ABSTRACT
This experiment is to investigate whether interspecies differences exist in the effects of leucine (Leu) on digestive enzyme secretion in pancreatic tissues through in vitro. Five healthy Simmental cattle and Duroc × Landrace × Yorkshire growing-finishing pigs were selected, and pancreatic tissues were taken for culture after slaughter. Leu levels of 0, 2.62, 5.24, 10.48 mg/mL. Leu levels of 0, 5.24 mg/mL were selected to determine the expression of mRNA and proteins in the signaling pathways. The results showed that Leu increased the activity of digestive enzyme in cattle and pig, and there was an interaction was found on trypsin and chymotrypsin (P < 0.05). Leu had a significant effect on the mRNA (PKB, S6K1, 4E-BP1 and ATF4) and protein expression (PKB and 4E-BP1) and the phosphorylation (PKB, S6K1 and ATF4) levels (P < 0.05). Animal species had significant effects on the mRNA (PKB, S6K1 and ATF4) and protein (S6K1 and 4E-BP1) expression, and the phosphorylation (4E-BP1) levels (P < 0.05). There was an interaction between animal species and Leu on the expression of protein (PKB and S6K1) (P < 0.05). In summary, interspecies differences exist in the regulation of Leu on digestive enzyme secretion in pancreatic tissues in vitro of different animals.
KEYWORDS:
1. Introduction
The exocrine function of the pancreas has a very important role in the digestion and absorption of food. Starch is the main source of energy in mammals and over 90% is digested in the small intestine of monogastric animals, while the small intestine of ruminants can digest only 40% – 62% of preruminal starch (Harmon et al. Citation2004). Studies have shown that pancreas α-amylase secretion limits the digestion of starch in the small intestine (Harmon et al. Citation2004; Huntington et al. Citation2006). Several studies have shown that insufficient secretion of pancreatic α-amylase is the limiting factor in the utilization of rumen bypass starch (Swanson et al. Citation2002; Harmon et al. Citation2004). The research group has proven that duodenal perfusion of Leu or phenylalanine can increase pancreas α-amylase (Yu et al. Citation2014a; Yu et al. Citation2014b). Amino acids affect the rate of translation initiation and elongation mainly through two signaling pathways, mTOR and ISR. When the body has sufficient amino acid intake, the body will activate the mTOR signaling pathway to maintain amino acid homeostasis by increasing the consumption of amino acids. When there is an amino acid deficiency in the body, the body initiates the ISR signaling pathway to maintain amino acid balance in the body by decreasing amino acid consumption and increasing the source of amino acids (Guo et al., Citation2018a).
In recent years, many scholars have studied the effect of Leu on pancreatic digestive enzyme secretion in different animals, but there are few reports on the difference between the regulatory mechanism of pancreatic digestive enzyme secretion in monogastric animals and ruminants. Cattle and pigs are typical ruminants and monogastric animals, respectively, so we chose cattle and pigs as study model. Based on this, this study used the method of animal pancreatic tissue cultured in vitro to compare the effects of Leu concentration on pancreatic digestive enzyme secretion of cattle and pigs, and reveal the differences in pancreatic digestive enzyme secretion between monogastric animals and ruminants.
2. Material and methods
All study procedures were reviewed and approved by the Institutional Animal Care and Use Committee at Inner Mongolia Agricultural University of China and were performed in accordance with the guiding principles for the care and use of laboratory animals. All animal experiments took place at Inner Mongolia Agricultural University of China.
2.1. Animals and experimental design
Five healthy Simmental cattle (male, 12 months of age, 538 ± 19.5 kg) and Duroc × Landrace × Yorkshire growing-finishing pigs (male, 8 months of age, 214 ± 13.6 kg) were selected. These animals were euthanized by captive bolt pistol after 30 days of feeding (the ingredients and nutrient composition of the starter are shown in ). The pancreas was removed immediately, washed with cold saline (0.9% NaCl), sent to the laboratory in cold saline, placed on ice for tissue stripping and cut into 2 × 2 mm small segments (approximately 100 mg) that were blotted dry with paper towels and weighted using a glass weigh funnel. Incubation of pancreatic tissue was performed using methods described by Swanson et al. (Citation2003). The Leu concentration gradients were set to 0, 2.62, 5.24 and 10.48 mg/mL, with 5 repetitions for each treatment. Place the culture plate in a self-sealing bag and oxygenate to ensure 95% oxygen concentration and 5% carbon dioxide concentration. The samples were placed into a 37℃ constant temperature water bath shaker with a vibration frequency of 90 times/min. After culture for 1 h, the culture plate was placed on ice, and the pancreatic tissue was collected and stored in a cryopreservation tube at −20℃ for measuring the enzyme activity in the tissue (α-amylase, lipase, trypsin, chymotrypsin).
Table 1. Ingredients and chemical composition of the cattle and pigs (air-dry basis).
Two groups of samples with Leu concentrations of 0 and 5.24 mg/mL were selected for the determination of the expression of key genes and proteins in the mTOR and ISR signaling pathways.
2.2. Sample collection
At the end of the incubation time, amino acid culture plates with different concentrations of substrates were removed and placed on ice. Then, 700 μL of KRB solution was extracted from each well of the culture plate with a pipette gun and stored in a labeled freezing tube at −20℃ until analysis for enzyme activity release. A separate aliquot of tissue was homogenized in saline and stored in a labeled freezing tube at −80°C until analysis of enzyme activities.
KRB and homogenate samples were used to determine α-amylase activity (Walker and Harmon Citation1996). The activities of trypsin and lipase were analyzed by using the method of Xu et al. (Citation2009). Detection kits (Nanjing Jiancheng Bioengineering Institute, Nanjing, China) were used to analyze chymotrypsin activities within one week. Enzyme activities were expressed in units (one unit is defined as 1 μmol of the product released per minute at 39°C).
2.3. In vitro enzyme release
To measure the activity of digestive enzymes in the tissue and incubated solution, we collected the supernatant of the homogenate. The activities of α-amylase, trypsin, lipase and chymotrypsin in the supernatant of the homogenate and culture medium were determined using commercial kits (Nanjing Jiancheng Bioengineering Institute, China). One unit of enzyme activity was defined as 1 μmol of product released per minute at 39℃.
2.4. Quantification of mRNA levels
RNA was isolated from the pancreas samples according to the method described by previous studies (Wathes et al. Citation2009). The total RNA concentration was quantified by measuring its absorbance at 260 nm using a NanoDrop 2000 spectrophotometer (Thermo Scientific Inc. Wilmington, DE, USA). Reverse transcription (RT) of the mRNA was performed using a PrimeScript® RT Reagent Kit (TaKaRa Biotechnology Co., Ltd., Dalian, China). The primers used for PCR are listed in and . The mRNA levels of various tissues were assessed using the 2− ΔΔCt method (Livak and Schmittgen Citation2001).
Table 2. The PCR primers used to amplify the genes encoding PKB, 4E-BP1, S6K1, eIF-2α, ATF4 and β-actin in pig.
Table 3. The PCR primers used to amplify the genes encoding PKB, 4E-BP1, S6K1, eIF-2α, ATF4 and β-actin in cattle.
2.5. Protein immunoblot analysis
The total protein extraction kit (with Protease Inhibitor Cocktail) was used to extract the sample proteins, and then the BCA quantification kit was used for total protein quantification. An 8-12% separation gel and 5% concentrate gel were prepared, with 60 μg of total protein per well for loading, 10–15 μl per well, 60 V for concentrate and 80 V for separation gel for electrophoresis for approximaately 2 hr. The PVDF membrane was soaked in methanol for 20 sec, and then transferred to Tris-glycine transfer buffer (containing 5% methanol) for equilibration for at least 5 min; SDS-PAGE gels were equilibrated in Tris-glycine transfer buffer for at least 30 min; the membranes were transferred at a constant pressure of 100 V with full humidity for 2 hr under cooling conditions. After the end of the transfer, the membrane was put into T-TBS (containing 5% skim milk powder or BSA), blocked at room temperature for 1 hr, and then T-TBS rinsed for 5 min × 3. The primary antibodies used were rabbit anti-goat and mouse anti-goat polyclonal serum antibodies (4E-BP1 and PKB from Calbiochem, Germany; S6K1, ATF4 and eIF-2α from Abcam, UK), and the secondary antibodies were goat anti-rabbit and goat anti-mouse horseradish peroxidase conjugates (Beijing Synthetic Technology Co., Ltd., China). The primary antibody was dissolved in T-TBS (containing 3% skim milk powder or BSA) in a certain proportion and incubated overnight at 4°C; then, T-TBS was rinsed for 5 min × 4. The secondary antibody was dissolved in T-TBS (containing 2% skim milk powder) in a certain proportion and incubated for 1 h at room temperature; then T-TBS was rinsed for 5 min × 5. Using SuperSignal® West Dura Extended Duration Substrate, prepare approximately 1 ml of ECL working solution according to the instructions, incubate the transfer film at room temperature for 1 min, remove the excess ECL reagent, seal the film with plastic wrap, place the X-ray film in the dark box for 5-10 min exposure and then develop and fix the film. The optical density values of the bands were analyzed by BandScan 5.0 software, and each band was repeated three times. The relative expression of the target protein = {target protein (optical density value)/internal reference (optical density value)}×10n was expressed, and the results were expressed as the mean ± standard deviation.
2.6. Statistical analysis
The data for the digestive enzyme activities were analyzed using the general linear model procedure of SAS 9.2 (SAS Institute, Cary, NC, USA) to determine the effects of Leu. Orthogonal polynomial contrasts (linear and quadratic) were used to characterize the effect of Leu concentration on pancreas enzymatic parameters. The highest order contrast (linear and quadratic) that was statistically significant is reported. Statistical models included main effects for Leu, animals and their interactions. The data were analyzed by variance analysis and t test in SAS 9.2 software. The results are reported as the least squares means ± standard errors of the means, and a treatment difference was declared significant at P < 0.05.
3. Results
3.1. Digestive enzyme secretion
As shown in , levels of Leu linearly increase the α-amylase, trypsin and chymotrypsin activity in tissue (P < 0.01) and incubated solution (P < 0.01). Compared with the control group (0 mg/mL), Leu had no effect on lipase activity release from tissue (P > 0.05) but decreased lipase activity in incubated solution (P < 0.01).
Table 4. Interaction of Leu on pancreatic enzyme activity of different animals in vitro.
High levels of Leu (5.24 and 10.48 mg/mL) increased the α-amylase and lipase activity in tissue (P < 0.01) and α-amylase, lipase, trypsin, chymotrypsin release in incubated solution (P < 0.01); the activity of chymotrypsin in tissues tended to increase and then decrease with increasing Leu concentration (P < 0.01) and reached the peak value at 5.24 mg/mL, while it had no influence on the activity of trypsin (P > 0.05).
There was a significant effect of animal species on the secretion of lipase and trypsin from pancreatic tissue (P < 0.05) and a significant effect on the activity of lipase, trypsin and chymotrypsin released from the incubated solution (P < 0.01). The Leu concentration had a significant effect on α-amylase, trypsin and chymotrypsin secreted by pancreatic tissue (P < 0.01) and on the activity of α-amylase, trypsin and chymotrypsin released from the incubated solution (P < 0.01). There was an interactive effect of animal species and Leu concentration on the activity of trypsin and chymotrypsin secreted by pancreatic tissue (P < 0.01) and on the activity of lipase, trypsin and chymotrypsin released from the incubated solution (P < 0.01). Combining the previous results of our group with the results of Experiment 1, the optimal concentration to promote pancreatic enzyme secretion was concluded to be 5.24 mg/mL, so it was used as the Leu incubation concentration for Experiment 2.
3.2. mTOR Pathway
As shown in , the mRNA expression of the PKB and S6K1 genes in the mTOR signaling pathway in animal pancreatic tissues differed significantly by animal species (P < 0.01), and the effect of 4E-BP1 gene mRNA expression tended to be significant (P = 0.051). The Leu concentration had a significant effect on the mRNA expression of the PKB, 4E-BP1 and S6K1 genes (P < 0.05). Animal species and Leu concentration had an interactive effect on PKB and 4E-BP1 gene mRNA expression (P < 0.01) and no interaction on S6K1 (P > 0.05).
Figure 1. The effect of Leu on the expression of key genes in the mTOR pathway in the pancreatic tissue of cattle and pigs in vitro.
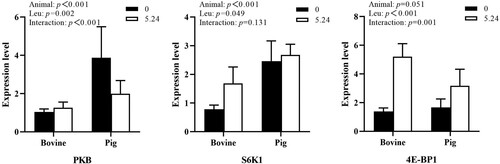
As shown in , there was a significant effect of animal species on 4E-BP1 and SK61 protein expression in cattle and pig pancreatic tissues in vitro (P < 0.01) and a nonsignificant effect on PKB protein expression (P > 0.05), i.e. protein expression of 4E-BP1 and S6K1 in cattle and pig pancreatic tissues differed at certain leucine concentrations. The Leu concentration had a significant effect on PKB and 4E-BP1 protein expression in cattle and pig pancreatic tissues (P < 0.01) but not on S6K1 protein expression (P > 0.05). There was an interactive effect of animal species and Leu concentration on the expression of PKB, 4E-BP1 and S6K1 in cattle and pig pancreatic tissues (P < 0.05).
Figure 2. The effect of Leu on the expression and phosphorylation of key proteins in the mTOR signaling pathway in the pancreatic tissue of cattle and pigs in vitro.
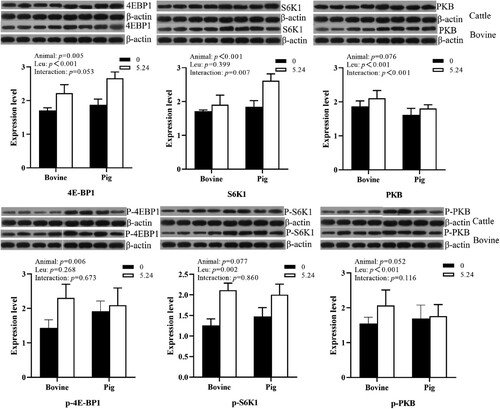
Animal species had a significant effect on the expression of PKB and 4E-BP1 protein phosphorylation levels in cattle and pig pancreatic tissues (P < 0.05); Leu concentration significantly increased the phosphorylation levels of PKB, S6K1 protein (P < 0.01); and there was no interaction between animal species and Leu concentration on protein phosphorylation levels (P > 0.05).
3.3. ISR Pathway
As shown in , animal species had a significant effect on ATF4 gene mRNA expression in cattle and pig in vitro pancreatic tissues (P < 0.01); Leu had a significant effect on ATF4 gene mRNA expression (P < 0.01); animal species and Leu concentration had an interactive effect on ATF4 gene mRNA expression (P < 0.01) and no interactive effect on eIF-2α gene mRNA expression (P > 0.05).
Figure 3. The effect of Leu on the expression of key genes in the ISR pathway in the pancreatic tissue of cattle and pigs in vitro.
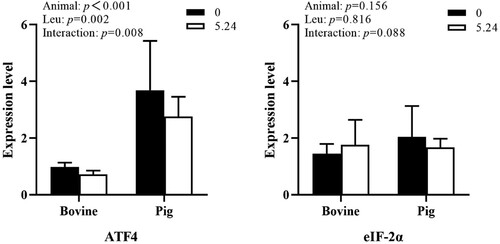
As shown in , animal species had a significant effect (P < 0.05) on ATF4 and eIF-2α protein expression in cattle and pig in vitro pancreatic tissues. At the same Leu concentration, the protein expression of ATF4 and eIF-2α in pig pancreatic tissues was higher than that in cattle pancreatic tissues. Leu was able to significantly affect ATF4 protein expression (P < 0.01). There was an interactive effect of animal species and Leu concentration on ATF4 protein expression, but no interactive effect on eIF-2α protein expression (P > 0.05).
Figure 4. The effect of Leu on the expression and phosphorylation of key proteins in the ISR signaling pathway in the pancreatic tissue of cattle and pigs in vitro.
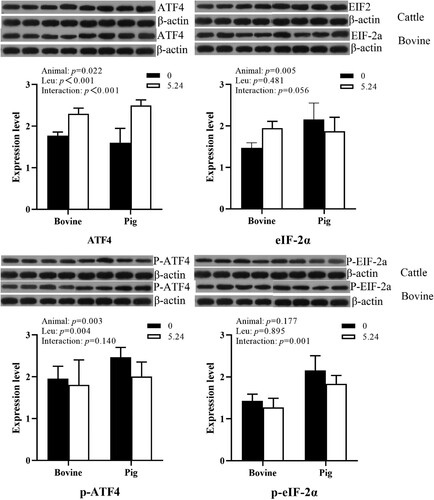
Animal species had a significant effect (P < 0.01) on the phosphorylation level of ATF protein in cattle and pig in vitro pancreatic tissues; Leu significantly affected the phosphorylation level of ATF4 protein (P < 0.01); there was no interaction between animal species and Leu on the phosphorylation level of ATF4 protein (P > 0.05) and an interaction on the phosphorylation level of eIF-2α (P < 0.05).
4. Discussion
Few studies have directly compared the differences between animals, particularly monogastric animals with strong small intestinal digestion, and ruminants with poor digestion and a key limiting factor being inadequate pancreatic amylase secretion (Harmon et al. Citation2004; Huntington et al. Citation2006; Xu et al. Citation2009). Therefore, the aim of this experiment was to compare the differences in pancreatic digestive enzyme secretion as well as regulatory pathways between cattle and pig pancreatic digestive enzymes through in vitro studies. The exocrine function of pancreatic tissue is evaluated by means of in vitro tissue culture. This method allows the exclusion of other factors and thus can better help us to study the effect of nutrients on the secretory function of the pancreas (Swanson et al. Citation2003). Leu is one of the BBCAs that mainly regulates protein metabolism. Studies have shown that the addition of pre-ruminal leucine to the diet improves small intestinal starch digestibility in dairy cows (Ren et al. Citation2020). In addition, Leu supplementation can affect the development and growth of pancreas in Holstein calves (Cao et al., Citation2018a). Studies have also shown that the addition of Leu into milk does not affect the activities of α-amylase, lipase and trypsin in the pancreas of Holstein calves (Cao et al., Citation2018a; Guo et al., Citation2018b). In contrast, in monogastric animals, Leu can inhibit the expression of pancreatic α-amylase mRNA and reduce the secretion of α-amylase (Hashimoto and Hara Citation2003, Citation2004). Existing studies have demonstrated that the effect of duodenal proteins on pancreatic α-amylase gene expression and secretion differs between ruminants and monogastrics, with monogastrics (Brannon Citation1990) showing a decrease but ruminants (Richards et al. Citation2003; Swanson et al. Citation2004; Suryawan et al. Citation2011) showing an increase in gene expression and secretion. It is hypothesized that there are interspecies differences in the regulation of pancreatic digestive enzyme secretion by Leu.
This experiment combined with previous studies, through the method of tissue culture in vitro, to study the effect of leucine on the activity of pancreatic digestive enzymes in vitro in cattle and pigs and whether there are interspecific differences in pancreatic digestive enzymes. When the in vitro tissue incubation method is used, most of the other factors that could affect the synthesis of enzymes are excluded, and Leu remains as the only main factor regulating pancreatic exocrine function; therefore, its effects on the synthesis of enzymes should be much more distinct.
Mammalian target of rapamycin (mTOR) is a kinase that serves as a core catalytic component of mTOR complex 1 (mTORC1) and mTOR complex 2; the mTOR pathway plays a very important role in the translation initiation and is the central regulator of metabolism in animal bodies (Schalm and Blenis Citation2002). The best-characterized downstream effectors of mTOR include two signaling pathways that act in parallel to control mRNA translation: the 70-kDa ribosomal protein S6 kinase 1 (S6K1) pathway and the eukaryotic translation initiation factor 4E binding protein 4 (4EBP1) pathway (Fingar et al. Citation2004). mTOR activated by amino acids, catalyzes the phosphorylation of ribosomal proteins S6K1 and 4EBP1 (Wang and Proud Citation2006). Integrated stress response (ISR) is a complex signal pathway in eukaryotic cells that is activated under the influence of external factors such as cell oxidative stress, amino acid deficiency, glucose deficiency and virus infection (Harding et al. Citation2003). The results of this trial showed that Leu increased the secretion of digestive enzymes in cattle and porcine pancreatic tissue and upregulated mRNA expression and protein phosphorylation levels of key genes in the mTOR and ISR signaling pathways. When the body has sufficient amino acid intake, the body will activate the mTOR signaling pathway to maintain amino acid homeostasis by increasing the consumption of amino acids. Leu stimulates milk protein synthesis in dairy cows through the mTOR signaling pathway (Harding et al. Citation2003; Wang and Proud 2006; Yu et al. Citation2013). Generally, in monogastric, Leu decreased the mRNA expression of α-amylase and inhibited the secretion of α-amylase (Hashimoto and Hara Citation2003, 2004). Leu was found to enhance the activity of α-amylase, trypsin and chymotrypsin in pancreatic tissue in vitro in dairy goats (Yu et al. Citation2013). Leu infusion in the duodenum increased the activity of small intestinal trypsin and lipase in goats (Cao et al., Citation2018b). The results of this experiment showed that Leu could enhance the activity of α-amylase, trypsin and chymotrypsin in pancreatic tissues and culture fluid, which is consistent with previous results. When BBCA diet was fed to rats, Leu could increased the phosphorylation level of 4E-BP1 and S6K1 protein in rat pancreatic alveolar cells (Anthony et al. Citation2000; Sans et al. Citation2006). Studies have shown that Leu deficiency can lead to the phosphorylation of eIF-2α in mouse liver and increase the expression of the ATF4 gene. After Leu intake, it reduces the phosphorylation level of eIF-2α and the expression of the ATF4 gene (Carraro et al. Citation2010). Leu could regulate the synthesis of pancreatic enzymes by increasing the mRNA expression and the phosphorylation level of protein factors in the mTOR pathway (Cao et al. Citation2019). The above results are consistent with the results of this assay that leucine can enhance the secretion of pancreatic digestive enzymes through the regulation of mTOR and ISR signaling pathways.
The results of this experiment showed that pancreatic digestive enzyme secretion differs between cattle and pigs, and that mRNA expression and phosphorylation levels of key genes in the mTOR and ISR signaling pathways differ between animals. The differences in nutrient utilization between ruminants and monogastrics are mainly due to the different feeds consumed over a long evolutionary period, and the physiological processes of digestion and absorption are much more complex in ruminants than in monogastrics. For ruminants, nutrients are generally first fermented by rumen microorganisms, whereas monogastric animals are absorbed through digestive juices that breakdown the nutrients into simpler forms. Because monogastric animals do not have amylase-secreting glands, starch cannot be degraded in the stomach and goes directly to the small intestine, which contains a variety of digestive juices, such as pancreatic juice. 15%−25% of undigested starch in the rumen enters the small intestine directly (Harmon Citation2009), but the digestibility of starch in the small intestine of ruminants is limited, with only 40%–62% of the pre-rumen starch being digested (Harmon et al. Citation2004). This is consistent with the results of this trial, which showed interspecific differences in mRNA expression and protein phosphorylation levels of key genes of the mTOR and ISR signaling pathways in cattle and pigs. The results of this test found that cattle pancreatic α-amylase secretion was higher than that of pigs, while the ratio of volume to body weight in cattle (Cao et al., Citation2018b) pancreas was smaller than that of pig (Wang Citation2003), indicating that in the absence of other factors interfering (consistent pancreas weight), the secretion of digestive enzymes in bovine pancreas is not weaker than in pigs.
Existing studies have demonstrated that the effect of duodenal proteins on pancreatic α-amylase gene expression and secretion differs between ruminants and monogastrics, with monogastrics (Brannon Citation1990) showing a decrease but ruminants (Richards et al. Citation2003; Swanson et al., Citation2004; Suryawan et al., Citation2011) showing an increase in gene expression and secretion. However, there are no studies directly comparing whether there are interspecies differences in the secretion of pancreatic digestive enzymes by Leu in monogastric and ruminant animals. The results of the present study revealed that Leu interacted with pancreatic digestive enzyme secretion and regulatory pathways in different animals.
5. Conclusions
The results of this study showed that there were interspecies differences between monogastric and ruminant pancreatic digestive enzyme secretion in vitro; cattle pancreatic digestive enzyme secretion was higher than that of pigs, and Leu regulated the secretion of digestive enzymes in cattle and pig pancreatic tissues in vitro through the mTOR and ISR signaling pathways, with different regulatory mechanisms and interspecies differences in the two signaling pathways.
Author contribution
Zhanhe Zhang and Ming Xu conceived and designed the experiments; Zhanhe Zhang, Xuan Ma, Mengya Wang and Ru Li performed the experiments; Donglin Wu and Kai Shao contributed reagents, materials and analysis tools; and Zhanhe Zhang and Xuan Ma wrote the paper. All authors read and approved the final manuscript.
Disclosure statement
No potential conflict of interest was reported by the author(s).
Data availability statement
The data used to support the findings of this study are available from the corresponding author upon request.
Additional information
Funding
References
- Anthony JC, Anthony TG, Kimball SR, Vary TC, Jefferson LS. 2000. Orally administered leucine stimulates protein synthesis in skeletal muscle of postabsorptive rats in association with increased eIF4F formation. The Journal of Nutrition. 130(2):139–145. doi: 10.1093/jn/130.2.139.
- Brannon PM. 1990. Adaptation of the exocrine pancreas to diet. Annual Review of Nutrition. 10:85–105. doi: 10.1146/annurev.nu.10.070190.000505.
- Cao Y, Liu K, Liu S, Guo L, Yao J, Cai C. 2019. Leucine regulates the exocrine function in pancreatic tissue of dairy goats in vitro. BioMed Research International. 2019:7521715.
- Cao Y, Yang X, Guo L, Zheng C, Wang D, Cai C, Yao JA. 2018a. Regulation of pancreas development and enzymatic gene expression by duodenal infusion of leucine and phenylalanine in dairy goats. Livestock Science. 216:9–15.
- Cao YC, Yang XJ, Guo L, Zheng C, Wang DD, Cai CJ, Liu SM, Yao JH. 2018b. Effects of dietary leucine and phenylalanine on pancreas development, enzyme activity, and relative gene expression in milk-fed Holstein dairy calves. Journal of Dairy Science. 101(5):4235–4244. doi: 10.3168/jds.2017-13987.
- Carraro V, Maurin AC, Lambert-Langlais S, Averous J, Chaveroux C, Parry L, Jousse C, Ord D, Ord T, Fafournoux P, et al. 2010. Amino acid availability controls TRB3 transcription in liver through the GCN2/eIF2α/ATF4 pathway. PLoS one. 5(12):e15716. doi: 10.1371/journal.pone.0015716.
- Fingar DC, Richardson CJ, Tee AR, Cheatham L, Tsou C, Blenis J. 2004. mTOR controls cell cycle progression through its cell growth effectors S6K1 and 4E-BP1/eukaryotic translation initiation factor 4E. Molecular and Cell Biology. 24(1):200–216. doi: 10.1128/MCB.24.1.200-216.2004.
- Guo L, Liang Z, Zheng C, Liu B, Yin Q, Cao Y, Yao J. 2018a. Leucine affects α-amylase synthesis through PI3K/Akt-mTOR signaling pathways in pancreatic acinar cells of dairy calves. Journal of Agricultural and Food Chemistry. 66(20):5149–5156. doi: 10.1021/acs.jafc.8b01111.
- Guo L, Zheng C, Liang ZQ. 2018b. Progress of research on the effect of branched-chain amino acids on mammalian protein turnover and the mechanism [J]. J Agric Biotechnol. 26(006):995–1003.
- Harding HP, Zhang Y, Zeng H, Novoa I, Lu PD, Calfon M, Sadri N, Yun C, Popko B, Paules R, et al. 2003. An integrated stress response regulates amino acid metabolism and resistance to oxidative stress. Molecular Cell. 11(3):619–633. doi: 10.1016/S1097-2765(03)00105-9.
- Harmon DL. 2009. Understanding starch utilization in the small intestine of cattle. Asian-australasian Journal of Animal Science. 22:915–922. doi: 10.5713/ajas.2009.r.08.
- Harmon DL, Yamka RM, Elam NA. 2004. Factors affecting intestinal starch digestion in ruminants: a review. Canadian Journal of Animal Science. 84(3):309–318. doi: 10.4141/A03-077.
- Hashimoto N, Hara H. 2003. Dietary amino acids promote pancreatic protease synthesis at the translation stage in rats. The Journal of Nutrition. 133(10):3052–3057. doi: 10.1093/jn/133.10.3052.
- Hashimoto N, Hara H. 2004. Dietary branched-chain amino acids suppress the expression of pancreatic amylase mRNA in rats. Bioscience, Biotechnology, and Biochemistry. 68(5):1067–1072. doi: 10.1271/bbb.68.1067.
- Huntington GB, Harmon DL, Richards CJ. 2006. Sites, rates, and limits of starch digestion and glucose metabolism in growing cattle. Journal of Animal Science. 84(Suppl):E14–E24. doi: 10.2527/2006.8413_supplE14x.
- Livak KJ, Schmittgen TD. 2001. Analysis of relative gene expression data using real-time quantitative PCR and the 2(-Delta Delta C(T)) method. Methods (San Diego, Calif.). 25(4):402–408. doi: 10.1006/meth.2001.1262.
- Ren H, Bai H, Su X, Pang J, Li X, Wu S, Cao Y, Cai C, Yao J. 2020. Decreased amylolytic microbes of the hindgut and increased blood glucose implied improved starch utilization in the small intestine by feeding rumen-protected leucine in dairy calves. Journal of Dairy Science. 103(5):4218–4235. doi: 10.3168/jds.2019-17194.
- Richards CJ, Swanson KC, Paton SJ, Harmon DL, Huntington GB. 2003. Pancreatic exocrine secretion in steers infused postruminally with casein and cornstarch. Journal of Animal Science. 81(4):1051–1056. doi: 10.2527/2003.8141051x.
- Sans MD, Tashiro M, Vogel NL, Kimball SR, D'Alecy LG, Williams JA. 2006. Leucine activates pancreatic translational machinery in rats and mice through mTOR independently of CCK and insulin. The Journal of Nutrition. 136(7):1792–1799. doi: 10.1093/jn/136.7.1792.
- Schalm SS, Blenis J. 2002. Identification of a conserved motif required for mTOR signaling. Current Biology: Cb. 12(8):632–639. doi: 10.1016/S0960-9822(02)00762-5.
- Suryawan A, Orellana RA, Fiorotto ML, Davis TA. 2011. Triennial Growth Symposium: leucine acts as a nutrient signal to stimulate protein synthesis in neonatal pigs. Journal of Animal Science. 89(7):2004–2016. doi: 10.2527/jas.2010-3400.
- Swanson KC, Benson JA, Matthews JC, Harmon DL. 2004. Pancreatic exocrine secretion and plasma concentration of some gastrointestinal hormones in response to abomasal infusion of starch hydrolyzate and/or casein. Journal of Animal Science. 82(6):1781–1787. doi: 10.2527/2004.8261781x.
- Swanson KC, Matthews JC, Woods CA, Harmon DL. 2002. Postruminal administration of partially hydrolyzed starch and casein influences pancreatic alpha-amylase expression in calves. The Journal of Nutrition. 132(3):376–381. doi: 10.1093/jn/132.3.376.
- Swanson KC, Matthews JC, Woods CA, Harmon DL. 2003. Influence of substrate and/or neurohormonal mimic on in vitro pancreatic enzyme release from calves postruminally infused with partially hydrolyzed starch and/or casein. Journal of Animal Science. 81(5):1323–1331. doi: 10.2527/2003.8151323x.
- Walker JA, Harmon DL. 1996. Technical note: a simple, rapid assay for alpha-amylase in bovine pancreatic juice. Journal of Animal Science. 74(3):658–662. doi: 10.2527/1996.743658x.
- Wang X, Proud CG. 2006. The mTOR pathway in the control of protein synthesis. Physiology (Bethesda, Md.). 21:362–369.
- Wang XY. 2003. Studies on the development pattern of early digestive system in Jiangquhai meat-type line[D]. YangZhou University.(in Chinese).
- Wathes DC, Cheng Z, Chowdhury W, Fenwick MA, Fitzpatrick R, Morris DG, Patton J, Murphy JJ. 2009. Negative energy balance alters global gene expression and immune responses in the uterus of postpartum dairy cows. Physiological Genomics. 39(1):1–13. doi: 10.1152/physiolgenomics.00064.2009.
- Xu M, Du S, Wang J, Yu ZP, Harmon DL, Yao JH. 2009. Influence of rumen escape starch on pancreatic exocrine secretion of goats. Journal of Animal Physiology and Animal Nutrition. 93(1):122–129. doi: 10.1111/j.1439-0396.2007.00792.x.
- Yu ZP, Xu M, Liu K, Yao JH, Yu HX, Wang F. 2014a. Leucine markedly regulates pancreatic exocrine secretion in goats. Journal of Animal Physiology and Animal Nutrition. 98(1):169–177. doi: 10.1111/jpn.12069.
- Yu, ZP, Xu, M, Wang, F, Liu, K, Yao, JH, Wu, Z, Qin DK, Sun FF. 2014b. Effect of duodenal infusion of leucine and phenylalanine on intestinal enzyme activities and starch digestibility in goats. Livestock Science. 162:134–140. doi: 10.1016/j.livsci.2014.01.023.
- Yu ZP, Xu M, Yao JH, Liu K, Li F, Liu Y, Wang F, Sun FF, Liu NN. 2013. Regulation of pancreatic exocrine secretion in goats: differential effects of short- and long-term duodenal phenylalanine treatment. Journal of Animal Physiology and Anim Nutrition. 97(3):431–438. doi: 10.1111/j.1439-0396.2012.01276.x.