Abstract
A new occurrence of eclogites was found in the Kesandere valley in the eastern most part of the Bitlis complex, SE Anatolia. These high-pressure (HP) relics were preserved in calc-arenitic metasediments within the high-grade metamorphic basement of the Bitlis complex. The eclogitic parageneses were strongly overprinted during decompression and heating. These new eclogites locality complements the evidence of blueschist-facies metamorphism documented recently in the meta-sedimentary cover sequence of this part of the Bitlis complex. Thermodynamic calculations suggest peak conditions of ca. 480–540 °C/1.9–2.4 GPa. New U/Pb dates of 84.4 ± .9 and 82.4 ± .9 Ma were obtained on zircons from two Kesandere eclogite samples. On the basis of geochemical criteria, these dates are interpreted to represent zircon crystallization during the eclogitic peak stage. Kesandere eclogites differ from those previously described in the western Bitlis complex (Mt. Gablor locality) in terms of lithologic association, protolithic origin, and peak P–T conditions (600–650 °C/1.0–2.0 GPa, respectively). On the other hand, eclogitic metamorphism of Kesandere metasediments occurred shortly before blueschist-facies metamorphism of the sedimentary cover (79–74 Ma 40Ar/39Ar white mica). Therefore, the exhumation of Kesandere eclogites started between ca. 82 and 79 Ma, while the meta-sedimentary cover was being buried. During this short time span, Kesandere eclogite were likely uplifted from ~65 to 35 km depth, indicating a syn-subduction exhumation rate of ~4.3 mm/a. Subsequently, eclogite- and blueschist-facies rocks were likely retrogressed contemporarily during collision-type metamorphism (around 72–69 Ma). The Bitlis HP rocks thus sample a subduction zone that separated the Bitlis–Pütürge (Bistun?) block from the South-Armenian block, further north. To the south, Eocene metasediments of the Urse formation are imbricated below the Bitlis complex. They contain (post Eocene) blueschists, testifying separation from the Arabian plate and southward migration of the subduction zone. The HT overprint of Kesandere eclogites can be related to the asthenospheric flow provoked by subducting slab retreat or break off.
Introduction
As the easternmost crystalline massif of Anatolia at the northern front of the Arabian platform, the Bitlis complex occupies a key position for the paleogeographic and tectonic evolution of the Anatolian-Arabian collision belt. Was the Bitlis complex part of the Anatolian plate, or was it alternatively an individual continental block (e.g. Göncüoğlu & Turhan, Citation1997; Şengör & Yılmaz, Citation1981)? In the latter case, what is its relation with adjacent metamorphic massifs? Was the Bitlis complex buried in a subduction zone (Oberhänsli, Bousquet, Candan, & Okay, Citation2012; Oberhänsli et al., Citation2010) or not (e.g. Şengör, Citation1990; Şengör, Özeren, Genç, & Zor, Citation2003; Yılmaz, Citation1993)? During the last decade, similar long-standing, first-order questions for Western Anatolia were answered through the discovery of high-pressure (HP), low-temperature (LT) metamorphic parageneses in the Tethyan sedimentary rocks from wide areas (e.g. Candan et al., Citation2005; Gautier, Citation1984; Okay, Citation1978, Citation1980a, Citation1980b, Citation1982, Citation1984, Citation1989a, Citation1989b, Citation2002; Okay, Harris, & Kelley, Citation1998; Okay & Kelley, Citation1994; Okay & Monié, Citation1997; Okay, Monod, & Monié, Citation2002; Pourteau, Candan, & Oberhänsli, Citation2010; Rimmelé et al., Citation2003; Seaton, Whitney, Teyssier, Toraman, & Heizler, Citation2009; Whitney & Davis, Citation2006).
Eclogites are known from the central part of the Bitlis complex at Mt. Gablor (Okay, Arman, & Göncüoglu, Citation1985) south of Muş. There, kyanite eclogites occur within garnet–mica schists and gneisses. Metamorphic conditions were estimated to 600–650 °C and 1.0–2.0 GPa. A Pan-African age for the eclogitic metamorphism was assumed (Okay et al., Citation1985). Recent investigation in the sedimentary cover of the Bitlis complex defined widespread blueschist-facies metamorphism recorded by index minerals such as glaucophane and Fe, Mg carpholite in metabasic and metapelitic rocks, respectively (Oberhänsli et al., Citation2010, Citation2012). Dates of 79–74 Ma were determined from phengite by 40Ar/39Ar laser ablation methods for the peak metamorphic assemblage (1.2 GPa; 350–400 °C). Along the southern front of the eastern Bitlis complex non-metamorphic sediments of the Eocene Maden complex separate the Bitlis blueschist metasediments from metamorphic Tertiary rocks of the Urse formation. Mafic intercalations within the Urse fm. metasediments show blueschist relicts (.6 GPa; 350 °C; Oberhänsli et al., Citation2010, Citation2012). These Tertiary HP metamorphic rocks document an additional subduction realm south of the Bitlis complex separating the Bitlis Pütürge (Bistun?) block from the Arabian plate.
A new occurrence of eclogites was found in the Kesandere valley in the easternmost part of the Bitlis complex (N38°18.221′; E42°33.819′). They differ significantly from those described by Okay et al. (Citation1985) at Mt. Gablor in terms of their lithologic association and protolithic origin (Türkünal, Citation1980). The recently discovered eclogites are included in the high-grade basement rocks of the Bitlis complex. They add to the occurrences of blueschists and carpholite-, and/or chloritoid-bearing rocks of the metasedimentary sequence. Here, we describe the new eclogite locality and present detailed petrologic data and isotopic ages. Then, we compare these new findings with previous investigations from Mt. Gablor, the Menderes Massif and the HP relicts of the Bitlis sedimentary sequence (Oberhänsli et al., Citation2010, Citation2012).
Geologic setting of eclogites in the Kesandere valley
The Kesandere valley is an N–S incision in the eastern part of the basement rocks of the Bitlis complex. It leads from the Tatvan–Gevaş road southward towards Hizan. From North to South the valley cuts Mesozoic to Palaeozoic marbles, basement schists and finally basement gneisses (Figure ). The Pan-African basement consists mostly of sillimanite-bearing garnet gneiss, locally with kyanite relics (Şengün, Citation1993), and augengneiss and hornblende-gneiss of probably igneous origin (Göncüoglu & Turhan, Citation1984). In the Kesandere section (Figure ) the upper part of the basement sequence is composed of mica schists, garnet–mica schists and geisseous garnet-bearing quartzites with scarce discontinuous bands of marble and metabasites. Şengün (1993) observed an unconformity between the supposedly Precambrian basement rocks and the Palaeozoic–Mesozoic sedimentary cover. In the schists, the few mafic intercalations show amphibolite-facies overprint. The complete section is folded into an antiform. South of the gneiss unit, quartzite, marble and graphite-rich schists of a low–grade unit occur. According to Şengün (1993, his Figure 12), folding precedes metamorphism, while thrusting occurred after metamorphism. He reported a metamorphic gradient within the folded structure ranging from the chlorite- to the biotite- and finally to the garnet zone cutting the core of the fold structure. This points to the fact, that the metamorphic structure is younger than deformation depicted by the major fold.
Figure 2. (a) Cross-section in the Kesandere Valley representing the relation between high-grade and low-grade sequence and the occurrences of eclogites; (b) detailed section illustrating the occurrence of eclogitic mafic rocks associated with different metasedimentary lithotypes; (c) photograph of eclogite lenses intercalated with calc-mica schists; (d) photograph showing eclogite boudins associated with the basement gneiss.
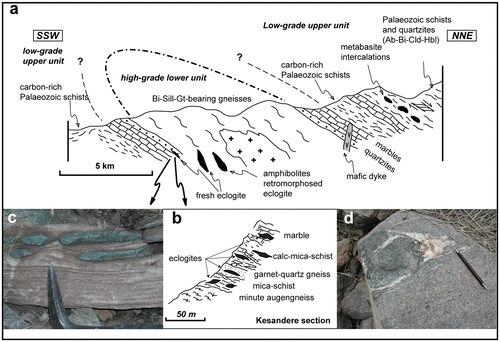
Along the valley section (NNE – SSW), we observed that mica schists grade into quartzite and dolomitic marble, possibly representing a transgressive metasedimentary sequence. Below the quartzites (Figure (a)), high-grade gneisses of sedimentary and magmatic origin occur. They are strongly folded and partly migmatized. The metapelitic gneisses are composed of biotite, garnet, sillimanite, K-feldspar, plagioclase and quartz. However, the main lithologies show more arcosic compositions that reacted to form two-mica gneisses. Intercalations of mafic material in these gneisses occur as boudins or continuous layers. Most of the mafic material is garnet amphibolite with some retrogression into epidote–chlorite amphibolites. In the central part, south of the major fold axis of the gneisses, some of the amphibolite boudins preserved eclogite parageneses. Fresh eclogite relics are found in low-strain islands in the gneisses at the limit towards the metasediments and within the metasediments (Figure (a)). These well-preserved garnet–omphacite rocks and their strongly retrogressed equivalents are new evidence for a HP metamorphic event in the Bitlis complex.
Parts of the Kesandere eclogites occur as small lenses within marble, calc-mica schist, garnet–quartz gneiss and mica schist (Figure (b) and (c)), while others occur within fine-grained augengneiss (Figure (d)). The metasedimentary section (Figure (b)) starts with mica schists, probably corresponding to a clayey weathering horizon above the basement gneiss. The garnet–quartz gneiss stems from impure clayey sandstone, which was overlain by a marly limestone sequence now represented by calc-mica schist. At the top, the sequence grades into carbonates. This section strongly resembles a transgressive sedimentary sequence. Mafic material is intercalated in quartz-rich pelite, marl and limestone.
Along the section (Figure (b)) the three lithologies, micaschists, calcschists and marbles, vary in proportion. The base of the section comprises ca. 70 m of pelitic quartzite to quartz-rich metapelite mostly composed of quartz, biotite and garnet. Then, ca. 50 m of calcschist comprising quartz, white mica, zoisite/epidote, garnet and calcite occur. The top of the metasedimentary section is made up by ca. 80 m of white to grey marbles. Disrupted but concordant mafic layers, possibly tuffaceous in origin, may still be recognized. The mafic rocks, are metamorphosed into eclogite, made up by garnet and omphacite. Actually eclogitic assemblages are observed only in low-strain islands, which were not affected by intense, syn-exhumation deformation. In total, the metasedimentary complex builds up a section of ca. 200 m thickness. It is intercalated with augengneiss.
Methods
In selected samples electron microprobe analyses were carried out using a JEOL JXA-8200 probe, equipped with five WDS spectrometers and an EDS spectrometer, at the Institute of Earth and Environmental Sciences of the University of Potsdam. The analytical conditions were: 15 kV accelerating voltage and 10 nA beam current with counting times of 20 s at peak and 10 s at background. Natural oxides and minerals were used as standards. Detection limits vary with atomic number and range between 50 and 100 ppm.
For U–Pb dating we used zircons from two eclogite samples were extracted using standard magnetic techniques and heavy liquids, handpicked under a binocular microscope, and then were embedded in epoxy resin. The samples were polished to approximately half thickness to reach up to the centre of the zircon grains. Transmitted and reflected light images were taken to document cracks and inclusions in zircons, and the shape of zircon grains and their position in the mount. The internal structures of zircons were documented by cathodoluminescence (CL) using a CAMECA SX51 instrument at conditions of 15 kV and 120 nA in Institute of Geology and Geophysics, Chinese Academy of Sciences, Beijing.
Zircon U–Pb geochronology and trace element analyses of samples B157-1 and B157-2 were performed using Laser Ablation Inductively Coupled Plasma Mass Spectrometry (LA-ICP-MS) at University of Science and Technology of China in Hefei. A pulsed 193 nm ArF Excimer laser system with 10 J/cm2 beam energy at a repetition ratio of 10 Hz coupled to a PerkinElmer Elan DRCII quadrupole ICP-MS was used for ablation. Spot size generally was 60 μm in diameter, sometimes 44 μm where necessary. NIST610 was used as an external standard to normalize U, Th and Pb contents on unknowns. Raw data were processed using the LaTecalc program. Common Pb correction was applied using the method of Andersen (Citation2002). Uncertainties of individual analyses are reported at 1σ; weighted average ages are calculated at 2σ level and uncertainties in ages are reported at the 95% confidence level. For detailed analytical procedure see Yuan et al. (Citation2004). Standard zircon 91500 was analyzed to calibrate the mass discrimination and elemental fractionation, the U/Pb ratios were processed using a macro program LaDating@Zrn written in Excel spread sheet software. Age calculations were carried out using the Isoplot/Ex 3.0 program (Ludwig, Citation2003).
Kesandere eclogites
Petrography
Basement rocks
The metapelitic gneisses (Figure (a)) typically consist of quartz, K-feldspar, plagioclase, garnet, biotite, white mica and amphibole. Sillimanite and kyanite occur as minor components. The lithologies of the country rocks containing the eclogitic boudins do not show HP index minerals.
In mafic lithologies included in the basement gneisses, HP metamorphism produced zoisite – phengitic mica – garnet ± omphacite assemblages. However, even the best preserved eclogitic rocks (Figure (A)) contain retrograde biotite, symplectitic domains and keliphitic rims around garnet, as well as amphibole replacing omphacite, which document a later barrovian-type high-temperature, medium-pressure overprint. Finally, various retrograde stages led to the formation of amphibole–biotite coronas on garnet and a matrix of amphibole–plagioclase symplectites containing large elongate epidote/clinozoisite and sphene crystals (Figure (C) and (D)). Clinozoisite formed in pressure shadows of garnet pseudomorphs, and is wrapping around the garnet pseudomorphs, clearly showing that they formed earlier than the amphibole–plagioclase symplectites.
Figure 3. Photomicrographs of (A) eclogite texture with omphacite and garnet, + nic., width 7 mm, sample Van 95; (B) garnet and omphacite, pl. width 3 mm, sample Van 95; (C) garnet with symplectite of retrogressed omphacite, sample Van 100; (D) biotite corona around garnet pl. l., width 5 mm, sample Van 98.
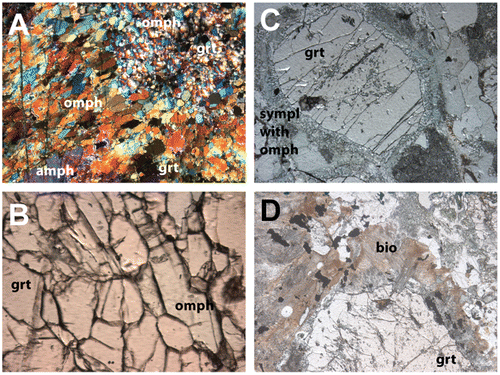
Cover rocks
In the metasedimentary part of the section, low-strain islands comprise fresh eclogites with typical garnet–omphacite (Figure (C)). Rarely white mica is found in textural parageneses. In deformed parts most mafic rocks are overprinted and comprise conspicuous biotite. The typical assemblage is biotite–hornblende–epidote/zoisite.
Mineral chemistry
In eclogitic samples from the metasedimentary cover sequence, garnet, clinopyroxene and white mica were analyzed in the freshest parts. Garnet (Table ) is not zoned but varies in different bulk rocks. It is almandine-rich (42–56 Mol.%) and contains moderate to high pyrope- (16–28 Mol.%) and grossular (17–31 Mol.%) components. In most samples, unzoned clinopyroxene (Table ) corresponds to omphacite with 11–21% of the endmember jadeite, while omphacite of one sample still preserves Jd48–59. White mica is muscovite-rich with moderate paragonite and celadonite components (Si ≈ 3.2 pfu). Its composition is not typical for HP conditions and we assume that white mica recrystallized during decompressional heating as indicated by biotite coronas (Figure (D)). Amphiboles (Table ) show pargasitic to edenitic as well as actinolithic (ferroan-tremolitic) compositions. Epidote is rich in the clinozoisite end-member with up to 2.5 pfu of Al. Biotite generally exhibits low K2O contents (6.6–8.7 wt.%) indicating variable degrees of chloritisation.
Table 1. Representative electron micro-probe analyses of garnets.
Table 2. Representative electron micro-probe analyses of pyroxenes.
Table 3. Representative electron micro-probe analyses of amphiboles.
Petrology
Metamorphic P–T conditions of the basaltic eclogites found among metasedimentary rocks have been estimated using of classical exchange thermo- and barometers and phase diagram calculations using the JUN92.bs database file and THERIAK-DOMINO software package (De Capitani & Brown, Citation1987; De Capitani & Petrakakis, Citation2010).
Applying the classical geothermobarometric tools (Carswell & Harley, Citation1990; Krogh, Citation2000; Newton & Smith, Citation1967; Råheim & Green, Citation1974) on garnet–clinopyroxene pairs of well-preserved eclogite samples (Van 95), provide pressures ranging from 1.9 to 2.4 GPa and temperatures spanning 480–540 °C (Figure ). These P–T conditions are somewhat colder that those estimated by Okay et al. (Citation1985; 1.0–2.0 GPa/600–650 °C) for metagabbroic eclogites of Mt. Gablor, further to the west of the Bitlis complex. Using the compositions of garnet rims and clinopyroxenes from the simplectitic rims in retrogressed samples (Van 100), distinctively higher temperatures, up to 600 possibly 650 °C, are obtained using, the same methods. Similar temperatures are calculated using garnet and biotite for an other, biotite-bearing retrogressed eclogite sample (Van 98) (Bhattacharya, Mohanty, Maji, Sen, & Raith, Citation1992; Perchuk & Lavrent’eva, Citation1983) though again pressures remain badly constrained.
Figure 4. P–T estimates for Kesandere eclogites using geothermobarometers (Bhattacharya et al., Citation1992; Krogh, Citation2000; Perchuk & Lavrent’eva, Citation1983; Råheim & Green, Citation1974) and comparison with values published for eclogites from Mt. Gablor (Okay et al., Citation1985) in the western Bitlis complex.
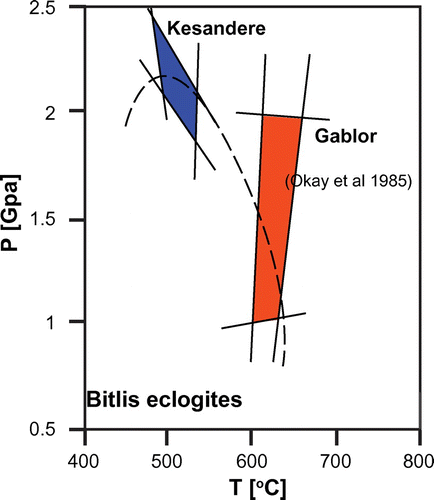
One well-preserved eclogite sample was used for thermodynamic modeling. Its molar bulk composition, determined by XRF, is given in Figure . Since eclogites are preserved in low strain islands, closed chemical system conditions were assumed. As a result, we reproduced quite adequate observed mineral assemblages and phase compositions for 2 GPa and 520 °C (Figure , red pentagon) using THERIAK (op cit.). The corresponding petrogenetic grid produced with DOMINO (op cit.) shows phase distribution and fits quite well with our petrographic observations. A small field remains for the stability of the observed biotite-free, omphacite–garnet–phengite paragenesis. P–T conditions for this domain fit with the conditions deduced with geothermobarometric methods. The phase diagram shows a large stability field for biotite. Biotite- and amphibolite-bearing parageneses, observed in similar but more retrogressed rocks, are predicted for any kind of retrograde evolution, (either isothermal decompression or syn-decompression heating), which may be envisaged for the eclogite exhumation. The absence of phengite and the little amount of chlorite in the retrogressed eclogites suggest syn-decompression heating out of white-mica stability field, and into chlorite stability field at a late retrograde stage only.
Figure 5. Petrogenetic grid calculated with THERIAK and DOMINO (De Capitani & Brown, Citation1987; De Capitani & Petrakakis, Citation2010) for the rock composition: Si(47.41)–Al(10.36)–Fe(7.19)–Mg(14.48)–Ca(13.39)–Na(6.85)–K(.11)–O(?)–H(30). The red pentagon indicates P–T conditions at which the THERIAK software reproduced the mineral compositions measured in this rock as close as possible. The colored fields depict the stability domain of the major phases in the rock. The exhumation path with question marks is inferred from the observed mineral assemblages (see Text).
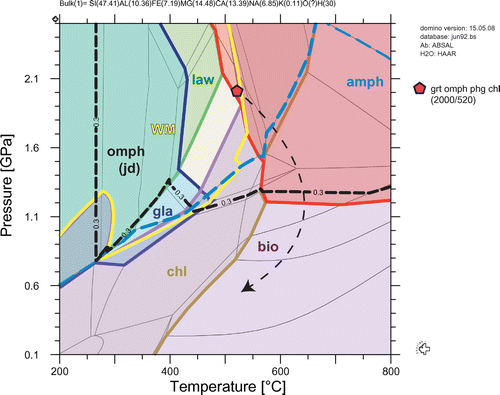
Zircon U/Pb geochronology and trace element composition
Both dated samples are eclogite, and were collected SW of Tatvan – Kesandere. They provide from the same rocks, in which the petrology has been studied.
Sample B157-1 contains abundant clear, colourless, euhedral to subhedral, irregular sized zircon grains of 100–200 µm with aspect ratios of 1:1.5–1:3. Short- to rarely long-prismatic zircon grains have pitted surfaces. CL images of this sample reveal that some zircon grains contain CL-bright and structure less cores surrounded by a narrow or rarely wide outer CL-grey overgrowth (Figure ; gr 11 and 12). The remaining cores and surrounding overgrowths show weak oscillatory zoning. Most of the zircons show oscillatory zoning with narrow or wide bands from centre to rim (Figure ; gr 14, 16, 21 and 23). They display inhomogeneous (grey or bright) CL intensities. A few zircons have diffuse sector zoning (Figure ; gr 24).
Figure 6. CL images of selected zircons in sample B-157-1 and B-157-2 showing LA-ICP-MS analysis spots (circles) and 206Pb/238U ages. The dashed circles represent measurements with imponderable data from spots on the rims. The squares on zircons show areas of trace element analyze points.
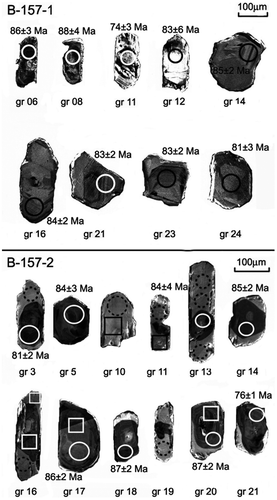
A total of 33 spots were analyzed on 28 grains. Only 19 spots from core and zircon grains with oscillatory zoning provided reproducible results, whereas the 14 other spot analyses along rims and within overgrowths did not deliver reliable ages. Although data slightly scatter along the concordia, while 18 analyses cluster on the concordia, the scattered values do not reflect another age group. Sixteen analyses from oscillatory zircon cores yield a concordia age of 82.4 ± .9 Ma, combining to mean 206Pb/238U and 207Pb/235U ages of 82.6 ± 2.1 Ma and 82.9 ± 1.2 Ma, respectively (Table and Figure (a)). We conclude that the concordia age of 82.4 ± .9 Ma reflects the timing of zircon crystallization, most likely during eclogitic metamorphic stage.
Table 4. LA-ICP-MS U–Pb analytical data and calculated ages for zircons from eclogite samples, B-157-1 and B-157-2.
Figure 7. Condordia diagrams showing U/Pb isotope ratios and ages derived from LA-ICP-MS analyses for eclogite samples B-157-1 (a) and B-157-2 (b).

Sample B157-2 was collected within 100 m of sample B157-1. The zircons from this sample comprise abundant clear, colourless, light pink, euhedral to subhedral, irregular sized 100–250 µm long and short- to long-prismatic grains with aspect ratios of 1:1–1:4. A few zircons show probably inherited cores, most probably derived from the country rocks, indicating a possibly tuffaceous nature of the eclogite layers. In particular some large and rounded grains show fir-tree zoning (Figure ; gr 17). However, CL images reveal that many zircon crystals contain CL-dark cores with weak igneous oscillatory zoning (Figure ; gr 3, 13, 14, 16, 20 and 21). Most of these cores are surrounded by narrow to wide, irregular, structure less or very weak zoned CL-bright rims, which were formed by recrystallization during exhumation and retrograde metamorphism.
Oscillatory zoning in cores representing preserved relict textures of the eclogites is generally cut by the rims, which is typical for recrystallized zircons (Hoskin & Schaltegger, Citation2003; Pidgeon, Nemchin, & Hitchen, Citation1998; Wu & Zheng, Citation2004). Resorption, which results in recrystallization towards the core is common and affects a majority of the zircon population in this sample. The boundaries between the recrystallized and possibly (?) magmatic domains are curved (Figure (b); gr 3, 11, 13, 16, 19, 20 and 21). Recrystallization of zircons developed mostly at the crystal terminations. Recrystallized zircon grains keep their original magmatic (?) crystal shapes; therefore, these zircons can be distinguished from overgrowth zircon types by their morphology and internal structure (Wu & Zheng, Citation2004).
A total of 31 spots on 21 grains were analyzed, but only 14 spots on cores delivered reasonable results while 14 spot analyses from rims failed. These fourteen analyses from magmatic zircons and cores cluster on the concordia and define a concordia age of 84.4 ± .9 Ma corresponding to a mean 206Pb/238U and 207Pb/235U ages of 84.5 ± 1.5 Ma and 81.2 ± 3.1 Ma, respectively (Table and Figure (b)). Therefore, we propose that the concordia age of 84.4 ± .9 Ma represents the zircon crystallization age during prograde metamorphism.
Trace element composition
Ten zircon grains, five from cores and five from rims, were analyzed for trace elements. The magmatic cores with typical oscillatory zoning texture have low Th (1.6–32 ppm) and moderate U (79–259 ppm) contents with a Th/U ratio of .008–.026 with the exception of one sample (10*) that shows a Th/U ratio of .4 (Table ). Such ratios are typical for metamorphic zircons, although Vavra, Schmid, and Gebauer (Citation1999), Rubatto (Citation2002), Möller, O’Brien, Kennedy, and Kröner (Citation2003) and Harley, Kelly, and Möller (Citation2007) suggested that the Th/U ratio could not be used to discriminate between magmatic and metamorphic zircon. The distinct positive Ce anomaly (Figure ) claims for a metamorphic origin of the zircons (Hoskin & Schaltegger, Citation2003; Rubatto, Citation2002).
Figure 8. Chondrite-normalized REE pattern for zircons of sample B-157-2. Normalized values are from Sun and McDonough (Citation1989).
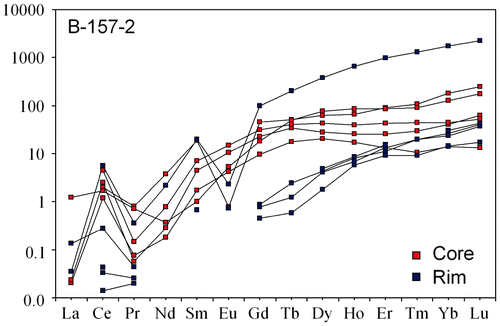
Whether the oscillatory zoning might be a magmatic relict texture or product of crystallization of eclogitization is widely discussed in literature. In some studies, the Th/U ratio of zircon is used for the discrimination of their possible origin, and values of ca. 1.0 (Wu & Zheng, Citation2004), ≥.5 (Hoskin & Schaltegger, Citation2003) are suggested for magmatic zircon while the Th/U ratio is less than .1 in metamorphic zircon (Wu & Zheng, Citation2004).
The cores in sample B157-2 have relatively high total REE content (Table and Figure ) with flat HREE slopes. The chondrite normalized REE patterns of these zircons have a positive Ce anomaly. Except for zircon grain 1c, they do not show a significant negative Eu anomaly. This pattern could be typical for zircons, which crystallized at eclogite facies conditions, where feldspar was unstable (Rubatto, Citation2002). Since the basaltic protoliths of eclogites generally contain significant amounts of feldspar, a magmatic origin from feldspar poor and/or completely feldspar-free rocks, characteristic for a magmatic origin seems unlikely.
Table 5. Trace element composition of zircon analyzed by LA-ICP-MS for eclogite sample B-157-2.
The zircons crystallized during the HP metamorphism. The rims, which formed during HT overprint, differ from cores by their very low Th (.02–.33 ppm), very low U contents (1.9–13.5 ppm) and lower Th/U ratios (.01–.12) except for grain 4r. Hence, the rims of the zircons do not give any reasonable ages. The zircon rims of this sample are characterized by nearly flat LREE patterns and slightly steep HREE patterns, which are typical for partial solid-state recrystallization (Hoskin & Ireland, Citation2000). It also shows that HREE were expelled from zircon during sub-solidus recrystallization (Hoskin & Black, Citation2000). In eclogite facies rocks, rutile is strongly enriched in Ta and Nb and has very high Nb/Ta ratio (Rudnick, Barth, & Horn, Citation2000; Wu & Zheng, Citation2004). Nb/Ta ratio (4–12 ppm) of the recrystallized rims in our sample is lower than magmatic protolith cores (10–39 ppm) and suggests the existence of rutile during the recrystallization of the rims of zircons after eclogite-facies conditions.
Discussion of age data
In both samples, separated zircons contain distinct cores and rims. In sample B157-1, only zircon cores give 82 Ma ages, while rims recrystallizing during decompressional heating did not allow calculating any meaningful age. Zircons of sample 157-2 show the same textural features. Cores with oscillatory zoning gave 84 Ma but no age could be determined from the bright, unzoned rims. The fact that no reliable age data was retrievable from the rims needs further investigations. Our data reveal that the Kesandere eclogites in the western Bitlis Massif formed during the middle Late Cretaceous, whereas a Pan-African age was previously assumed for the Mt. Gablor, W Bitlis Massif (Okay et al., Citation1985).
Eclogites and associated rocks occur within the Pan-African high-grade basement, which is cut by granitoids (e.g. 545 Ma – old Mutki granite; Ustaömer, Ustaömer, Collins, & Robertson, Citation2008; M in Figure ). However, these HT metamorphic country rocks do not contain evidence of HP metamorphism. Kesandere eclogites and coexisting marble, quartzite and metapelite, although mapped as unconformably overlying the basement (Şengün, Citation1983), most likely belong to a tectonic slice within the HT series.
When combined with the Late Cretaceous HP metamorphism characterized by carpholite and blueschists occurrences (Oberhänsli et al., Citation2010, Citation2012), it can be envisaged that eclogite-facies metamorphism (younger than 82–84 Ma) should be related to the closure of this Neothethyan oceanic domain.
Comparison to other Anatolian HP units
Eclogite boudins occurring within high-grade gneiss units are reported from the Menderes massif (Candan et al., Citation2001; Oberhänsli, Candan, Dora, & Dürr, Citation1997). However, the fact that in the Kesandere Valley eclogites are found incorporated in high-grade basement rocks and in a metasedimentary sequence marks a major difference as compared to the eclogite occurrences at Mt. Gablor (western Bitlis complex) or in the Menderes Massif (Western Anatolia). There eclogites are associated with augengneiss and their protoliths were mainly gabbros (Candan et al., Citation2001; Oberhänsli, Candan, & Wilke, Citation2010; Okay et al., Citation1985; Oberhänsli et al., Citation1997; Türkünal, Citation1980).
In the Menderes Massif, augengneiss are coarse-grained alkali feldspar gneisses with typical HT assemblages. They contain sillimanite and garnet. These basement gneisses comprise a comparatively high amount of garnet amphibolite lenses, which sometimes preserved their gabbroic textures and precursor lithology. In large mafic bodies, eclogites can be found along shear bands that cut the coronitic high-grade metagabbros. Retrograde metamorphism and associated deformation transformed these mafic rocks to garnet amphibolites. Eclogites deriving from basaltic protoliths (e.g. Kiraz) also occur within the augengneiss basement. U/Pb zircon data revealed a Pan-African intrusion age (540 Ma) of the metagabbros and shortly later eclogite-facies metamorphic overprint at 530 Ma (Oberhänsli, Candan, et al., Citation2010).
Protoliths of the kyanite eclogites at Mt. Gablor were interpreted as Pan African in age (Okay et al., Citation1985), i.e. similar to the Menderes eclogites. In its original report, Türkünal (Citation1980) observed that eclogites are associated with albite-rich augengneiss. He interpreted the eclogites as ouralitized metagabbros. Again this bears similarity to the Menderes massif, where eclogites also show gabbroic protoliths (Candan et al., Citation2001), but contrasts to the Kesandere eclogite locality, which consists mainly of a calc-arenitic metasedimentary sequence.
In consequence, while our new eclogite age of 84–82 Ma for the Kesandere eclogites is consistent with the 79–74 Ma age of the blueschist-facies metamorphism in the Bitlis metasedimentary cover (Oberhänsli et al., Citation2012), it contrasts with the demonstrated Pan-African age of the Menderes eclogites, as well as the inferred Pan-African age for Mt Gablor eclogite in the western Bitlis complex. Whereas, in the Menderes, eclogitization (530 Ma) followed granulitization and the crystallization of the gabbroic protolith (530 Ma; Oberhänsli, Candan, et al., Citation2010), eclogite and associated high-grade rocks in Kesandere followed a classical clock-wise P–T evolution during continental subduction (ca. 84–74) then collision (70–67 Ma). Ages to the latter range were recently obtained from Barrovian-type metamorphic rocks of the Pütürge massif (74–70 Ma; 39Ar/40Ar white-mica; Rolland et al., Citation2012), which was long regarded as the western equivalent of the Bitlis complex (Yılmaz, Citation1993; Yılmaz, Yığıbaş, & Genç, Citation1993) but did not experience HP–LT conditions. We, therefore, infer that during the accretion of the Pütürge–Bitlis block to the trench, solely the eastern sector entered the subduction zone, whereas the entire terrane later experienced collision-related metamorphism.
Moreover, according to available data, the subduction-relation metamorphic evolution of the Bitlis complex is strikingly coeval with the HP metamorphism in the Tavşanlı Zone, NW Anatolia (88–80 Ma Rb–Sr and Ar–Ar phengite dates; Seaton et al., Citation2009; Sherlock, Kelley, Inger, Harris, & Okay, Citation1999). However, these two domains fundamentally differs from each other on the following aspects: (a) The Tavşanlı Zone was buried into a very cold subduction zone (Okay, Citation2002; Whitney & Davis, Citation2006), whereas peak metamorphic conditions were relatively “warmer” for the Bitlis complex; (b) the HP metamorphic rocks of the Bitlis complex were strongly overprinted by collision-type metamorphism, whereas the Tavşanlı Zone HP rocks were cooled down during their exhumation, as indicated by widespread pristine lawsonite; (c) the Bitlis complex represents a continental passive margin fragment, including neritic sedimentary cover and substratum, whereas the Tavşanlı Zone consists mainly of metamorphosed mafic and pelagic sedimentary rocks (Okay, Citation1984) and (d) the evolution of the Bitlis complex is related to the closure of a oceanic domain once located between the Arabian continental to the south, and the Anatolide–Tauride block to the north, whereas the Tavşanlı Zone stems from the northern edge of the later related then to the closure of another oceanic realm further north (Barrier & Vrielynck, Citation2008; Okay & Tüysüz, Citation1999; Şengör & Yılmaz, Citation1981), and likely are connected eastwards to the Amassia–Stepanevan blueschists (Rolland, Billo, Corsini, Sosson, & Galoyan, Citation2008).
Discussion and conclusions
The eclogites we report from the eastern Bitlis complex occur in association of calcschists and former oceanic sediments. Thus, tectonic incorporation into the high-grade basement of the Bitlis complex must be assumed. P–T estimates for the eclogites are around 2 GPa and 520 °C, pointing to a burial depth of ca. 65 km, while the blueschists from the sedimentary cover sequence were subducted to ca. 35 km depth only (ca. 1.2 GPa and 350–400 °C; Oberhänsli et al., Citation2010). Petrological studies and PT estimates indicate that Kesandere eclogites were affected by a considerable heating phase during their exhumation. On the base of mineral trace element compositions, the age of 84.4 ± .9 Ma is interpreted to represent the crystallization age of zircon, while the age of 82.4 ± .9 Ma is interpreted as the time of crystallization of the eclogite paragenesis. 39Ar/40Ar in situ laser ablation age determination (Oberhänsli et al., Citation2012) yielded 79–74 Ma for the peak metamorphic assemblage Fe–Mg–carpholite–chlorite–phengite and 74–71 Ma for the retrograde assemblage chloritoid–chlorite–phengite–kyanite from metasedimentary cover rocks of the Bitlis complex. This leaves a short time span for the exhumation of the eclogites from ca. 65 to 35 km depth that can be interpreted as exhumation rate of ca. 4.3 mm/a. The new data clearly show that eclogite and blueschist metamorphic peak conditions were reached at different times within the subduction complex, suggesting coeval exhumation, like in Western Anatolia (Pourteau et al., Citation2013).
The retrograde evolution of Kesandere eclogites strongly contrasts with the isothermal decompression experienced by the carpholite-, chloritoid, and glaucophane, bearing i.e. lower-grade, HP metasedimentary cover (Oberhänsli et al., Citation2010). This implies a twofold evolution: the eclogite phase recorded in the basement was followed by a barrovian-type overprint in amphibolite-facies conditions. Contrarily, the widely distributed and well-preserved blueschist assemblages in the metasedimentary cover were affected by low to moderate retrograde heating under greenschist-facies conditions. The different HP rocks sampled different depth compartments of a subduction complex (Figure (A)) and recorded different exhumation conditions. Continental collision and/or slab break-off eventually led to the HT overprint during exhumation (Figure (B)). South of the Bitlis complex Tertiary blueschists (Oberhänsli et al., Citation2010) testify a significant separation from the Arabian plate, and therefore an additional subduction zone (Figure (C)). Given the differing intensities of collisional-type metamorphic overprint, the eclogite, and low-grade, HP units were juxtaposed in the upper crust during late collisional stages, when the Bitlis complex (Bistun block?) was amalgamated with the South Armenian block. Subduction as well as exhumation processes ran not co-linear but with oblique motions leading to the present day position of the Pütürge complex, to the west, that did not experience Cretaceous subduction events and the Bitlis complex, to the east, with its HP imprint (Figure (D)).
Figure 9. Sketch of cross sections for Eastern Anatolia from Late Cretaceous to Oligo–Miocene. (A) Late Cretaceous–Paleocene: the northern part of the Bistun (?) bock is in subduction, recording different stages of eclogite (Kesandere, Gablor) and blueschist facies (metasedimentary cover) in the Bitlis complex while the southern Pütürge complex remains unaffected. (B) Paleocene–Eocene: slab break off of the Bitlis subduction lithosphere leads to barrovian HT overprint of the eclogites and arc volcanism in the Maden complex. (C) Late Eocene–Oligocene: on going subduction south of the Bitlis–Pütürge complex produced glaucophane in the Urse Formation. (D) Oligo–Miocene: oblique Tethyan (clockwise) exhumation led to the juxtaposition of the Pütürge complex without HP to the west and the HP Bitlis complex to the east. The Maden complex, overlying the Pütürge basement conformably, separates the two parts of the Bistun (?) block while it is tectonically imbricated in the southern front of the Bitlis complex.
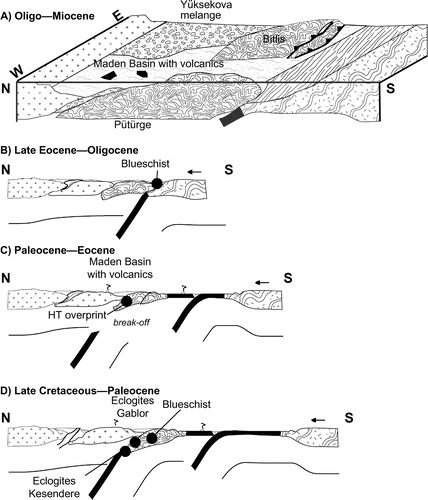
Our isotopic age data contrast with the Pan-African ages that were (i) assumed for the eclogites at Mt. Gablor (western Bitlis complex) and (ii) demonstrated for the Menderes Massif eclogites (530 Ma, Oberhänsli, Candan, et al., Citation2010).
Besides, our petrologic investigations lead to the conclusion that the P–T evolution of eclogites from the two massifs diverged in the sense that the Menderes massif underwent a high-grade granulitic phase before Pan-African eclogitisation, while the Kesandere samples clearly indicate that the Late Cretaceous eclogites of the Bitlis complex experienced a classical subduction-to-collision, clockwise P–T loop with a later decompressional heating stage, related to asthenospheric flow during slab break off following collision and ongoing subduction south of the Bitlis–Pütürge complex (Figure ).
Acknowledgements
Our investigations in the Bitlis complex were part of the MEBE and DARIUS Program. We thank M. F. Brunet, E. Barrier and J. P. Cadet (MEBE and DARIUS team in Paris) for their technical and financial support. We thank our two colleagues Donna Whitney and Gültekin Topuz for critically reviewing this paper.
References
- Andersen,T. (2002).Correction of common lead in U–Pb analyses that do not report 204Pb.Chemical Geology,192,59–79.10.1016/S0009-2541(02)00195-X
- Barrier, E., & Vrielynck, B. (2008). Palaeotectonic maps of the Middle East tectono-sedimentary-palinspastic maps from Late Norian to Piacenzia. Retrieved from Commission for the Geological Map of the World (CGMW/CCGM)/UNESCO, http://www.ccgm.org, Atlas of 14 maps, scale 1/18,500,000.
- Bhattacharya,A.,Mohanty,L.,Maji,A.,Sen,S. K., &Raith,M. (1992).Non-ideal mixing in the phlogopite-annite binary: Constraints from experimental data on Mg–Fe partitioning and a reformulation of the biotite-garnet geothermometer.Contributions to Mineralogy and Petrology,111,87–93.10.1007/BF00296580
- Candan, O., Çetinkaplan, M., Oberhänsli, R., Rimmelé, G., & Akal, C. (2005).Alpine high-P/low-T metamorphism of the Afyon Zone and implications for the metamorphic evolution of Western Anatolia, Turkey. Lithos,84,102–124.
- Candan,O.,Dora,O. Ö.,Oberhänsli,R.,Çetinkaplan,M.,Partzsch,J. H.,Warkus,F. C., &Dürr,S. (2001).Pan-African high-pressure metamorphism in the Precambrian basement of the Menderes Massif, western Anatolia, Turkey.International Journal of Earth Science,89,793–807.
- Carswell,D. A., &Harley,S. L. (1990).Mineral barometry and thermometry. InD. A.Carswell (Ed.),Eclogite facies rocks (pp.83–110).New YorkNY:Blackie.10.1007/978-94-010-9263-0
- De Capitani,C., &Brown,T. H. (1987).The computation of chemical equilibrium in complex systems containing non-ideal solutions.Geochimica et Cosmochimica Acta,51,2639–2652. doi:10.1016/0016-7037(87)90145-110.1016/0016-7037(87)90145-1
- De Capitani,C., &Petrakakis,K. (2010).The computation of equilibrium assemblage diagrams with Theriak/Domino software.American Mineralogist,95,1006–1016. doi:10.2138/am.2010.335410.2138/am.2010.3354
- Gautier,Y. (1984).Deformations et métamorphisme associés à la suture téthysienne en Anatolie Centrale (Région de Sivrihisar, Turquie) [Deformation and metamorphism related to the Tethyan suture of Central Anatolia (Sivrihisar, Turkey)]. ( PhD thesis).Paris-Sud Orsay, p.236.
- Göncüoglu,M. C., &Turhan,N. (1984).Geology of the Bitlis metamorphic belt. InO.Tekeli &M. C.Göncüoglu (Eds.),Geology of the Taurus Belt.Proceedings of the International Symposium on the Geology of the Taurus Belt, 26–29 September 1983 (pp.237–244).Ankara: Mineral Research and Exploration Institute of Turkey (MTA).
- Göncüoğlu,M. C., &Turhan,N. (1997).Rock units and metamorphism of the basement and Lower Paleozoic cover of the Bitlis Metamorphic Complex, SE Turkey. InM. C.Göncüoğlu &A. S.Derman (Eds.),Lower Paleozoic evolution in northwest Gondwana (Vol.3, pp.75–81).Ankara:Turkish Association of Petroleum Geology, Special Publications.
- Harley,S. L.,Kelly,N. M., &Möller,A. (2007).Zircon behaviour and the thermal histories of mountain chains.Elements,3,25–30.10.2113/gselements.3.1.25
- Hoskin,P. W. O., &Black,L. P. (2000).Metamorphic zircon formation by solid-state recrystallization of protolith igneous zircon.Journal of Metamorphic Geology,18,423–439.
- Hoskin,P. W. O., &Ireland,T. R. (2000).Rare earth element chemistry of zircon and its use as a provenance indicator.Geology,28,627–630.10.1130/0091-7613(2000)28<627:REECOZ>2.0.CO;2
- Hoskin,P. W. O., &Schaltegger,U. (2003).The composition of zircon and igneous and metamorphic petrogenesis. InJ. M.Hanchar &P. W. O.Hoskin (Eds.),Zircon. Reviews in mineralogy and geochemistry (Vol.53, pp.27–62). Washington, DC:Mineralogical Society of America.
- Krogh,R. E. (2000).The garnet-clinopyroxene Fe2+–Mg geothermometer: An updated calibration.Journal of Metamorphic Geology,18,211–219.10.1046/j.1525-1314.2000.00247.x
- Ludwig,K. R. (2003).User’s manual for Isoplot 3.0: A geochronological toolkit for Microsoft Excel. Berkeley, CA:Berkeley Geochronology Center, Special Publication,Vol. 4, p.70.
- Möller,A.,O’Brien,J. P.,Kennedy,A., &Kröner,A. (2003).Linking growth episodes of zircon and metamorphic textures to zircon chemistry: An example from the ultrahigh-temperature granulites of Rogaland (SW Norway). InD.Vance,W.Müller, &I. M.Villa (Eds.),Geochronology: Linking the isotopic record with petrology and textures (Vol. 220, pp.65–81).London:Geological Society, Special Publications.
- Newton,R., &Smith,J. (1967).Investigations concerning the breakdown of albite at depth in the earth.Journal of Geology,75,268–286.10.1086/jg.1967.75.issue-3
- Oberhänsli,R.,Bousquet,R.,Candan,O., &Okay,A. I. (2012).Dating subduction events in East Anatolia.Turkish Journal of Earth Sciences,21,1–18. doi:10.3906/yer-1006-26
- Oberhänsli,R.,Candan,O.,Bousquet,R.,Rimmelé,G.,Okay,A., &Goff,J. (2010).Alpine HP evolution of the eastern Bitlis complex, SE Turkey. InM.Sosson,N.Kaymakci,R.Stephenson,V.Strarostenko, &F.Bergerat (Eds.),Sedimentary basins, tectonics from Black Sea and Caucasus to the Arabian platform (Vol. 340, pp.461–483).London:Geological Society, Special Publications.
- Oberhänsli,R.,Candan,O.,Dora,O. Ö., &Dürr,St (1997).Eclogites within the Menderes Massif/western Turkey.Lithos,41,135–150.10.1016/S0024-4937(97)82009-9
- Oberhänsli,R.,Candan,O., &Wilke,F. (2010).Geochronological evidence of Pan-African eclogites from the Central Menderes Massif, Turkey.Turkish Journal of Earth Sciences,19,431–447. doi:10.3906/yer-1001-20
- Okay,A. (1978).Sodic pyroxenes from metabasites in the Eastern Mediterranean.Contributions to Mineralogy and Petrology,68,7–11.10.1007/BF00375442
- Okay,A. (1980a).Mineralogy, petrology and phase relations of glaucophane-lawsonite zone blueschists from the Tavsanly region, northwest Turkey.Contributions to Mineralogy and Petrology,72,243–255.10.1007/BF00376143
- Okay,A. (1980b).Lawsonite zone blueschists and a sodic amphibole producing reaction in the Tavşanlı Region, northwest Turkey.Contributions to Mineralogy and Petrology,75,179–186.
- Okay,A. (1982).Incipient blueschist metamorphism and metasomatism in the Tavşanlı Region, northwest Turkey.Contributions to Mineralogy and Petrology,79,361–367.10.1007/BF01132065
- Okay,A. (1984).Distribution and characteristics of the northwest Turkish blueschists. InJ. E.Dixon &A. H. F.Robertson (Eds.),The geological evolution of the Eastern Mediterranean (Vol. 17, pp.455–466).London:Geological Society, Special Publication.
- Okay,A. (1989a).An exotic eclogite/blueschist slice in a Barrovian-style metamorphic terrain, Alanya Nappes, southern Turkey.Journal of Petrology,30,107–132.10.1093/petrology/30.1.107
- Okay,A. (1989b).Alpine-Himalayan blueschists.Annual Review of Earth and Planetary Sciences,17,55–87.10.1146/annurev.ea.17.050189.000415
- Okay,A. (2002).Jadeite-chloritoid-glaucophane-lawsonite blueschists in North-West Turkey: Unusually high P/T ratios in continental crust.Journal of Metamorphic Geology,20,757–768.10.1046/j.1525-1314.2002.00402.x
- Okay,A.,Arman,M. B., &Göncüoglu,M. C. (1985).Petrology and phase relations of the kyanite-eclogites from Eastern Turkey.Contributions to Mineralogy and Petrology,91,196–204.10.1007/BF00377767
- Okay,A.,Harris,N. B. W., &Kelley,S. P. (1998).Blueschist exhumation along a Tethyan suture in northwest Turkey.Tectonophysics,285,275–299.10.1016/S0040-1951(97)00275-8
- Okay,A., &Kelley,S. (1994).Tectonic setting, petrology and geochronology of jadeite + glaucophane and chloritoid + glaucophane schists from northwest Turkey.Journal of Metamorphic Geology,12,455–466.10.1111/jmg.1994.12.issue-4
- Okay,A. I., &Monié,P. (1997).Early Mesozoic subduction in the Eastern Mediterranean: Evidence from Triassic eclogite in northwest Turkey.Geology,25,595–598.10.1130/0091-7613(1997)025<0595:EMSITE>2.3.CO;2
- Okay, A. I., & Tüysüz O. (1999). Tethyan sutures of northern Turkey. In Durand B., Jolivet L., Horváth F., Séranne M. (Eds.), The Mediterranean basins: Tertiary extension within the Alpine Orogen (pp. 475–515). London: Geological Society, Special Publications, 156.
- Okay,A. I.,Monod,O., &Monié,P. (2002).Triassic blueschists and eclogites from northwest Turkey: Vestiges of the Paleo-Tethyan subduction.Lithos,64,155–178.10.1016/S0024-4937(02)00200-1
- Perchuk,L. L., &Lavrent’eva,I. V. (1983).Experimental investigation of exchange equilibria in the system cordierite-garnet-biotite. InS. K.Saxena (Ed.),Kinetics and equilibrium in mineral reaction (pp.199–239).Berlin:Springer Verlag.10.1007/978-1-4612-5587-1
- Pidgeon,R. T.,Nemchin,A. A., &Hitchen,G. J. (1998).Internal structures of zircon from Archean granites from the Darling Range batholith: Implications for zircon stability and the internal interpretation of zircon U–Pb ages.Contributions to Mineralogy and Petrology,132,288–299.10.1007/s004100050422
- Pourteau, A., Candan, O., & Oberhänsli, R. (2010). High-pressure metasediments in central Turkey: Constraints on the Neotethyan closure history. Tectonics, 29, TC5004. doi:10.1029/2009TC002650
- Pourteau, A., Sudo, M., Candan,O., Lanari, P., Vidal, O., & Oberhänsli, R. (2013). Neotethys closure history of Anatolia: Insight from 40Ar–39Ar geochronology and P-T estimation in high-pressure metasediments. Journal of Metamorphic Geology, 31, 585–606. doi:10.1111/jmg.12034.
- Råheim,A., &Green,D. H. (1974).Experimental determination of the temperature and pressure dependence of the Fe–Mg partition coefficient for coexisting garnet and clinopyroxene.Contributions to Mineralogy and Petrology,48,179–203.10.1007/BF00383355
- Rimmelé,G.,Oberhänsli,R.,Goffé,B.,Jolivet,L.,Candan,O., &Çetinkaplan,M. (2003).First evidence of high-pressure metamorphism in the “cover series” of the southern Menderes Massif. Tectonic and metamorphic implications for the evolution of SW Turkey.Lithos,71,19–46.10.1016/S0024-4937(03)00089-6
- Rolland, Y., Billo, S., Corsini, M., Sosson, M., & Galoyan, G. (2008). Blueschists of the Amassia-Stepanavan Suture Zone (Armenia): Linking Tethys subduction history from E-Turkey to W-Iran. International Journal of Earth Sciences, 98, 533–550.
- Rolland, Y., Perincek, D., Kaymakci, N., Sosson, S., Barrier, E., & Avagyan, A. (2012). Evidence for ∼80–75 Ma subduction jump during Anatolide–Tauride–Armenian block accretion and ∼48 Ma Arabia-Eurasia collision in Lesser Caucasus-East Anatolia. Journal of Geodynamics, 56–57, 76–85.
- Rubatto,D. (2002).Zircon trace element geochemistry: Partitioning with garnet and the link between U–Pb ages and metamorphism.Chemical Geology,184,125–138.
- Rudnick,R.,Barth,M., &Horn,I. (2000).Rutile-bearing refractory eclogites: Missing link between continents and depleted mantle.Science,287,278–281.10.1126/science.287.5451.278
- Seaton,N. C. A.,Whitney,D. L.,Teyssier,C.,Toraman,E., &Heizler,M. T. (2009).Recrystallization of high-pressure marble (Sivrihisar, Turkey).Tectonophysics,479,241–253.10.1016/j.tecto.2009.08.015
- Sherlock,S.,Kelley,S. P.,Inger,S.,Harris,N., &Okay,A. I. (1999).40Ar–39Ar and Rb–Sr geochronology of high-pressure metamorphism and exhumation history of the Tavsanli Zone, NW Turkey.Contributions to Mineralogy and Petrology,137,46–58.
- Şengör,A. M. C. (1990).Plate-tectonics and orogenic research after 25 years – A Tethyan perspective.Earth-Science Reviews,27(1–2),1–201.
- Şengör,A. M. C.,Özeren,S.,Genç,T., &Zor,E. (2003).East Anatolian high plateau as a mantle supported, north–south shortened domal structure.Geophysical Research Letters,30,8045.10.1029/2003GL017858
- Şengör,A. M. C., &Yılmaz,Y. (1981).Tethyan evolution of Turkey, a plate tectonic approach.Tectonophysics,75,181–241.
- Şengün,M. (1983).The metamorphism and the relationship between infra and suprastructures of the Bitlis Massif – Turkey.Mineral Research and Exploration Bulletin,115,1–13.
- Şengün, M. (1993). Geological evolution of the Anatolian segment of the Tethyan belt. Geological Bulletin of Turkey, 36, 81–98.
- Sun,S. S., &McDonough,W. F. (1989).Chemical and isotopic systematics of oceanic basalts: Implications for mantle composition and processes. InA. D.Sanders &M. J.Norry (Eds.),Magmatism in the ocean basins (Vol.42, pp.313–345).London:Geological Society, Special Publications.
- Türkünal,S. (1980).Geology of the eastern and southeastern Anatolia (in Turkish).Ankara: Jeofizik Mühendisleri Odası Yayınları, 8, 64.
- Ustaömer,P. A.,Ustaömer,T.,Collins,A. S., &Robertson,A. H. F. (2008).Cadomian (Ediacaran–Cambrian) arc magmatism in the Bitlis Massif, SE Turkey: Magmatism along the developing northern margin of Gondwana.Tectonophysics, 473, 99–112. doi:10.1016/j.tecto.2008.06.010
- Vavra,G.,Schmid,R., &Gebauer,D. (1999).Internal morphology, habit, and U–Th–Pb microanalysis of amphibole to granulite facies zircon: geochronology of the Ivrea Zone (Southern Alps).Contributions to Mineralogy and Petrology,134,380–404.10.1007/s004100050492
- Whitney,D. L., &Davis,P. B. (2006).Why are lawsonite eclogites so rare?: Metamorphism and preservation of lawsonite eclogite, Sivrihisar, Turkey.Geology,34,473–476.10.1130/G22259.1
- Wu,Y., &Zheng,Y. (2004).Genesis of zircon and its constraints on interpretation of U–Pb age.Chinese Science Bulletin,49,1554–1569.
- Yılmaz,Y. (1993).New evidence and model on the evolution of the southeast Anatolian orogen.Bulletin of the Geological Society of America,105,252–271.
- Yılmaz,Y.,Yığıbaş,E., &Genç,C. (1993).Ophiolitic and metamorphic assemblages of southeast Anatolia and their significance in the geological evolution of the orogenic belt.Tectonics,12,1280–1297.10.1029/93TC00597
- Yuan,H. L.,Gao,S.,Liu,X. M.,Li,H. M.,Günther,D., &Wu,F. Y. (2004).Accurate U–Pb age and trace element determinations of zircon by laser ablation-inductively coupled plasma-mass spectrometry.Geostandards and Geoanalytical Research,28,353–370.10.1111/ggr.2004.28.issue-3