitude 27.97 E. It covers an area of 5,580 km2 and its storage capacity is 185 km3. The mean depth of the lake is 29 m; the maximum depth is 97 m. On the Zambia side, the lake is divided into four strata for the sake of fisheries management, Stratum I and II are in Sinazongwe, Stratum III is in Gwembe, and Stratum IV is in Siavonga (). This water body was selected for this research because it is a commercial fishery from which one of the highly consumed small fish species L. miodon commercial fish species and a pelagic fishery is caught (Cochrane Citation1984; Magadza Citation2011; Hasimuna et al. Citation2023a). L. miodon is a pelagic clupeid which is an r-selected fish species that reaches maturity at about 35 mm in total length and grows to around 5 cm (Bell-Cross and Bell-Cross Citation1971; Hasimuna et al. Citation2023a). Furthermore, this fish feeds on aquatic insects, crustaceans, phytoplankton, rotifers, and zooplankton. A total of 150 individuals of L. miodon were obtained from randomly selected lake points in each stratum as described by Hasimuna et al. (Citation2023a), giving a total of 600 individuals collected from all the strata. L. miodon was picked for investigation in this study due to its significance in providing micronutrients such as calcium, zinc, vitamin B12 and iron as well as its high accessibility and consumption among the less privileged consumers (Nölle et al. Citation2020). In addition, this species is consumed whole by a large section of the population and any risks to consumers would have far-reaching consequences to the lives of consumers. shows the four strata from which the samples were obtained.
Sample preparation
For the determination of the three trace elements in the whole body of L. miodon, the process involved five (5) basic steps namely: (1) flask preparation, (2) sample preparation, (3) sample digestion, (4) sample dilution, and (5) sample filtration. The apparatus used included the weighing balance, 100 mL volumetric flasks, hot plate, funnels, filter papers, deionized water, fume hood, and hot air oven. The 100 mL volumetric flasks were washed with soap and tap water and soaked in 10% nitric acid for 12 h to avoid any cross-contamination.
The flasks were then rinsed with deionized water (from bench top water purification system at CVRI) and dried in a hot air oven for 90 min. The fresh L. miodon was also dried in the hot air oven to completely remove the moisture and then the samples were ground into powder as a whole in groups. About 2 g of L. miodon samples from each group were weighed and transferred into the sample flasks labelled with respective identification numbers (IDs). A reagent blank was also prepared with no sample added but following all the steps for sample extraction. Twenty (20) mL of 65% nitric acid (Kanto Chemical Co. Japan) was added to the sample flasks and the blank flask. Then 10 mL of 70% perchloric acid (Merck, South Africa) was added in the same way.
The sample flasks were shaken slowly and left to stand in the fume hood for 12 h for acids to digest the samples at ambient temperature. After 12 h of digestion, the samples were placed on the hot plate and boiled until the brown fumes stopped and the samples became clear. The samples were then filtered in 100 mL volumetric flasks and diluted to the mark. Finally, the samples were read using the Microwave Plasma Atomic Emission Spectrometer (MP-AES) 4200, by Agilent Technology, Germany. Sample analysis was done at the Zambia Agricultural Research Institute (ZARI) in Lusaka.
Quality control
To ensure precise and accurate results, standard procedures were followed during sampling as well as laboratory work following the American Public Health Association (APHA) guidelines. [32] L. miodon samples were analyzed for the concentration of the anions and Heavy Metal determination. The multi-parameter analyzer was calibrated with different standards, and daily prepared from standard stock solutions of the respective ions (1000 mg/L) and deionized water. The standardization of the instruments was carried out several times during the procedure for anions and heavy metals. The standards for the four heavy metals (Cu, Fe, and Zn) used were all from PerkinElmer, USA. The readings of all parameters were replicated to check the reliability and accuracy of the instrument, method, and dataset.
For the analysis of heavy metals, three standards and a blank sample were run by the Microwave Plasma Atomic Emission Spectrometer (MP-AES) by Agilent Technology, Germany. The standard and blank samples were repeated after an interval of 10 samples to check the validity of the results. The reproducibility was obtained at a 90 ± 5% confidence level, and the mean value of each sample was used for further analysis. Throughout this study, all the reagents used were of analytical grade and were certified to be pure by the Chemical and Mineral Laboratory of the Copperbelt University, School of Mines and Mineral Sciences, Metallurgy Department. This was necessary for obtaining a calibration curve after diluting a stock standard solution.
Analysis of the three metals
The Cu, Fe, and Zn concentrations in the sample solutions were determined using the MP-AES 4200, by Agilent. Sample analysis was done at the ZARI in Lusaka. Before running the samples for heavy metal analysis, the MP-AES was calibrated and standardized as described in our earlier study (Hasimuna et al. Citation2023a). The concentration of three heavy metals was plotted against the calibration curves of absorbance for each individual which helped to determine the concentration in the fish sample.
Human health risk assessment
Estimated daily intake (EDI)
The following equation (Equation 1) was used to calculate the estimated daily intake of Kariba Kapenta (Ahmed et al. Citation2019):
(1)
(1) where Cn is the average of the mean concentration level of a particular element in the fish (mg/kg dry-wt); IGr is the acceptable ingestion rate, which is 55.5 g/day (Adults, 20 years and older) and 52.5 g/day (Children, 12–19 years). Bwt is the body weight.
Target Hazard Quotient (THQ) for non-carcinogenic risk assessment
The ratio of EDI and the Oral Reference Dose (RfD) was used to estimate THQ. RfDs for Cu, Fe, and Zn are presented in . According to the United States Environmental Protection Agency (USEPA Citation2008), ratio values less than 1 imply non-significant risk effects. THQ formula is expressed as shown in Equation 2 below (USEPA Citation2008):
(2)
(2) where THQ is the Target Hazard Quotient, Ea is the exposure duration; Ep is the exposure frequency; and At is the average time for the non-carcinogenic element (Ea × Ep).
Hazard Index (HI)
The HI was calculated as a summation of the target hazard quotients of the elements (Cu, Fe, and Zn) found in L. miodon for each age group Equation 3.
(3)
(3) Where HI < 1 is safe, > 1 is hazardous, and THQ (Cu) is the target hazard quotient for Cu intake (Baki et al. Citation2018; Hasimuna et al. Citation2023a).
Data analysis
The concentrations of Cu, Fe, and Zn in L. miodon were analyzed using descriptive statistics. Normality and homoscedasticity tests were performed on the means of these four elements using the Shapiro–Wilk and Bartlett tests, respectively. The data exhibited homogeneity and normal distribution, enabling the use of analysis of variance (ANOVA) to compare the mean concentrations of the trace elements (Cu, Fe, and Zn) across the four strata. Post hoc analysis was conducted using the Tukey test to identify significant differences among the means after the ANOVA test. The statistical significance level for all tests was set at 5%. The entire analysis was conducted using R Statistical Software version 4.1.2.
Results
Heavy metal accumulation in the fish
In this study, the cumulative mean concentrations (in mg/kg dry wt.) of all three trace elements in L. miodon in the lake were as follows: Fe (5.55) > Zn (5.113) > Cu (0.152). The mean concentration of iron was the highest followed by that of Zinc, and copper had the lowest concentration (). The mean concentrations of all metals obtained in this study were compared to those of other selected studies as presented in .
Figure 2. Mean concentrations of trace elements in L. Kariba, Zambia. The error bars represent the standard error and different values represent the mean concentration of each element.
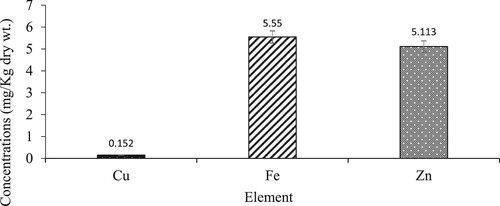
Table 1. Comparison of present study results to selected studies.
The distribution of Cu in L. miodon across the four strata of L. Kariba is shown in . There was a significant (p-value < 0.05) difference in the mean concentrations in (mg/kg dry wt.) of Cu following the order: Stratum I (0.163) > Stratum III (0.149) > Stratum II (0.155) > Stratum IV (0.139). Stratum one had the highest concentration followed by Stratum III while Stratum II and IV had the lowest concentrations respectively. shows the pairwise comparisons of Cu between strata.
Figure 3. Mean concentrations of copper in the four strata of L. Kariba, Zambia. The error bars represent the standard error and different letters show significant differences.
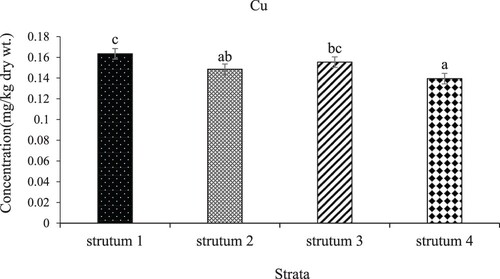
On the other hand, the mean concentration (mg/kg dry wt.) of Fe in L. miodon across the four strata of L. Kariba showed varied amounts across strata (p-value < 0.05): Stratum II (7.149) > Stratum IV (5.959) > Stratum III (5.186) > Stratum I (3.905) having strata pairwise comparisons as shown in . Stratum two had the highest concentration followed by Stratum IV whereas Stratum III and IV had the lowest concentrations.
Figure 4. Mean concentrations of iron in the four strata of L. Kariba, Zambia. The error bars represent the standard error and different letters show significant differences.
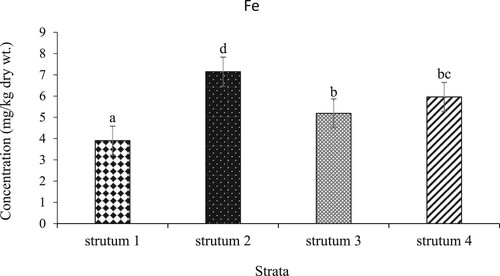
Conversely, the mean concentrations (mg/kg dry wt.) of Zn in L. miodon across the four L. Kariba were significant (p-value < 0.05) across L. Kariba strata. in Stratum II followed by Stratum III (). The general order in terms of distribution was as follows: Stratum II (7.149) > Stratum IV (5.959) > Stratum III (5.186) > Stratum I (3.905) respectively. Pairwise comparisons are shown in .
Assessment of health risks associated with trace elements
The health risk assessment from trace elements was determined by way of comparing the mean concentration of each element against the maximum permissible levels set by international bodies as shown in . In terms of mean concentration across the lake, Copper had the lowest mean concentration followed by zinc and Iron respectively. The mean concentrations were in the order Fe (5.550) > Zn (5.113) > Cu (0.152).
Table 2. Maximum Permissible Limit (MPL) of heavy metals in fish (mg/kg dry wt.) according to international standards.
Estimated Daily Intake (EDI) and the recommended daily allowance RDA
shows a comparison of the EDIs of trace elements in L. miodon and the respective RDAs for selected age groups. Generally, the EDIs were in the order: Fe > Zn > Cu in both adults and were far lower than the RDAs (Moreira et al. Citation2012) for the respective metals.
Table 3. A comparison between estimated daily intake (EDI) and the recommended daily allowance (RDA) for males and females.
Target Hazard Quotient (THQ) and Hazard Index (HI)
The calculated THQs for each trace element in this study were less than one (<1) and were in the order of: Zn (0.014) > Fe (0.006) > Cu (0.000) respectively. On the other hand, the HI was also less than one (HI = 0.02). THQ and HI greater than one (>1) indicates an overall health risk of ingestion of heavy metals through the consumption of food (i.e. fish, invertebrates, and vegetables), and is rated high and classified as harmful (USEPA Citation2008). Since the hazard index (0.020) was less than one, it means that the non-carcinogenic risk effect for the consumers of L. miodon is low.
Discussion
In our present study, the concentration levels of the three prominent heavy metals (Cu, Fe, and Zn) were observed on L. miodon from the four strata of Lake Kariba. Cu is a significant element in all living organisms and is required for cellular respiration, free radical defence, neurotransmitter function, and tissue biosynthesis (Soylak and Unsal Citation2010; Hefnawy and El-Khaiat Citation2015; Hasimuna et al. Citation2022). Excess accumulation of Cu is dangerous to people as it can lead to hepatic cirrhosis, liver and kidney disorder, nausea, diarrhoea, fever, hemolytic anaemia and degeneration of basal ganglia (Behbahani et al. Citation2013; Hefnawy and El-Khaiat Citation2015; Rajeshkumar and Li Citation2018; Korkmaz et al. Citation2019). L. miodon from Lake Kariba had a copper concentration of 0.152 (mg/kg dry wt.) which is below the levels FAO and the joint FAO/WHO recommended maximum permissible limit of 30 mg/kg dry wt (FAO Citation1983; FAO/WHO Citation1989). Berg et al. (Citation1995) also found that Tilapia rendalli and Oreochromis mortimeri had low Cu concentrations from Kassesse Bay of the same lake on the Zimbabwean side. In addition, higher Cu concentrations were found in Stratum I and III while Stratum II and IV had the lowest concentrations respectively. The concentration of Cu just like many other metals is influenced by several natural and anthropogenic sources such as agriculture, domestic wastes, mining, and mineral processing (Hosseini et al. Citation2015; Korkmaz et al. Citation2019; Kwaansa-Ansah et al. Citation2019; Li et al. Citation2020; Hasimuna et al. Citation2021, Citation2022; Hasimuna et al. Citation2023b). The higher levels of Cu in Stratum I are not surprising because this is the stratum that receives water from the Zambezi River which passes through the North-Western Province of Zambia which is one of the two major mining provinces of Zambia (Hasimuna et al. Citation2022) while the main key driver of increased Cu levels of stratum III may be due to runoff, leaching and anthropogenic activities that use Cu-containing compounds, such as pesticides and fertilizers can introduce copper into Lake Kariba (Tumbare Citation2008; Hasimuna et al. Citation2021). However, complex environmental processes affect the concentration of Cu in aquatic ecosystems such as being bound in the sediments. This is similar to the observation made by Rader et al. (Citation2019) who reported that Cu undergoes an adsorption process, naturally precipitating or binding to sediments and suspended particles in water. Within natural water bodies, these interactions can constrain Cu's bioavailability, leading to the transport of Cu from the water column to sediment, thereby reducing the concentration of dissolved Cu within the lake.
Moreover, the low concentration of Cu can also be influenced by Lake Kariba's chemical properties like pH and alkalinity. When conditions encourage the creation of less soluble Cu compounds, it can lead to reduced copper levels in the water. According to Rader et al. (Citation2019), Cu is swiftly removed from natural water systems. In their study, over 70% of the introduced copper was eliminated from the water column within just 16 days of dosing. Cu also has a high affinity and binds to various dissolved ligands and solid phases, affecting its transformation and bioaccumulation in aquatic ecosystems. Cu may bind to various inorganic and organic constituents, such as hydrous manganese oxides and iron oxides, clay, organic matter, and sulfides which will affect the transformations and fluxes of copper between various compartments in the aquatic environment (De Jonge et al. Citation2012; Rader et al. Citation2019). Other studies in sub-Saharan Africa have recorded Cu concentrations lower than the FAO permissible value of 30 mg/kg (Berg et al. Citation1995; Kwaansa-Ansah et al. Citation2019; Kamzati et al. Citation2020; Mannzhi et al. Citation2021). Therefore, the mean concentrations of Cu were within the recommended maximum limit of 30 mg/kg dry wt, hence posing no threat to the health of the fish and the consumers.
Fe is present in aquatic ecosystems in three different forms, however, dissolved ferrous iron (Fe2+) is generally the most bioavailable and readily assimilated by fish and other aquatic organisms (Bury et al. Citation2011). The presence of Fe in fish is important in facilitating several metabolic functions such as oxygen transportation, deoxyribonucleic acid (DNA) synthesis, and electron transportation (Hasimuna et al. Citation2022). Regardless of this, its concentration in the body tissues must be regularly monitored because excessive quantity can cause tissue damage (Nazanin et al. Citation2014). The imbalance of iron causes anaemia, increased likelihood of bacterial infection, and potential susceptibility to other divalent metal toxicity, liver damage, such as Parkinson's, Alzheimer's, type-2 Diab and possibly neurodegenerative diseases (Bury et al. Citation2011; Ardeshir et al. Citation2017). The results of the present study reported a mean concentration of Fe of 5.55 mg/kg dry wt which is below the permissible limit of Fe consumption of 100 mg/kg dry wt, and any levels above this are considered excessive (WHO Citation1989). Similarly, other studies reported lower mean concentrations of Fe in the muscle tissues of various fish species from sub-Saharan Africa (Mannzhi et al. Citation2021; Simukoko et al. Citation2021; Hasimuna et al. Citation2022). The results of the current study are lower than those reported by Kamzati et al. (Citation2020) who indicated a range of 16.342–32.314 mg kg−1 in fish species (e.g. Clarias gariepinus and Oreochromis shiranus chilwae) from an endorheic Lake. Our results also differ from those of Omara et al. (Citation2020), who reported far higher Fe mean concentrations (272.8 ± 0.36 mg/kg) in the muscle of the African lungfish (Protopterus annectens) from the Nyabarongo and Nyabugogo rivers, Rwanda. This could be attributed to the fish species studied (e.g. Clarias gariepinus, Oreochromis shiranus chilwae and Protopterus annectens) being k-selected species unlike our r-selected L. miodon species (Hasimuna et al. Citation2023a) may have accumulated more elements since they have a longer growing period and hence have a longer exposure duration. Unlike our current study in L. Kariba and that of Kamzati et al. (Citation2020) in L. Chilwa which are within the permissible limit, Omara et al. (Citation2020) reported levels beyond the permissible limits in the Nyabarongo and Nyabugogo rivers, Rwanda which could be due to some discharges in the river.
The analysis showed that the mean concentration of Fe in all the strata was within the permissible levels (). The permissible limit of Fe consumption is 100 mg/kg dry wt and any levels above this are considered excessive (WHO, Citation1989). In this analysis, iron mean concentration was highest in stratum two compared to the others with stratum one having the least concentration. Higher Fe concentrations in Stratum II and IV can be attributed to the increased development in agricultural and industrial exacerbated by rapid human population growth (Chifamba Citation2007; Tumbare Citation2008; Hosseini et al. Citation2015). In addition, run-off-induced climate change impacts have increased the transportation of iron-bearing sediments, organic matter, and dissolved iron in water bodies (Anderson et al. Citation2023; Riise et al. Citation2023). An increase in water temperature also enhances the dissolution of iron and other minerals from sediments, increasing the availability of dissolved iron for uptake by aquatic organisms (Félix-Bermúdez et al. Citation2020). Furthermore, Fe is the most abundant and important metal with 98% of it used in steel production, manufacturing, transportation, and agriculture hence it can easily be drained in aquatic ecosystems (Bury et al. Citation2011; Hasimuna et al. Citation2021).
Zn is integral to metalloenzyme function, DNA replication, protein synthesis, and cell division (Brown et al. Citation2001; Bredesen Citation2015; Korkmaz et al. Citation2019). However, excessive levels of Zn in aquatic environments can be toxic to fish and other aquatic organisms, leading to adverse effects on their health and survival. High zinc levels can damage fish gills, which are critical for oxygen uptake and waste elimination (Taslima et al. Citation2022). This can result in respiratory distress. Zn toxicity can disrupt fish reproductive systems, causing reduced egg production, poor egg quality, and decreased hatchability of eggs (Taslima et al. Citation2022). The bioconcentration of Zinc in fish increases with time and the food web with significantly higher Zn levels has been reported to have harmful impacts on people such as causing deficiency of Cu and Fe, nausea, headache, fever, vomiting, skin irritant, tiredness, and adnominal pain (Papagiannis et al. Citation2004; Yi et al. Citation2017; Hasimuna et al. Citation2022).
The current study found a mean concentration of Zn to be 5.113 mg/kg dry wt which was lower than the 40 mg/kg dry wt recommended limit by the joint committee of the Food Agriculture Organization and the World Health Organization (FAO/WHO Citation1989). The results of this study are slightly higher than those reported in other studies in sub-Saharan Africa whose mean concentrations in fish muscles ranged between 0.022 and 4.815 mg/kg (Berg et al. Citation1995; Kwaansa-Ansah et al. Citation2019; Adeogun et al. Citation2020; Kamzati et al. Citation2020; Mannzhi et al. Citation2021). This could be explained due to the differences in species and organs examined (Kotzé et al. Citation1999). Our current study examined the whole fish owing to the size of the fish compared to the other studies which examined individual organs such as the muscle. However, our study is consistent with the findings of Gale et al. (Citation2004) who reported that Whole-body analyses for Zn in small sunfish showed significantly higher (Zn) than those observed in fillets of the same species. This could have led to recording elevated due to critical organs and parts of the fish such as the liver, bones and gonads which are reported to be sites where Zn is stored. This is in agreement with the findings of other studies which reported high levels of Zn in fish which is consumed whole (Gale et al. Citation2004) and in critical organs such as the liver, vertebrae and gonads (Kotzé et al. Citation1999; Murugan et al. Citation2008). Furthermore, higher Zn concentration was reported in Stratum II and Stratum III compared to the other strata. This could be attributed to anthropogenic activities such as agriculture and aquaculture in these areas and the fact that Zn is more mobile compared to other elements like Cu which remain bound up as insoluble complexes in soil and sediments (Kotzé et al. Citation1999; Gale et al. Citation2004; Murugan et al. Citation2008; Yahya et al. Citation2018).
According to Kamzati et al. (Citation2020), Zn can enter water bodies through runoff from agricultural and industrial areas, as well as waste effluent. Surface runoff from semi-urban regions, fertilizer leaching, and waste burning are proposed key factors for heightening zinc levels in Stratum II and Stratum III as they are key agricultural areas (Hasimuna et al. Citation2023a). This could also be due to differences in habitats and land-based activities in strata II and III. This is similar to the observations made by Yap and Al-Mutairi (Citation2022) and Vu et al. (Citation2017) regarding land-based discharges and surface run-offs which were attributed to be the cause of high nutrients and chemicals in the surface waters. These are said to be major niche habitats for reef-associated, pelagic-neritic, and benthopelagic fishes which had accumulated higher mean concentrations of trace elements in the Malaysian Peninsular. Lastly, other possible sources of these metals are chemicals used in industries, boating, tourism and daily life wastewater of humans which eventually gets into aquatic organisms and poses threats as reported in numerous studies (Yozukmaz et al. Citation2018; Guo et al. Citation2020; Johnson et al. Citation2020; Lu et al. Citation2020; Zhang et al. Citation2022). Furthermore, other potential sources of Zn, Cu and Cu to Lake Kariba could be attributed to anthropogenic activities by humans releasing them from natural sources such as sediments both within and outside the water body as was reported from Lake Taihu (Li et al. Citation2022). Further, the perspective of sedimentology that looks at the characteristics of heavy metals and their environmental behaviours will be critical (Yahya et al. Citation2018) by considering metal concentration in the soil as well as the associated ecological and environmental activities.
Health risks analysis
All metals’ THQs tested in this study in both males and females were less than 1. As such, the consumption of the fish species may not threaten the health of consumers. These results are consistent with other results found by Simukoko et al. (Citation2021) in fish from the same lake. Similarly, the HI which is an indicator of the health effect of both single metal exposure and the cumulative effect of multiple metal exposure was less than 1. This indicates that consuming L. miodon regularly does not pose a health threat within the lifetime of the consumer. A study assessing Cu, Zn and other elements in muscles of Sardinella maderensis in the coastal waters of Ghana found HIs that were greater than 1, implying consumption of this fish species may result in some non-carcinogenic effects (Nyarko et al. Citation2023). This difference in findings may be a result of the varied species and degree of pollution of the water bodies assessed. The presence of pollutants (e.g. metals) in fish is dependent on the species, body size, physiological state, feeding patterns, tissue type, metabolite properties, type of pollutant and degree of pollution in the environment (Đikanović et al. Citation2016; Vu et al. Citation2017; Hasimuna et al. Citation2022; Yap and Al-Mutairi Citation2022).
Additionally, L. miodon meets some of the key characteristics of r-selected fish species such as rapid development, small body size and short life span which reduces the levels of trace element accumulation in their body (Reznick et al. Citation2002; Yap and Al-Mutairi Citation2022). To add on, the accumulation of metals in fish has been linked to fish length and size (Li et al. Citation2020; Yap and Al-Mutairi Citation2022), therefore, the small size and length of L. miodon allow this species to have less exposure and risk to the accumulation of metals investigated in this study. Furthermore, based on our estimated EDI and given the RDA, it can be suggested that L. miodon can be consumed by both sexes of any age group without posing any health risk for all the assessed metals and can be a major source of micronutrients (Mphande and Chama Citation2015).
Conclusion
In conclusion, this study delved into the assessment of copper (Cu), iron (Fe), and zinc (Zn) concentrations in L. miodon fish sampled from stratum I, II, and III of Lake Kariba, Zambia, with a focus on potential health implications for consumers. The results of our investigation indicate that the levels of Cu, Fe, and Zn detected in the fish fall below the thresholds associated with health risks for consumers of L. miodon. Thus, based on the findings of this study, we advocate the consumption of L. miodon by individuals of age groups between 19 and 70 years as a source of essential nutrients, particularly micronutrients. Furthermore, this species has a faster growth rate and hence spends less time in the aquatic environment which may bring about less accumulation of trace elements and heavy metals to levels that can cause harm as was found by Hasimuna et al. (Citation2023a). This makes consumption of this species regarding trace and heavy metals safe for human consumption. Nevertheless, it is essential to acknowledge that while the metal concentrations in the fish remain within permissible limits, ongoing agricultural, mining, and other human activities within the region likely contribute to the presence of these trace elements. As a precautionary measure, it is imperative to implement effective monitoring strategies for these anthropogenic activities to mitigate their impact on the lake's fish population. Furthermore, this underscores the importance of raising awareness among stakeholders responsible for Lake Kariba's management. Regulatory bodies, particularly government environmental health management agencies, should establish and enforce stringent regulations to safeguard the lake's ecosystem and the health of its consumers. Periodic monitoring of heavy metal levels in the lake is also recommended, coupled with the adoption of measures to reduce heavy metal contamination, ensuring the long-term ecological health of the lake and the safety of fish consumption.
Authors’ Contribution
Conceptualization: OJH;
Supervision: WWLJ, AHM, JY;
Resources and Funding Acquisition: OJH & WWLJ;
Data Collection and Investigation: OJH, JM, SS;
Materials and Methodology: OJH, MC, WWLJ, AHM, JY;
Writing the Original Draft Manuscript: OJH, JM, KN, CJP, KS;
Data Curation, Software, and Formal Analysis: OJH, IM;
Visualization and Project Administration: OJH, JM;
Validation and Review Editing: WWLJ, AHM, JY, MC;
Additionally, every author reviewed the final manuscript and approved its submission to the journal for publication.
Acknowledgements
The authors wish to express their appreciation to the Zambian Department of Fisheries in the three districts for their invaluable support during this study. Special thanks are also extended to Mr. Moses Fwanyanga and Mr. Gabriel N. Zikita, who generously dedicated their time and provided invaluable assistance during the sample collection process.
Data availability
The data used to support the findings and conclusions of this study are available within the manuscript.
Disclosure statement
No potential conflict of interest was reported by the author(s).
Additional information
Funding
References
- Adeogun AO, Ibor OR, Omiwole R, Chukwuka AV, Adewale AH, Kumuyi O, Arukwe A. 2020. Sex-differences in physiological and oxidative stress responses and heavy metals burden in the black jaw tilapia, Sarotherodon melanotheron from a tropical freshwater dam (Nigeria). Comp Biochem Physiol C Toxicol Pharmacol. 229:108676. doi:10.1016/j.cbpc.2019.108676.
- Ahmed AS, Sultana S, Habib A, Ullah H, Musa N, Hossain MB, Rahman MM, Sarker MSI. 2019. Bioaccumulation of heavy metals in some commercially important fishes from a tropical river estuary suggests a higher potential health risk in children than adults. PLoS One. 14:e0219336. doi:10.1371/journal.pone.0219336.
- Aksu Ö, Yabanli M, Can E, Kutluyer F, Kehayias G, Can ŞS, Kocabaş M, Demir V. 2012. Comparison of heavy metals bioaccumulation by Dreissena polymorpha (Pallas, 1771) and Unio elongatulus eucirrus (Bourguignat, 1860) from Keban Dam Lake, Turkey. Fresenius Environ Bull. 21(7):1942–1947.
- Alloway BJ. 2013. Sources of heavy metals and metalloids in soils. In: Alloway B.J., editor. Heavy metals in soils: trace metals and metalloids in soils and their bioavailability, environmental pollution. Vol. 22. Dordrecht: Springer; p. 11–50. doi:10.1007/978-94-007-4470-7.
- Anderson LE, DeMont I, Dunnington DD, Bjorndahl P, Redden DJ, Brophy MJ, Gagnon GA. 2023. A review of long-term change in surface water natural organic matter concentration in the northern hemisphere and the implications for drinking water treatment. Sci Total Environ. 858:159699. doi:10.1016/j.scitotenv.2022.159699.
- Ardeshir RA, Movahedinia AA, Rastgar S. 2017. Fish liver biomarkers for heavy metal pollution: a review article. American Journal of Toxicology. 2(1):1–8.
- Baki MA, Hossain MM, Akter J, Quraishi SB, Shojib MFH, Ullah AA, Khan MF. 2018. Concentration of heavy metals in seafood (fishes, shrimp, lobster and crabs) and human health assessment in Saint Martin Island, Bangladesh. Ecotoxicol Environ Saf. 159:153–163. doi:10.1016/j.ecoenv.2018.04.035.
- Behbahani M, Salarian M, Amini MM, Sadeghi O, Bagheri A, Bagheri S. 2013. Application of a new functionalized nanoporous silica for simultaneous trace separation and determination of Cd (II), Cu (II), Ni (II), and Pb (II) in food and agricultural products. Food Anal Methods. 6:1320–1329. doi:10.1007/s12161-012-9545-9.
- Bell-Cross G, Bell-Cross B. 1971. Introduction of Limnothrissa miodon and Limnocaridina tanganicae from Lake Tanganyika into Lake Kariba. Fisheries Research Bulletin Zambia. 5:207–214.
- Berg H, Kiibus M, Kautsky N. 1995. Heavy metals in tropical lake Kariba, Zimbabwe. Water, Air, Soil Pollut. 83:237–252. doi:10.1007/BF00477355.
- Bharti JJS, Kumar SS, Kumar V, Kumar A, Kumar D. 2022. A review on the capability of zinc oxide and iron oxide nanomaterials, as a water decontaminating agent: Adsorption and photocatalysis. Appl Water Sci. 12(3):46. doi:10.1007/s13201-021-01566-3.
- Bredesen DE. 2015. Metabolic profiling distinguishes three subtypes of Alzheimer's disease. Aging. 7(8):595–600. doi:10.18632/aging.100801.
- Brown KH, Wuehler SE, Peerson JM. 2001. The importance of zinc in human nutrition and estimation of the global prevalence of zinc deficiency. Food Nutr Bull. 22(2):113–125. doi:10.1177/156482650102200201.
- Bury NR, Boyle D, Cooper CA. 2011. Iron. Fish Physiol. 31(Part A):201–251. doi:10.1016/S1546-5098(11)31004-7.
- Can E, Yabanli M, Kehayias G, Aksu Ö, Kocabaş M, Demir V, Kayim M, Kutluyer F, Şeker S. 2012. Determination of bioaccumulation of heavy metals and selenium in tissues of brown trout Salmo trutta macrostigma (Dumeril, 1858) from Munzur Stream, Tunceli, Turkey. Bull Environ Contam Toxicol. 89:1186–1189. doi:10.1007/s00128-012-0824-3.
- Chali M, Musuka CG, Nyimbili B. 2014. The impact of fishing pressure on Kapenta (Limnothrissa Miodon) production in Lake Kariba, Zambia: a case study of Siavonga District. Int J Agric For Fish. 2014(2):107–116.
- Chifamba PC. 2007. Trace metal contamination of water at a solid waste disposal site at Kariba, Zimbabwe. Afr J Aquat Sci. 32(1):71–78. doi:10.2989/AJAS.2007.32.1.10.147.
- Cochrane KL. 1984. The influence of food availability, breeding seasons and growth rate on commercial catches of Limnothrissa miodon (Boulenger) in Lake Kariba. J Fish Biol. 24(6):623–635. doi:10.1111/j.1095-8649.1984.tb04833.x.
- De Jonge M, Teuchies J, Meire P, Blust R, Bervoets L. 2012. The impact of increased oxygen conditions on metal-contaminated sediments part I: effects on redox status, sediment geochemistry and metal bioavailability. Water Res. 46(7):2205–2214. doi:10.1016/j.watres.2012.01.052.
- Đikanović V, Skorić S, Gačić Z. 2016. Concentrations of metals and trace elements in different tissues of nine fish species from the Međuvršje Reservoir (West Morava River Basin, Serbia). Arch Biol Sci. 68(4):811–819. doi:10.2298/ABS151104069D.
- FAO. 1983. Evaluation of certain food additives and contaminants. Thirty-third Report of the Joint FAO/WHO Expert Committee on Food Additives. World Health Organ Tech Rep Ser. 1989;776:1-64. PMID: 2493707.
- Félix-Bermúdez A, Delgadillo-Hinojosa F, Torres-Delgado EV, Muñoz-Barbosa A. 2020. Does sea surface temperature affect the solubility of iron in mineral dust? The Gulf of California as a case study. J Geophys Res: Oceans. 125(9):e2019JC015999. doi:10.1029/2019JC015999.
- Gale NL, Adams CD, Wixson BG, Loftin KA, Huang Y. 2004. Lead, zinc, copper, and cadmium in fish and sediments from the Big River and Flat River Creek of Missouri’s Old Lead Belt. Environ Geochem Health. 26:37–49. doi:10.1023/B:EGAH.0000020935.89794.57.
- Goyer RA, Clarkson TW. 2001. Toxic effects of metals. In: CD Klaasen, editor. Casarett and Doull’s toxicology: the basic science of poisons. New York: McGraw-Hill; p. 811–867.
- Guo C, Chen Y, Xia W, Qu X, Yuan H, Xie S, Lin LS. 2020. Eutrophication and heavy metal pollution patterns in the water-suppling lakes of China’s south-to-north water diversion project. Sci Total Environ. 711:134543. doi:10.1016/j.scitotenv.2019.134543.
- Hasimuna OJ, Chibesa M, Ellender BR, Maulu S. 2021. Variability of selected heavy metals in surface sediments and ecological risks in the Solwezi and Kifubwa Rivers, North-western Province, Zambia. Sci Afr. 12:e00822. doi:10.1016/j.sciaf.2021.e00822.
- Hasimuna OJ, Chibesa M, Mumbula I, Mphande J, Jere WWL, Phiri D, Nawanzi K, Siavwapa S, Maseko AF, Munganga BP, et al. 2023a. Contamination of selected heavy metals in Limnothrissa miodon (Boulenger, 1906) in the four strata of Lake Kariba Zambia: are the consumers at risk? J Environ Sci Health B. doi:10.1080/03601234.2023.2235262.
- Hasimuna OJ, Maulu S, Chibesa M. 2022. Assessment of heavy metal contamination in water and largescale yellowfish (Labeobarbus marequensis, Smith 1841) from Solwezi River, North-Western Zambia. Cogent Food Agric. 8(1):2121198. doi:10.1080/23311932.2022.2121198.
- Hasimuna OJ, Maulu S, Monde C, Mweemba M. 2019. Cage aquaculture production in Zambia: Assessment of opportunities and challenges on Lake Kariba, Siavonga district. The Egyptian Journal of Aquatic Research. 45(3):281–285. http://dx.doi.org/10.1016/j.ejar.2019.06.007.
- Hasimuna OJ, Maulu S, Nawanzi K, Lundu B, Mphande J, Phiri CJ, Kikamba E, Siankwilimba E, Siavwapa S, Chibesa M. 2023b. Integrated agriculture-aquaculture as an alternative to improving small-scale fish production in Zambia. Front Sustainable Food Syst. 7:1161121. doi:10.3389/fsufs.2023.1161121.
- Hefnawy AE, El-Khaiat HM. 2015. The importance of copper and the effects of its deficiency and toxicity in animal health. Int J Livestock Res. 5(12):1–20. doi:10.5455/ijlr.20151213101704.
- Hosseini SV, Sobhanardakani S, Miandare HK, Harsij M, Regenstein JM. 2015. Determination of toxic (Pb, Cd) and essential (Zn, Mn) metals in canned tuna fish produced in Iran. J Environ Health Sci Eng. 13:59. doi:10.1186/s40201-015-0215-x.
- Johnson A, Jin X, Nakada N, Sumpter J. 2020. Learning from the past and considering the future of chemicals in the environment. Science. 367:384–387. doi:10.1126/science.aay6637.
- Jomova K, Makova M, Alomar SY, Alwasel SH, Nepovimova E, Kuca K, Rhodes CJ, Valko M. 2022. Essential metals in health and disease. Chem-Biol Interact 367:110173. doi:10.1016/j.cbi.2022.110173.
- Kamzati LLJ, Kaonga CC, Mapoma HWT, Thulu FG, Abdel-Dayem SM, Anifowose AJ, Chidya RCG, Chitete-Mawenda U, Sakugawa H. 2020. Heavy metals in water, sediment, fish and associated risks from an endorheic lake located in Southern Africa. Int J Environ Sci Technol. 17:253–266. doi:10.1007/s13762-019-02464-7.
- Khan MI, Zahoor M, Khan A, Gulfam N, Khisroon M. 2019. Bioaccumulation of heavy metals and their genotoxic effect on freshwater mussel. Bull Environ Contam Toxicol. 102:52–58. doi:10.1007/s00128-018-2492-4.
- Korkmaz C, Ay Ö, Ersoysal Y, Köroğlu MA, Erdem C. 2019. Heavy metal levels in muscle tissues of some fish species caught from north-east Mediterranean: evaluation of their effects on human health. J Food Compos Anal. 81:1–9. doi:10.1016/j.jfca.2019.04.005.
- Kotzé PJ, Preez HH, Vuren JV. 1999. Bioaccumulation of copper and zinc in Oreochromis mossambicus and Clarias gariepinus, from the Olifants River, Mpumalanga, South Africa. Water SA. 25:99–110.
- Kwaansa-Ansah EE, Nti SO, Opoku F. 2019. Heavy metals concentration and human health risk assessment in seven commercial fish species from Asafo Market, Ghana. Food Sci Biotechnol. 28(2):569–579. doi:10.1007/s10068-018-0485-z.
- Li J, Miao X, Hao Y, Xie Z, Zou S, Zhou C. 2020. Health risk assessment of metals (Cu, Pb, Zn, Cr, Cd, As, Hg, Se) in angling fish with different lengths collected from Liuzhou, China. Int J Environ Res Public Health. 17(7):2192. doi:10.3390/ijerph17072192.
- Li Y, Cheng X, Liu K, Yu Y, Zhou Y. 2022. A new method for identifying potential hazardous areas of heavy metal pollution in sediments. Water Res. 224:119065. doi:10.1016/j.watres.2022.119065.
- Lu G, Pan K, Zhu A, Dong Y, Wang WX. 2020. Spatial-temporal variations and trends prediction of trace metals in oysters from the Pearl River Estuary of China during 2011–2018. Environ Pollut. 264:114812. doi:10.1016/j.envpol.2020.114812.
- Magadza CHD. 2011. Indications of the effects of climate change on the pelagic fishery of Lake Kariba, Zambia–Zimbabwe. Lakes Reservoirs Res Manage. 16(1):15–22. doi:10.1111/j.1440-1770.2011.00462.x.
- Mandima J, Kortet R, Sarvala J. 2016. Limnothrissa miodon (Boulenger, 1906) in Lake Kariba: daily ration and population food consumption estimates, and potential application to predict the fish stock biomass from prey abundance. Hydrobiologia. 780:99–111. doi:10.1007/s10750-016-2732-8.
- Mannzhi MP, Edokpayi JN, Durowoju OS, Gumbo J, Odiyo JO. 2021. Assessment of selected trace metals in fish feeds, pond water and edible muscles of Oreochromis mossambicus and the evaluation of human health risk associated with its consumption in Vhembe district of Limpopo Province, South Africa. Toxicol Rep. 8:705–717. doi:10.1016/j.toxrep.2021.03.018.
- Mela M, Guiloski IC, Doria HB, Rabitto IS, Da Silva CA, Maraschi AC, Prodocimo V, Freire CA, Randi MAF, Ribeiro CO, de Assis HS. 2013. Risks of waterborne copper exposure to a cultivated freshwater Neotropical catfish (Rhamdia quelen). Ecotoxicol Environ Saf. 88:108–116. doi:10.1016/j.ecoenv.2012.11.002.
- Mishra VK, Tripathi BD. 2008. Concurrent removal and accumulation of heavy metals by the three aquatic macrophytes. Bioresour Technol. 99(15):7091–7097. doi:10.1016/j.biortech.2008.01.002.
- Mohiuddin M, Hossain MB, Ali MM, Hossain MK, Habib A, Afrin S, Rakib MRJ, Rahman MS, Yu QJ, AI-Sadoon MK, et al. 2022. Human health risk assessment for exposure to heavy metals in finfish and shellfish from a tropical estuary. J King Saudi Univ Sci. 34(2):102035. doi:10.1016/j.jksus.2022.102035.
- Moreira DCF, De Sá JSM, Cerqueira IB, Oliveira APF, Morgano MA, Amaya-Farfan J, Quintaes KD. 2012. Mineral inadequacy of oral diets offered to patients in a Brazilian hospital. Nutr Hosp. 27(1):288–297. https://scielo.isciii.es/pdf/nh/v27n1/39_original_28.pdf.
- Mphande J, Chama L. 2015. Preservation methods and storage periods affect the mineral and moisture composition of freshwater fish species. Int J Food Sci Nutr Eng. 5(3):147–153.
- Murugan SS, Karuppasamy R, Poongodi K, Puvaneswari S. 2008. Bioaccumulation pattern of zinc in freshwater fish Channa punctatus (Bloch.) after chronic exposure. Turk J Fish Aquat Sci. 8:55–59. https://www.trjfas.org/abstract.php?id=589.
- Muvengwi J, Muposhi VK, Veremu K, Mbiba M, Nyenda T. 2012. The diet of Limnothrissa miodon and Zooplankton Densities in Sanyati Basin, Lake Kariba. J Environ Sci Eng B. 2012:480–490.
- Nazanin A, Richard H, Roya K. 2014. Review on iron and its importance for human health. J Res Med Sci. 19(2):164–174. https://www.researchgate.net/publication/261957307.
- Nölle N, Genschick S, Schwadorf K, Hrenn H, Brandner S, Biesalski HK. 2020. Fish as a source of (micro) nutrients to combat hidden hunger in Zambia. Food Secur. 12:1385–1406. doi:10.1007/s12571-020-01060-9.
- Nyarko E, Boateng CM, Asamoah O, Edusei MO, Mahu E. 2023. Potential human health risks associated with ingestion of heavy metals through fish consumption in the Gulf of Guinea. Toxicol Rep. 10:117–123. doi:10.1016/j.toxrep.2023.01.005.
- Omara T, Nteziyaremye P, Akaganyira S, Opio DW, Karanja LN, Nyangena DM, Kiptui BJ, Ogwang R, Epiaka SM, Jepchirchir A, Maiyo A. 2020. Physicochemical quality of water and health risks associated with consumption of African lungfish (Protopterus annectens) from Nyabarongo and Nyabugogo rivers, Rwanda. BMC Res Notes. 13(1):1–6. doi:10.1186/s13104-020-4939-z.
- Papagiannis I, Kagalou I, Leonardos J, Petridis D, Kalfakakou V. 2004. Copper and zinc in four freshwater fish species from Lake Pamvotis (Greece). Environ Int. 30(3):357–362. doi:10.1016/j.envint.2003.08.002.
- Paschoalini AL, Bazzoli N. 2021. Heavy metals affecting Neotropical freshwater fish: a review of the last 10 years of research. Aquat Toxicol. 237:105906. doi:10.1016/j.aquatox.2021.105906.
- Rader KJ, Carbonaro RF, van Hullebusch ED, Baken S, Delbeke K. 2019. The fate of copper added to surface water: field, laboratory, and modelling studies. Environ Toxicol Chem. 38(7):1386–1399. doi:10.1002/etc.4440.
- Rahmani J, Fakhri Y, Shahsavani A, Bahmani Z, Urbina MA, Chirumbolo S, Karamti H, Moradi B, Bay A, Bjørklund G. 2018. A systematic review and meta-analysis of metal concentrations in canned tuna fish in Iran and human health risk assessment. Food Chem Toxicol. 118:753–765. doi:10.1016/j.fct.2018.06.023.
- Rajeshkumar S, Li X. 2018. Bioaccumulation of heavy metals in fish species from the Meiliang Bay, Taihu Lake, China. Toxicol Rep. 5:288–295. doi:10.1016/j.toxrep.2018.01.007.
- Renu K, Mukherjee AG, Gopalakrishnan AV, Wanjari UR, Kannampuzha S, Murali R, Veeraraghavan VP, Vinayagam S, Paz-Montelongo S, George A, et al. 2023. Protective effects of macromolecular polyphenols, metal (zinc, selenium, and copper)-Polyphenol complexes, and pectin in different organs with an emphasis on arsenic poisoning: a review. Int J Biol Macromol. 253:126715. doi:10.1016/j.ijbiomac.2023.126715.
- Reznick D, Bryant MJ, Bashey F. 2002. r and k-selection revisited: the role of population regulation in life-history evolution. Ecology. 83(6):1509–1520. doi:10.1890/0012-9658(2002)083[1509:RAKSRT]2.0.CO;2.
- Riise G, Haaland SL, Xiao Y. 2023. Coupling of iron and dissolved organic matter in lakes–selective retention of different size fractions. Aquat Sci. 85(2):57. doi:10.1007/s00027-023-00956-w.
- Satarug S, Garret SH, Sens MA, Sens DA, Pongrakhananon V. 2010. Heavy metals induce oxidative stress and trigger oxidative DNA damage and apoptosis in renal and hepatic cells. Toxicol Lett. 133(1):45–52.
- Simukoko CK, Mwakalapa EB, Bwalya P, Muzandu K, Berg V, Mutoloki S, Polder A, Lyche JL. 2021. Assessment of heavy metals in wild and farmed tilapia (Oreochromis niloticus) on Lake Kariba, Zambia: implications for human and fish health. Food Addit Contam A. 39:74–91 doi:10.1080/19440049.2021.1975830.
- Soylak M, Unsal YE. 2010. Chromium and iron determinations in food and herbal plant samples by atomic absorption spectrometry after solid phase extraction on single-walled carbon nanotubes (SWCNTs) disk. Food Chem Toxicol. 48(6):1511–1515. doi:10.1016/j.fct.2010.03.017.
- Taslima K, Al-Emran M, Rahman MS, Hasan J, Ferdous Z, Rohani MF, Shahjahan M. 2022. Impacts of heavy metals on early development, growth and reproduction of fish–A review. Toxicol Rep. 9:858–868. doi:10.1016/j.toxrep.2022.04.013.
- Traven L, Marinac-Pupavac S, Žurga P, Linšak Ž, Žeželj SP, Glad M, Linšak DT, Cenov A. 2023. Arsenic (As), copper (Cu), zinc (Zn) and selenium (Se) in northwest Croatian seafood: a health risks assessment. Toxicol Rep. 11:413–419. doi:10.1016/j.toxrep.2023.10.012.
- Tumbare MJ. 2008. Managing Lake Kariba sustainably: threats and challenges. Zambezi River Authority, Lusaka, Zambia. Management of Environmental Quality an International Journal. 19(6):731–739. doi:10.1108/14777830810904948.
- [USEPA] United States Environmental Protection Agency. 2008. Human health risk assessment protocol for hazardous waste combustion facilities. EPA530-R-05-006.
- Ustaoglu F, Islam MS. 2020. Potential toxic elements in the sediment of some rivers at Giresun, Northeast Turkey: A preliminary assessment for ecotoxicological status and health risk. Ecol Indic. 113:106237. doi:10.1016/j.ecolind.2020.106237.
- Vu CT, Lin C, Yeh G, Villanueva MC. 2017. Bioaccumulation and potential sources of heavy metal contamination in fish species in Taiwan: assessment and possible human health implications. Environ Sci Pollut Res Int. 24(23):19422–19434. doi:10.1007/s11356-017-9590-4.
- Wang S, Shi X. 2001. Molecular mechanisms of metal toxicity and carcinogenesis. Mol Cell Biochem. 222(1–2):3–9. doi:10.1023/A:1017918013293.
- WHO. 1989. Toxicological evaluation of certain food additives and contaminants. WHO Food Additives Series, No. 24. Cambridge University Press.
- Yahya AN, Mohamed SK, Mohamed AG. 2018. Environmental pollution by heavy metals in the aquatic ecosystems of Egypt. Open Access J Toxicol. 3(1):555603. doi:10.19080/OAJT.2018.03.555603.
- Yang QQ, Wang SL, Liu WJ, Yang YW, Jiang SQ. 2020. Spatial distribution of perfluoroalkyl acids (PFAAs) and their precursors and conversion of precursors in seawater deeply affected by a city in China. Ecotoxicol Environ Saf 194:110404. doi:10.1016/j.ecoenv.2020.110404.
- Yap CK, Al-Mutairi KA. 2022. Copper and zinc levels in commercial marine fish from Setiu, east coast of Peninsular Malaysia. Toxics. 10(2):52. doi:10.3390/toxics10020052.
- Yi Y, Tang C, Yi T, Yang Z, Zhang S. 2017. Health risk assessment of heavy metals in fish and accumulation patterns in the food web in the upper Yangtze River, China. Ecotoxicol Environ Saf. 145:295–302. doi:10.1016/j.ecoenv.2017.07.022.
- Yozukmaz A, Yabanli M, Sel F. 2018. Heavy metal bioaccumulation in Enteromorpha intestinalis,(L.) Nees, a macrophytic algae: the example of Kadin Creek (Western Anatolia). Braz Arch Biol Technol. 61. doi:10.1590/1678-4324-2018160777.
- Yunusa MA, Igwe EC, Mofoluke AO. 2023. Heavy metals contamination of water and fish-a review. FUDMA J Sci. 7(1):110–118. doi:10.33003/fjs-2023-0701-1255.
- Zhang Y, Zhang M, Yu W, Li J, Kong D. 2022. Ecotoxicological risk ranking of 19 metals in the lower Yangtze River of China based on their threats to aquatic wildlife. Sci Total Environ. 812:152370. doi:10.1016/j.scitotenv.2021.152370.