Abstract
In southeastern Turkey, the NE-trending Antakya Graben forms an asymmetric depression filled by Pliocene marine siliciclastic sediment, Pleistocene to Recent fluvial terrace sediment, and alluvium. Along the Mediterranean coast of the graben, marine terrace deposits sit at different elevations ranging from 2 to 180 m above present sea level, with ages ranging from MIS 2 to 11. A multisegmented, dominantly sinistral fault lying along the graben may connect the Cyprus Arc in the west to the Amik Triple Junction on the Dead Sea Fault (DSF) in the east. Normal faults, which are younger than the sinistral ones, bound the graben’s southeastern margin. The westward escape of the continental İskenderun Block, delimited by sinistral fault segments belonging to the DSF in the east and the Eastern Anatolian Fault in the north caused the development of a sinistral transtensional tectonic regime, which has opened the Antakya Graben since the Pliocene. In the later stages of this opening, normal faults developed along the southeastern margin that caused the graben to tilt to the southwest, leading to differential uplift of Mediterranean coastal terraces. Most of these normal faults remain active. In addition to these tectonic movements, Pleistocene sea level changes in the Mediterranean affected the geomorphological evolution of the area.
1. Introduction
One of the most important events in the Eastern Mediterranean’s tectonic evolution was the closing of the southern main, and the largest branch of Neotethys, the Bitlis-Zagros Ocean. During the Late Cretaceous, large ophiolite nappes from the northerly subducting Neotethys have obducted onto the Arabian Platform to the south (Aslaner, Citation1973; Dubertret, Citation1953). The Bitlis-Zagros Ocean was progressively consumed until the continental collision of the Arabian and the Anatolian Plates along the Bitlis-Zagros Suture Zone at the late Middle Miocene (Le Pichon & Angelier, Citation1979; McKenzie, Citation1972; Şengör, Citation1979). Most experts agree that the present Eastern Mediterranean Sea is a remnant of the Neotethys’ southern branch and still subducts northward beneath the Aegean-Anatolian Plate along the Hellenic-Cyprus Trench.
Wdowinski, Ben-Avraham, Arvidsson, and Ekstrom (Citation2006) divided the Cyprus Arc into three main segments: Predominantly sinistral motion at 7–8 mm/y characterizes the NE–SW oriented eastern segment. Collision between Sinai and Anatolian Plates at 7–8 mm/y characterizes the central segment. Along the western segment, the Nubian Plate subducts beneath the Anatolian Plate at 9–14 mm/y. An active NE–SW trending tear fault, the Paphos Fault, separates the central and western segments (Figure ).
Figure 1. A. Simplified tectonic setting of the eastern Mediterranean and surroundings, complied from Hall et al. (Citation2005) and Reilinger et al. (Citation2006). KOTJ: Karlıova Triple Junction, MTJ: Kahramanmaraş (or Türkoğlu) Triple Junction, ATJ: Amik Triple Junction, DSF: Dead Sea Fault, EAF: East Anatolian Fault, NAF: North Anatolian Fault, Sin: Sinai Block, ST: Strabo Trench, PT: Pliny Trench, Anb: Antalya Basin, Cb: Cilicia Basin, Mb: Mesaoria Basin, Lb: Latakia Basin, Cyb: Cyprus Basin, TR: Tartus Ridge, HF: Hatay Fault, MK: Misis-Kyrenia Fault Zone, Ab: Adana Basin, Ib: Iskenderun Basin, KOF: Karataş-Osmaniye Fault, PF: Paphos Fault. The white arrows and their corresponding numbers indicate the plate velocities relative to the Eurasian Plate, as derived from the GPS data. The black lines indicate major faults, and the arrows along the faults indicate offset direction. Hatched black lines with triangles indicate subduction zones. Hatched white rectangle shows location of inset map. B. The detailed bathymetry in the eastern Mediterranean Sea (after Hall et al., Citation2005). Black rectangle shows study area.
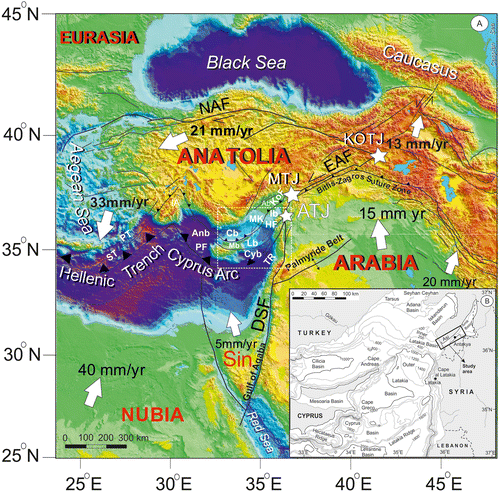
The Latakia Ridge on the eastern Cyprus Arc segment extends between the Hecataeus Ridge in the west and the Syrian coast near Latakia in the east (Figure (B); Hall, Aksu, Calon, & Yaşar, Citation2005). These two ridges mark the present plate boundary along the eastern Cyprus Arc. From deep seismic reflection data from south of Cyprus to the Syrian coast, Vidal, Alvarez-Marón, and Klaeschen (Citation2000) concluded that the deformation is partitioned here along strike-slip fault systems distributed over a zone more than 100 km wide, rather than forming a sharp plate boundary between African and Anatolian Plates. To the north of the Latakia-Hecataeus ridges sit the Cyprus, Mesaoria-Latakia and Cilicia basins (Figure (B)) that have been separated from each other by strike-slip faults. The boundary between the Cyprus and Latakia basins is also a sinistral fault system (Hall et al., Citation2005) that possibly trends eastward up to the Dead Sea Fault (DSF) through the study area, the Antakya Graben. This partly hypothetical fault is known as the Cyprus-Antakya Fault (CAF).
After the continental collision along the Bitlis-Zagros Suture, the Arabian Plate continued to move northward along the sinistral DSF (Figure (A)) and caused the Anatolian Plate to thicken and then to escape westward along the dextral North Anatolian and sinistral Eastern Anatolian (EAF) Faults (Figure ; Şengör, Citation1979). In the west, the retreating Hellenic Trench and the resulting slab-pull mechanism caused Aegean back-arc extension, which partly or completely facilitated the Anatolian Plate’s westward movement (Flerit, Armijo, King, & Meyer, Citation2004; Le Pichon & Angelier, Citation1979; Le Pichon, Lybéris, Angelier, & Renard, Citation1982; Mantovani, Viti, Babbucci, Tamburelli, & Albarello, Citation2006; Meulenkamp, Wortel, van Wamel, & Hoogerduyn Strating, Citation1988). GPS data indicate that the present movement rate of the Arabian Plate reaches up to 17.8 ± 1.1 mm/y (Figure ; Reilinger et al., Citation2006).
Different views exist about the offset and the age of initiation for the DSF, such as Early Miocene (Garfunkel, Zak, & Ben-Avraham, Citation1996; Hempton, Citation1987; Steinz & Bartov, Citation1991), Middle Miocene (Garfunkel, Citation1981; Khair, Krakaisis, & Papadimitriou, Citation2000), and Late Miocene (Lybéris, Citation1988), with total offsets at 105–110 km in the south and 60 km in the north (Garfunkel, Citation1981; Quennell, Citation1984; Westaway, Citation2003). On geological data, the slip rate was estimated at 3.8–10.0 mm/y (Quennell, Citation1958; McKenzie, Citation1970; Westaway, Citation2004), but at 3.0–7.5 mm/y both on geomorphological (Enzel, Amit, Bruce, Harrison, & Porat, Citation1994; Ginat, Enzel, & Avni, Citation1998) and archaeoseismological data (Ginat et al., Citation1998; Klinger et al., Citation2000; Meghraoui et al., Citation2003); 7.0 mm/y on palaeoseismological data (Gomez et al., Citation2003; Marco et al., Citation1997), and 4–8 mm/y based on GPS data (Alchalbi et al., Citation2010; McClusky, Reilinger, Mahmoud, Ben Sari, & Tealeb, Citation2003; Reilinger et al., Citation2006). Alchalbi et al. (Citation2010) suggested that the northern DSF demonstrates a slower slip rate, 1.8–3.3 mm/y, compare to the central and southern parts of the fault, possibly due to slip partitioning along the Palmyride active fold belt (Figure ). Although some authors, such as Girdler (Citation1990) and Butler, Spencer, and Griffiths (Citation1997, Citation1998), have advocated that the northern DSF has become inactive, recent GPS results (Alchalbi et al., Citation2010), field observations (Karabacak, Altunel, Meghraoui, & Akyüz, Citation2010), and palaeoseismological (Akyüz, Altunel, Karabacak, & Yalçıner, Citation2006) and archaeoseismological data (Altunel et al., Citation2009) demonstrated that it is still actively shearing and accumulating strain.
The DSF splits into different N- and NE-trending branches in northern Syria. The NE-trending segments end before the Turkish border, while two N-trending segments crosscut the border (Figures and ). Different geometries have been proposed for this part of the DSF (Karabacak & Altunel, Citation2013; Lybéris, Yürür, Chorowicz, Kasapoğlu, & Gündoğdu, Citation1992; Perinçek & Çemen, Citation1990; Rojay, Heimann, & Toprak, Citation2001; Westaway, Citation2003; Yürür & Chorowicz, Citation1998).
Figure 2. A digital elevation model for the study area and its surroundings, showing the major active faults and the morphotectonic units. MTJ: Kahramanmaraş or Türkoğlu Triple Junction, ATJ: Amik Triple Junction.
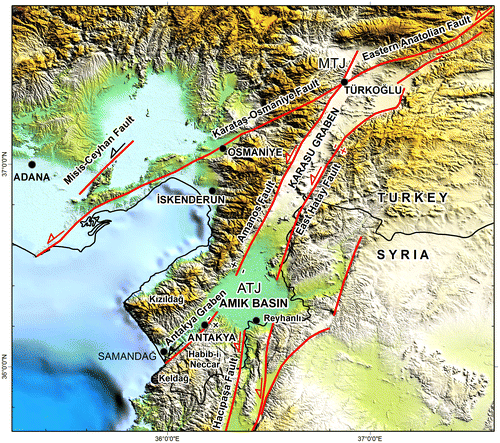
The Karasu (or Hassa) Graben in the north is delimited by two roughly N-trending normal sinistral faults, the Amanos Fault in the west, and the East Hatay Fault in the east (Figure ; Tatar, Piper, Gürsoy, Heimann, & Koçbulut, Citation2004). At the northern tip of the Karasu Graben is the Kahramanmaraş (or Türkoğlu) Triple Junction (MTJ), where the DSF connects to the EAF and the Karataş-Osmaniye Fault (KOF) (Figures and ; Gülen Barka, & Toksöz, Citation1987; Karig & Kozlu, Citation1990; Lybéris et al., Citation1992).
The Amanos Fault disappears in the Amik Basin in the south (Figure ). To the south of the Amik Basin, the Hacıpaşa Fault (Akyüz et al., Citation2006) is another segment of the DSF. Recent palaeoseismological data (Akyüz et al., Citation2006) showed that the Amanos and the Hacıpaşa Faults take the significant amount of slip in the northern part of the DSF. Karabacak et al. (Citation2010) proposed that the northern DSF began moving after the Pliocene and that 7.9 km of sinistral offset in buried pre-Quaternary Amik Basin suggests that the slip rate is at least 4.94 ± 0.13 mm/y for the Hacıpaşa Fault. Recently, however, Karabacak and Altunel (Citation2013) proposed a different scenario that, until the Late Pliocene, the DSF followed a different route through the Mediterranean Sea between the Hula Basin in Israel, and Amik Basin, and caused opening of the Amik Basin as a pull-apart basin. During the Late Pliocene–Pleistocene DSF migrated to its present position and the faults within the Antakya Graben became inactive.
The Antakya Graben is an asymmetrical topographical depression lying between the Amik Basin and the Mediterranean. The NE-trending faults in the Antakya Graben, corresponding to the onshore part of the CAF, start to be seen around the Mediterranean coast in the southwest, and reach to the Amik Basin in the northeast, where they disappear under or within the Quaternary alluvial fill. This geometry describes a triple junction in the Amik Basin connecting the Amanos Fault, the Hacıpaşa Fault, and CAF. Yürür and Chorowicz (Citation1998) and Över, Kavak, Bellier, and Özden (Citation2004) thought that the Amanos Fault forms the southern continuation of the EAF, joining to the DSF near the Amik Plain and to the Cyprus Arc via CAF, and that these faults form a FFF-type Amik Triple Junction (ATJ) in the Amik Basin. Mahmoud et al. (Citation2012) and Masson et al. (Citation2010) proposed FFT-type geometry for this triple junction. Recently, Meghraoui et al. (Citation2011) determined 3.8 ± 2 mm/y of sinistral and 1.8 ± 1.1 mm/y of normal offset along the Amanos Fault connecting the MTJ and the ATJ. They interpreted that the Karasu Graben opened behind the westward moving İskenderun Block delimited by MTJ, ATJ, and the Amanos Fault. From the K/Ar dates, the rift magmatism within the Karasu Basin occurred from 2.2 to 0.4 Ma (Parlak, Kop, Ünlügenç, & Demirkol, Citation1998).
The Antakya Graben sits on a critical position to test these different views on the geometry and kinematics of small blocks delimited by different fault segments and triple junctions mentioned above (Figure ). Although geology of the Antakya Graben and surroundings was partly or totally studied by different authors (Boulton & Robertson, Citation2007; Boulton & Robertson, Citation2008; Boulton, Robertson, Ellam, Şafak, & Ünlügenç, Citation2007; Boulton, Robertson, & Ünlügenç, Citation2006; Doğan et al., Citation2012; Över, Ünlügenç, & Bellier, Citation2002; Rojay et al., Citation2001; Yılmaz, Gürpınar, & Yiğitbaş, Citation1988), there is no agreement on the stratigraphy, fault geometry, and kinematics of the region, which is of critical importance to understand the tectonic evolution of the Eastern Mediterranean. We conducted a multidisciplinary campaign between 2006–2012 to produce a detailed geology map by using combined geological and geophysical methods and to test different hypotheses regarding the stratigraphy, structure, and recent tectonic evolution of this area.
In this paper, we concentrate on the Pliocene and younger geology of the Antakya (or Hatay) Graben (Figure ). In addition to geological and geophysical observations and measurements, we applied kinematic and morphometric methods to understand the state and effects of the recent tectonics. Our dating studies on the marine terrace deposits near Mediterranean coast are still continuing and we give also some preliminary results here.
2. Methodology
2.1. Geological mapping
Geology map of the region (Figure ) is classically produced in the field by using 1:25.000 scale topographical maps as base map. Locations of all observations recorded by using handheld GPS reaching up to 3 meters accuracy. Marine and fluvial terraces were mapped by using a Magellan Mobile Mapper Differential GPS (DGPS) to obtain below-meter (30–50 cm) accuracy in three directions.
The faults were traced in the field by using a Mala RAMAC Ground Penetrating Radar (GPR) System equipped with 500, 100, 50, and 25 MHz shielded and unshielded antennas, in addition to visual observations. GPR profiles were measured on each fault to precisely locate them, where the fault could not directly be observed due to surficial or artificial cover. Penetration depth of the GPR profiles ranges between 1 to 25 meters. These profiles are excluded to not increase the volume of the paper but reader can reach them from an open report (Tüysüz, Genç, İmren, & Tarı, Citation2012).
2.2. Seismic reflection profiles
We traced faults by using seismic reflection profiles in the southeastern part of the study area, where the faults are invisible due to surficial deposits, vegetation, and intense agricultural land use. Seismic reflection data were collected with 48 active (total 72) channels by using an electric-driven accelerated weight drop (25 kg) as the seismic energy source. The acquisition geometry was an off-end source-receiver array in that the offset and shot interval was 10 m and 2.5 m, respectively. The group (receiver) interval was 2.5 m and the Common-Depth Point (CDP) interval was 1.25 m. These parameters provided 24-fold CDP data. The sampling frequency was 0.5 ms with a record length of 1 s. The seismic line was positioned by DGPS. The data were processed in the Nezihi Canıtez Data Processing Laboratory, at the Department of Geophysics, Istanbul Technical University. Processing steps include geometry definition, static correction with a datum level at 5 m, editing the bad seismic traces, muting the direct and refracted arrivals, exponential and automatic gain corrections, band-pass filtering, CDP sorting, surface consistent residual static correction, velocity analysis, stack, and finite-difference wave-equation migration.
2.3. Kinematic analysis
The stress tensors and stress ratios for the faults were analyzed by using Stereonett (Johannes, Citation2000) and MyFault v.1.03 (Pangea Scientific) software with the Minimized Shear Stress Variation method (see details in Michael, Citation1984). Only measurements yielding high confidence ratios were used. The deviatoric stress ratio (R) was calculated by using following formula:(1)
where R = the deviatoric stress ratio,
σ1 = the compressional (maximum) deviatoric stress direction,
σ2 = the intermediate deviatoric stress direction, and
σ3 = the tensional (minimum) deviatoric stress directions.
R values > 0.55 indicate transpressional and R values < 0.45 indicate transtensional regimes.
3. Stratigraphy
The stratigraphic development of the Antakya Graben and its surroundings can be separated into palaeo- and neo-tectonic phases. The palaeotectonic phase in the Late Cretaceous to Miocene is mainly related with the closing of the Bitlis-Zagros Ocean. During the Campanian, the Mesozoic ophiolites were emplaced on the Arabian margin (Aslaner, Citation1973) and Maastrichtian to Miocene sediment was deposited on both the ophiolite nappes and the Arabian Platform. Since their evolution was completed before the Antakya Graben opened, the rock units developed during this first period are herein collectively called “basement rocks”. These rocks will briefly be described below. The rock units deposited during the neotectonic phase (The Plio-Quaternary units) will be called “Graben fill” and described in more detail (Figure ).
3.1. The basement rocks
At the visible base of the region, a well-studied ophiolitic complex (Figure ), the Kızıldağ Ophiolite (Aslaner, Citation1973; Bağcı, Parlak, & Höck, Citation2005, Citation2008; Dubertret, Citation1953; Inwood, Anderson, Morris, & Robertson, Citation2009 and references herein), consists of, from bottom to top, ultramafic tectonites, mafic and ultramafic cumulates, gabbros, sheeted dyke complexes, plagiogranites, pillow lava, and epi-ophiolitic sediment, such as cherts and pelagic limestones. U-Pb zircon ages for the plagiogranites in the Kızıldağ Ophiolite indicate its magmatic age as 90.3 ± 2.4 Ma (Parlak et al., Citation2009). On the basis of its age and tectonic setting, the Kızıldağ Ophiolite can be correlated with the Bear-Bassit and the Troodos ophiolites. Before the Late Maastrichtian, the Kızıldağ Ophiolite was emplaced over the autochtonous Cambrian to Campanian sediment on the Arabian Platform (Aslaner, Citation1973; Atan, Citation1969; Tekeli & Erendil, Citation1986).
Upper Maastrichtian carbonate-dominated sediment 50–150 m thick (Atan, Citation1969; Boulton et al., Citation2006, Citation2007; Boulton & Robertson, Citation2007; Tekeli & Erendil; Citation1986; Figure ) nonconformably overlies the Kızıldağ Ophiolite. The lower to middle Eocene (Kocaçiftçi & Ünlügenç, Citation2008) cherty and sandy limestones, which have some in situ reefs at base, overlie this neoautochtonous carbonate (Figure ). This 200–300 m thick Eocene shallow-marine sequence covers large areas, especially along the southeastern margin in the Antakya Graben. The Oligocene erosion followed the Eocene. The thick Miocene sedimentary sequence (see details in Boulton & Robertson, Citation2007) rests unconformably on the Eocene and the older units (Figure ).
In the study area, the Miocene sequenceFootnote1 can be correlated with those in the well-studied Adana Basin (Nazik, Citation2004; Schmidt, Citation1961; Ten Dam, Citation1952; Ternek, Citation1957; Yetiş, Citation1988; Yetiş, Kelling, Gökçen, & Baroz, Citation1995). At the base, the Aquitanian-Burdigalian red beds, siliciclastics and carbonates, locally called the Balyatağı formation (Şafak, Citation1993a, Citation1993b; Selçuk, Citation1985) were deposited in a continental to marginal marine environment. Overlying are the Latest Burdigalian-Langhian limestones (the Sofular formation; Şafak, Citation1993a, Citation1993b; Selçuk, Citation1985), which indicate a transgression associated with upwardly deepening deposition. Above, the limestones grade into the Langhian-Tortonian clayey limestones and marls (the Sebenoba formation, viz. Yılmaz et al., Citation1988; or the Tepehan and Nurzeytin formations, viz. Selçuk, Citation1985). Şafak (Citation1993a, Citation1993b) based his age assessments on foraminifera and ostracoda paleontology, whereas Boulton et al. (Citation2007) reported 87Sr/86Sr isotopic ratios consistent with ages of 13.24–7.17 Ma. In the lower part of the sequence, the marls indicate a transgression associated with upwardly deepening deposition, but then turn into a regressive sequence with thick, coarse conglomerate horizons in the upper part. During the Messinian, gypsum horizons alternate with marls and conglomerates (Figure ; Şafak, Citation1993a, Citation1993b; Selçuk, Citation1985). Coeval gypsum deposits occur throughout the Eastern Mediterranean, formed during a salinity crisis when the Mediterranean was isolated from the Atlantic Ocean (see CIESM, Citation2008; Faranda et al., Citation2013, and references therein). As the pre-Pliocene units act as a “basement,” these horizons have not been separated herein, but will be referred to as Sebenoba formation for the whole Langhian to Messinian sequence (viz. Yılmaz et al., Citation1988).
3.2. The graben fill
A thick Pliocene sedimentary succession, the Samandağ formation, and fluvial and marine terrace deposits form the main fill in the Antakya Graben (Figure ).
The Samandağ formation (Selçuk, Citation1985) comprises alternating marl, sandstone, siltstone, shale, and conglomerate. Marl and sandstones dominate the sequence, while conglomerates generally occur in the lower and upper sequence. Within the Antakya Graben, the lower contact for the Samandağ formation is rarely exposed. Boulton et al. (Citation2006, Citation2007) described its basal contact with the Messinian deposits as gradational and conformable, while Selçuk (Citation1985), Karakuş and Taner (Citation1994) and Şafak (Citation1993a, Citation1993b) recognized it as an angular unconformity. This unconformity outcrops most clearly in a road cut (UTM ZONE-37 240984/4016035; Figure ), where the Samandağ formation overlies the Sebenoba formation at a clear angular unconformity. In this location, the basal Samandağ formation comprises a very badly sorted, angular marl pebbles that rest on an unconformable surface, possibly indicating rapid erosion of a nearby uplifted area, such as a tilted fault block. Over this conglomerate lies another conglomerate horizon containing of coarse and badly sorted, grain-supported conglomerate with rounded clasts. Upsection, the conglomerate grades into intercalated homogenous, medium to thickly bedded sandstones, shales, and marl, deposited in a relatively quiet marine environment.
Figure 5. Angular unconformity (lower dashed line) between the southeast-dipping (right) Samandağ formation and overlying Sebenoba formation. At the base of the Samandağ formation is a very badly sorted, conglomerate with angular marl pebbles, which is overlain by another conglomerate containing of coarse and badly sorted but with rounded clasts. The contact between these two conglomerates is indicated by upper dashed line. See text for the location and explanation.
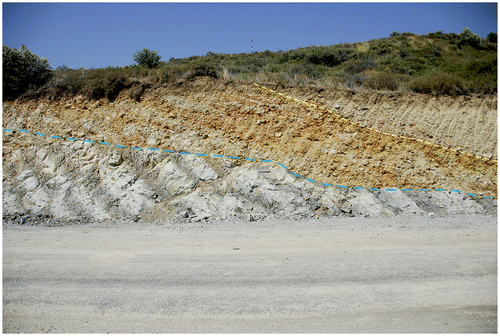
The molluscs suggest that the lower Samandağ formation was deposited in a littoral marine environment up to 150 m in depth, while the molluscs from the middle and upper formation reflect shallowing upward. The occurrence of some fluvial forms suggested a subtropical environment in the uppermost layers (Karakuş & Taner, Citation1994). The Samandağ formation reaches about 100–500 m in thickness and it is Piacenzian (Late Pliocene) in age (Karakuş & Taner, Citation1994).
Fluvial terrace deposits cover large areas within the Antakya Graben, especially around Büyük and Küçük Karaçay, the two biggest tributaries to the Asi River within the Antakya Graben. On the whole, resting unconformably on the Samandağ formation, the terrace deposits comprise grain-supported, badly sorted, loose boulders, and coarse conglomerates indicating a very high-energy depositional environment. Rounded and spherical pebbles and blocks reaching 2 m in diameter dominantly derived from the ophiolites. Limestone pebbles are smaller and rare. Palaeocurrent data suggest that the fluvial terraces were dominantly fed by the northwestern graben margin. Since the conglomerates lack fine grains, and nearly all their pebbles are ophiolitic, dominantly serpentinites and peridotites, these conglomerates could not be dated by palaeontological, 14C, optical simulated luminescence (OSL), or other methods.
In the southwestern Antakya Graben, near the Mediterranean coast, marine terrace deposits comprise homogenous beach and shore sands and conglomerates. Although the upper marine terrace deposits contain well sorted, cross-bedded greenish sands, in ophiolite and limestone clasts dominate, their basal lithology differ according to the underlying basement rock and the palaeoshore morphology.
In the marine terraces resting on the Miocene or Pliocene marls and sandstones, the base has well-sorted, cross-bedded sands with some conglomeratic horizons. Due to easily erodible nature of the underlying rocks, the basal topography in such places tends to be flat or gently undulating. In some locations, along the contact surface between the marls and overlying beach deposits, boring pelecypods, such as Lithophagus dug rounded holes, 3–8 cm deep and 1–2 cm in diameter, with the shells still preserved inside the holes.
Mainly located in the Antakya Graben’s southeastern margin, the marine terraces, which rest on the resistant Miocene limestones, start with a debris flow deposit that have limestone blocks and pebbles ranging from a few centimetres to a few metres in diameter embedded within a sand matrix. Pelecypods had also bored the surfaces on most of these blocks, indicating a previous coastal erosional process. The debris flows also contain reworked shells and shell fragments.
Marine terraces occur at different elevations along the Mediterranean coast (Erol, Citation1963; Pirazzoli, Laborel, Saliège, Kayan, & Person, Citation1991), the highest of which outcrops at 180 m apsl is on the southwestern graben margin. Recent wave-cut notches along the Mediterranean coast at 10–30 cm apsl indicate that uplifting is still active within the region. Although our studies on detailed classification, dating (ESR and AAR ages), and correlation of the marine terraces are still continuing, both our preliminary ages (Blackwell et al., Citation2011, Citation2012; Florentin et al., Citation2013) and published ESR and U dates (Doğan et al., Citation2012; Pirazzoli et al., Citation1991) indicate the terraces likely range from MIS 2 to 11.
4. Structural geology
N- and NE-trending faults are the main structural elements of the Antakya Graben. The faults in the northwestern margin and center of the graben are mainly obliquely or purely sinistral, while most of the faults on the southeastern margin are normal or obliquely sinistral (Figures and ).
Figure 6. The fault map for the Antakya Graben. The structural data from the faults within the Late Cenozoic units, all near the graben axis, show that the σ3 directions are nearly uniform in the graben and sit at a high angle to the graben margins. Filled arrows indicate minimum horizontal stress directions. Black encircled numbers indicate the kinematic data measurement locations. Rectangles show the locations for the seismic lines.
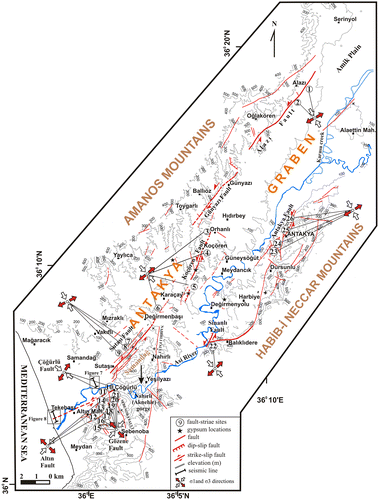
4.1. The faults on the northwestern margin
Two of the longest faults on the northwestern margin of the Antakya Graben are the Alazi and Günyazı Faults (Figures and ). Kinematic data on the 14 km-long Alazi Fault indicate its sinistral character with a normal component. This fault cuts the Miocene and Pliocene sediment in its southwestern part, while fluvial terraces overlie it near Havartepe (UTM ZONE-37 247206-4021939). In the northwest of the Alazi Fault, there are two more parallel faults within the Kızıldağ Ophiolites.
The Günyazı Fault trends parallel to the Alazi Fault. Although connection between Alazi and Günyazi Faults invisible in the field due to surficial cover, both faults are probably connected to each other. The Günyazi Fault cuts the Kızıldağ Ophiolite and the Miocene Sebenoba formation, but no kinematic data were observed on it.
4.2. The faults within the graben
The Koçören Fault (Figures and ) has a similar trend to the Alazi and Günyazı Faults and is separated from them by an extensional overstep. Cutting the Miocene sediment, the Koçören Fault is about 7 km long. Along the fault are some lenticular gypsum tectonic blocks, up to 10 m in diameter. In their lithology, these gypsum lenses can be compared with those in the Messinian sequence described above. Steep sinistral faults surround these lenses, which have a highly sheared internal structure. S-C structures within these blocks indicate dominantly sinistral movement with some normal component. These gypsum lenses are tectonically transported bodies along the Koçören Fault, and they are characteristic for all sinistral faults in the Antakya Graben. Kinematic data indicate that the sinistral Koçören Fault has some normal component, but in its southwestern tip (UTM ZONE-37 237436-4004521), it splays into some reverse faults forming a small positive flower structure, probably due to contractional overstepping on the Sutaşı Fault.
About 2 km east of Koçören Fault, the fluvial terrace deposits unconformably overlie another fault approximately 3 km long (UTM ZONE-37 239246-4006380). Kinematic data on this fault also indicate its sinistral to obliquely normal character.
The Sutaşı Fault (Figures and ) is about 8 km long. Morphologically, it is the best manifested fault in the Antakya Graben. It forms the contact between the Pliocene graben fill and the basement, including the ophiolites and the overlying Miocene limestones in the southeast. Kinematic data indicate obliquely sinistral character of this fault.
The Sutaşı Fault disappears under or within the alluvium in the southwest. This part of the fault is followed by seismic reflection and GPR profiles. A seismic reflection profile was measured on the southwestern continuation of the Sutaşı Fault, normal to the fault direction (Figure ).
Figure 7. Seismic reflection profile (A) on the Sutaşı Fault and its interpretation (B). See the text for explanations. The vertical exaggeration is ~2.3x. For the location of the seismic profile, see Figure 6.
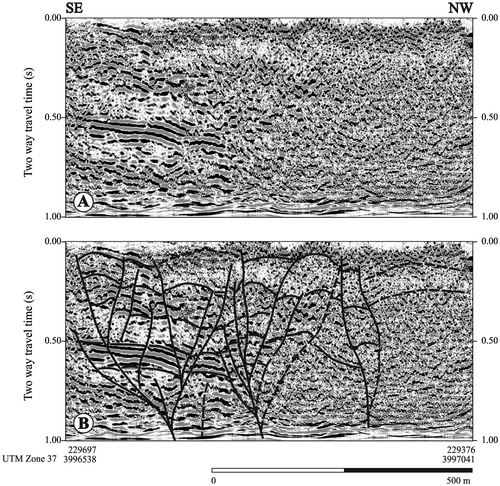
In the seismic reflection profile (Figure ), a 2 km wide distributed fault zone can be recognized. The fault pattern indicates at least two positive flower structures, implying a multi-branched strike-slip fault. Because the area where seismic profile measured was a highly disturbed agricultural field, similar to many spots in the study area, trenching was not possible, but clearly from the seismic profile, most faults approach the surface, indicating that they were recently active. The seismic profiles also show that the fault continues towards the Mediterranean within the alluvial deposits. These data suggest that this and similar sinistral faults continue offshore.
The Çöğürlü Fault (Figures and ) extends between Çöğürlü and the Mediterranean coast, forming contact between the Miocene and older basement rocks with the Pliocene graben fill. Along the fault, some gypsum lenses similar to those on the Koçören Fault occur. Due to the faulting, the Pliocene sediment just south of the fault dips steeply toward the vertical. Kinematic data indicate that the fault is sinistral. Having a normal character, the Gözene Fault cuts the Çöğürlü Fault. Like the Sutaşı Fault, the Çöğürlü Fault also displays a large deformation zone in the seismic reflection profile (Figure ), which indicates its multi-branched strike-slip nature.
4.3. The faults on the southeastern margin
The Altın Fault (Figures and ) has a concave northwestward geometry and pure normal character. It forms the contact between ophiolites, overlying Miocene limestones, and the Pliocene graben fill.
The Gözene Fault (Figures and ) is another concave northwestward fault with a purely normal offset. It forms the contact between the Miocene limestones and marls. By comparing the elevation of the contact between the serpentinites and overlying Miocene limestones on both blocks of the fault, 180–200 m vertical offset can be estimated. Both the Altın and the Gözene Faults display purely normal character according to lineaments on the fault planes. Some slickenlines indicating a normal offset overlie the slickenlines that indicate a sinistral offset in places, implying that the normal faults along the margins of the Antakya Graben are younger than the sinistral faults within the graben, and some sinistral faults reactivated once again as normal faults. On the other hand, the purely normal Gözene Fault cuts the sinistral Çöğürlü Fault.
On the road cut between Gözene and Çöğürlü villages (UTM ZONE-37 230700-3996415), the Gözene Fault forms the contact between the serpentinites of the Kızıldağ Ophiolite in the footwall and the slope deposits on the hanging wall (Figure ). A 14C date for a carbon sample within the slope deposits gave an age of 17.46 ± 0.07 ky BP (Sample number T4, Lab Nr. VERA-5587 VERA Laboratory, Vienna University; calibrated age is 19.3 ky with 95.4% confidence). These data indicate that the Gözene Fault is seismically active, and offers more support that the normal faults postdate the sinistral ones.
Figure 9. The Gözene Fault forms the contact between the serpentinites in the footwall (left) and the slope deposits on the hanging wall (right). Slickenlines on the fault surface indicate a pure normal offset. Dashed lines indicate different parts of the slope waste deposit having different attitudes, possibly indicating two different earthquakes. The arrow shows location of sample within the slope deposits for 14C dating.
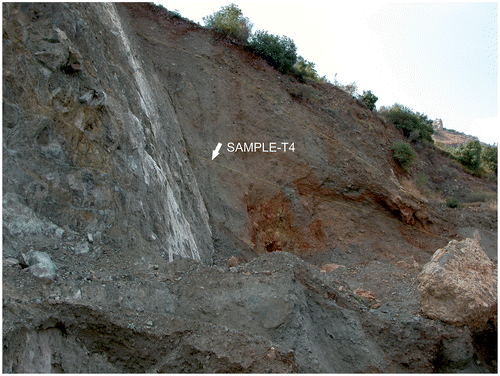
The Sinanlı Fault (Figures and ) cuts the Quaternary fluvial terraces, travertine, and scree deposits, as well as the basement units for about 8 km. Two overprinting slickenlines were determined on this fault, indicating an earlier obliquely sinistral and overlying pure normal movement. Around Balıklıdere Village (UTM ZONE-37 239272-4000901), two very close but different faults can be seen on this fault zone, a sinistral fault with a reverse component that is covered by fluvial terrace deposits and, about 6 m south of it, a normal fault that cut these terrace deposits (Figure ). These data also indicate that the normal faults postdate the sinistral faults, as previously indicated by Över et al. (Citation2004), and the weakness zones created by the sinistral faults were used later by the normal faults.
Figure 10. A schematic cross-section and photos along the Sinanlı Fault. On the northern side, fluvial terrace deposits overlie two reverse faults with 2.1 and 2.2 m stratigraphical offsets, while three normal faults on the southern side with 0.8–1.9 m stratigraphical offsets cut these deposits. The section is ~20 m long.
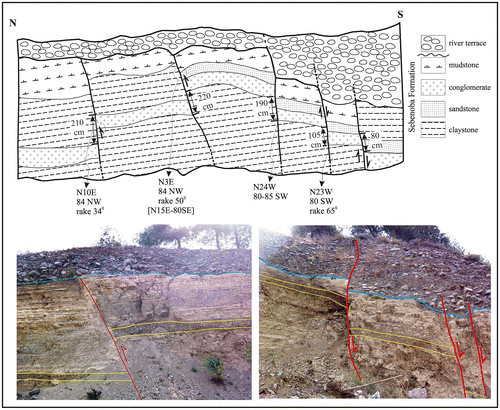
The Antakya Fault (Figures and ) is seen within the Antakya’s old city and can easily be recognized due to polished fault surfaces developed on the Eocene and the Miocene limestones. The oldest Christian church, Saint Pierre’s, sits in a cave that was dug on this fault’s surface. In its northern part, this 10 km-long fault separates the basement units from the Amik Plain alluvial deposits, while it has a distributed and split nature in the south. According to kinematic data, the fault has a dominantly normal component with a possibly older sinistral offset.
4.4. Kinematic data
Table lists kinematic data measured on the fault planes at 26 locations (Figure ). The R-values (Table ) indicate that both transtensional and extensional stress regimes occur in the region, creating dominantly sinistral and dominantly normal faults.
Table 1. Location of fault striae measurement sites.
Figure 11. Kinematic analysis of the faults within and on the margins of the Antakya Graben. See the text for explanations.
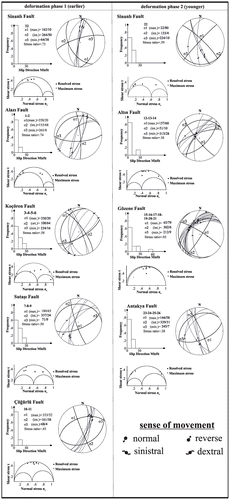
Table 2. Results of the kinematic analysis.
The Alazi, Günyazı, Koçören, Sutaşı, and Çöğürlü Faults have sinistral to obliquely sinistral character, and they are overlain by fluvial terrace deposits. These small faults having similar characteristics are interpreted as parts of an about 35 km-long fault trending between the Mediterranean coast and the Amik Plain. Seismic reflection data indicate that this fault is continuous below the waters of the Mediterranean towards the Cyprus Arc, and is probably onshore continuation of the CAF system. Kinematic data imply that this fault developed under a transtensional sinistral system, which is deactivated just before the deposition of fluvial terraces.
Kinematic data from the Altın, Gözene, Sinanlı, and Antakya Faults along the southeastern margin of the Antakya Graben indicate their pure normal or obliquely normal character. Most of these types of faults developed along the weakness zones of previous sinistral faults and they cut fluvial terraces. Additionally, marine terraces sitting on different elevations on the southeastern margin are delimited by such normal faults.
Given these observations, we interpret that the Antakya Graben started to open under the effects of the faulting having dominantly sinistral component, but an extensional system developed later within the graben as stated by Över et al. (Citation2002). This extensional system is probably active, as indicated by the age data from the Gözene Fault.
5. Morphology of the Antakya Graben
The Amanos Mountains to the northwest and the Habib-i Neccar and Ceb-el Akra (Keldağ) Mountains to the southeast border the Antakya Graben (or the Antakya-Samandağ Corridor). To the southeast, the Antakya Graben opens to the Mediterranean Sea, and is probably connected to the Latakia Basin under the waters of the Mediterranean Sea (Figure ; Hall et al., Citation2005). The morphology of the Antakya Graben was investigated by applying morphometric methods to understand the effects of the tectonic regime on the graben development during the Plio-Quaternary.
5.1. Geometry of the drainage network
The drainage network of the Antakya Graben comprises the Asi River in the center, and its asymmetric tributaries along both sides of the graben. The drainage network displays a parallel pattern on both sides of the graben, indicating structural control (Figure ). The valleys in the northwestern graben are longer and slope more gently compared to those in the southeast (Figures (A), (B)). Deeply incised into the basement units, including ophiolites and limestones upstream, the valleys are steep in the northwest, but become gentler towards the graben center, where they flow on the easily erodible Miocene and Pliocene bedrock. In the northwestern graben, the valleys stretch up to 32 km, while the longest southeastern valley is only 12 km long (Figure (C) and (D)). Due to this asymmetric structure, the drainage divide sits closer to the graben centre in the southeast.
Figure 12. The drainage network in the Antakya Graben. The encircled numbers show tributaries, the longitudinal profiles of which appear on Figure .
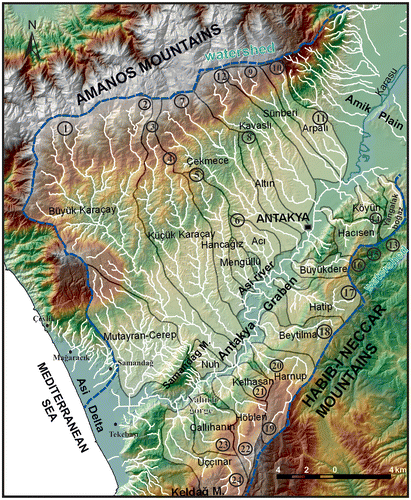
Figure 13. Longitudinal profiles of the tributaries to the Asi River; (A and B) on the NW graben flank, (C and D) on the SE graben flank. For the profile locations, see Figure .
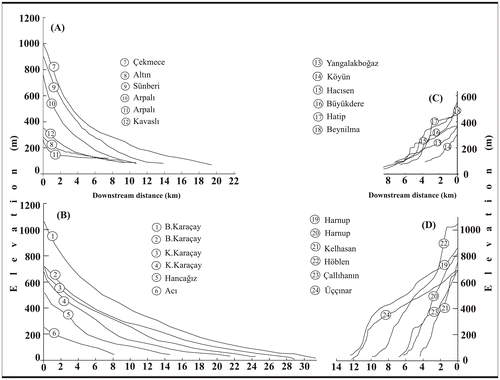
In an ideal case, without any lithological or structural control, both sides of a catchment should be symmetric around the basin center. For a perfectly symmetric basin, the Transverse Topographic Symmetry Factor (TTSF; Cox, Citation1994) would equal zero. As the asymmetry increases, the TTSF approaches 1.0. The calculated TTSF for the Antakya Graben ranges between 0.25 and 0.50 (Figure ), indicating that the basin center migrated southward about 2.85 to 6.80 km. As most of the basin sits on similar Upper Miocene and Pliocene marls and shales, this basin asymmetry can only be attributed to southeastward basin tilting, rather than lithological or climatic controls. Although the Asi River meanders, any evidence for systematic river avulsion triggered with such tilting could not be seen between Antakya and the Mediterranean coast, where the river flows mainly on a thin alluvium or bedrock. In contrast, southeastward avulsion of the Asi River during some historical earthquakes (e.g. the earthquake on 8 April 859 or 860 AD) has been reported (Guidoboni, Comastri, & Traina, Citation1994; Kaya & Kıyılı, Citation2009; Sbeinati, Darawcheh, & Mouty, Citation2005) on the Asi Delta, where some dried older meanders are still recognisable (Erol, Citation1963).
Figure 14. The TTSF (Cox, Citation1994) applied to the Antakya Graben. T, the basin asymmetry factor, averages 0.38. The T-vector values indicate that the basin center has migrated southward. Da: Distance to the basin midline, Dd: Distance between basin midline and drainage divide.
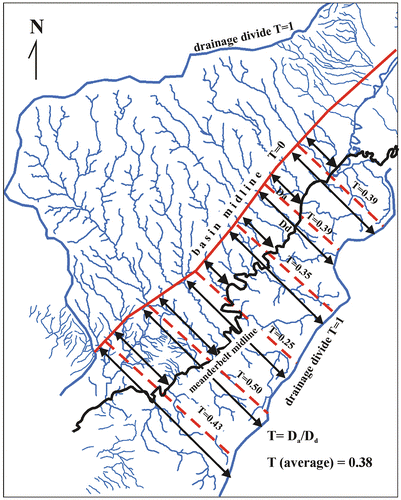
The hypsometric curve (total basin height against the total basin area) and hypsometric integral (the area under the hypsometric curve) can also elucidate the tectonic effects on the river morphology. Higher integral values, >0.5, indicate younger or immature valley geometry, while lower values, <0.4, indicate a gradual or mature geometry (For the details of the method see Keller & Pinter, Citation2002).
For the Asi River within the Antakya Graben, the hypsometric curve and integral values for 22 tributaries are calculated (Figure ). They range from 0.21 to 0.48 in the northwestern, and 0.47–0.65 in the southeastern margin. Given the absence of important climatic and lithological differences between the two sides of the graben, this difference in the hypsometric integral values can only be attributed to recent tectonic control. Clearly, from these data, the NE-trending dominantly normal faults in the southeastern graben margin control the vertical movements in the area. In contrast to these faults, the sinistral faults lying along the Antakya Graben do not display a characteristic signal affecting the graben morphology.
Figure 15. Hypsometric curves and hypsometric integral values for the Antakya Graben, (A) tributaries on the northwestern margin and (B) tributaries on the southeastern margin. A: the total surface of the basin, a: the surface area within the basin above a given elevation, h: the lowest elevation in the basin, and H: the highest elevation in the basin.
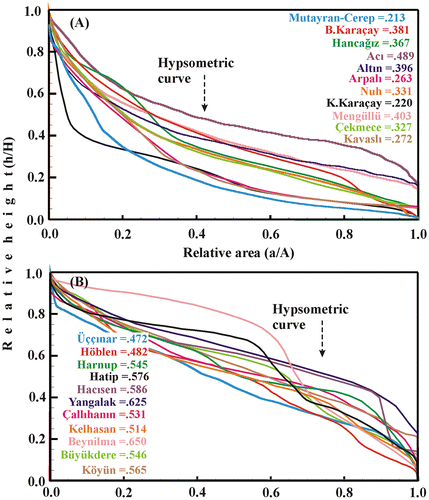
5.2. The river profile along the main Asi River channel
Also known as Orontes (in Greek) or Āşī (in Arabic), the Asi River rises in the eastern Beqaa Valley, Lebanon, and flows northward along the DSF. The central part of this meandering river’s valley ends at the rocky barrier at Jisr al-Hadid, where the river diverts to the west and the Amik Plain opens. The two large tributaries from the north, the Afrin and Karasu, here reach it across the former Amik Lake, which has now been drained through an artificial channel. The river turns abruptly west soon after entering the Amik Plain, and flows westward within a meandering valley along the south side of the plain. After passing through Antakya confined within an artificial channel, the river plunges southwest into the Antakya Graben, and finally forms a large delta along the Mediterranean coast, called the Samandağ (historical name, Seleucia Pieria) or Asi Delta (Figure ; Öner, Citation2008).
The meandering main Asi River flowing close to the southeastern margin of the Antakya Graben can be divided into several segments having different sinuosity. The sinuosity is higher, 2.30, in the Amik Plain, where the river flows across the nearly flat alluvial topography. Between the northeastern Antakya Graben and the Nahırlı (Aknehir) Gorge, where the river flows on a thin alluvium overlying the bedrock, mainly Miocene and Pliocene marls, sinuosity decreases to 1.62. After the Nahırlı Fault, the river enters the Nahırlı Gorge, where the sinuosity is 1.39. After passing the Sutaşı and Çöğürlü Faults, the river flows across the delta deposits, where its sinuosity is 1.40. This differentially decreasing of sinuosity from Amik Plain to the Mediterranean indicates differential increase in the valley slope due to structural control on the river.
While the channel pattern in a meandering river is closely related to the vertical tectonic movements, its sinuosity changes can be attributed to the effects of faulting, if no differences occur in the climate, sediment input, and the bedrock geology. As the self-organizing river behavior tends to keep the channel slope constant, increasing the valley slope downstream would increase the sinuosity, while increasing the valley slope upstream would cause the river to straighten and incise its channel. The Asi’s channel pattern implies that: 1. The Nahırlı, Sutaşı and Çöğürlü Faults caused the Samandağ block to uplift and the Asi River to incise the Nahırlı Gorge, 2. The Sinanlı Fault caused a sudden sinuosity change in the Asi River, possibly because the highly meandering river bed was downthrown along the Antakya and Sinanlı Faults (Figure ).
The longitudinal profile for the Asi River between the Amik Plain and the Mediterranean was prepared by using digital topography maps with 10 m resolution (Figure ). In the Amik Basin, the river bed sits ~84 m apsl. The total river length between its entrance into the Antakya Graben and the Mediterranean is ~52 km. The deepest gorge between Antakya and the Mediterranean is the Nahırlı Gorge that is marked by Nahırlı and Sutaşı-Çöğürlü Faults along both sides. The entrance to the gorge sits ~10 m higher than the exit, forming the steepest part of the profile. A good correlation exists between the steps (or knick points) in the profile and the elevations for the fluvial terraces and faults, indicating a clear tectonic control on the Asi valley’s development (cf. Figures and ).
5.3. The river offsets
The Sutaşı Fault, which marks the edge of the Samandağ Mountain uplifted basement rocks, serpentinites, and overlying Miocene limestones, against the Pliocene basin fill. On the NW-facing slope of this mountain, a trellis-type drainage network developed under the Sutaşı Fault’s control. The river offsets give some clues on the size of the offset along this sinistral fault (Figure (A)). Based on the beheaded valleys, ridges, wind gaps, captures, and river offsets, the digital elevation model for this region was restored to the pre-faulted condition (Figure (B)). This restoration implies that possibly ~750 ± 5 m of sinistral offset occurred along the Sutaşı Fault.
5.4. The fluvial terraces
The fluvial terraces at different elevations along the Asi River and its tributaries have previously been documented both in Turkey (Erol, Citation1963) and in Northern Syria (Bridgland et al., Citation2012; Bridgland, Philip, Westaway, & White, Citation2003 and references therein). The fluvial terraces cover a large area within the Antakya Graben, especially along the Asi River and its two largest tributaries, the Büyük Karaçay, and the Küçük Karaçay Rivers (Figure ; Erol, Citation1963). Our mapping studies (Figure ) indicated that there are fluvial terraces more than those indicated by Erol (Citation1963). As it was impossible to date these terrace deposits, they have been classified based on their elevations (Figure ).
Figure 18. Correlations between the fluvial terraces (circles) at different elevations along the Asi River and its tributaries. This study: solid black circles; Erol (Citation1963): open black circles. Lines indicate longitudinal profiles along the long tributaries to the Asi River. Roman numbers show the elevation groups for the terraces according to Erol (Citation1963), and dashed lines show the change in these levels along the river.
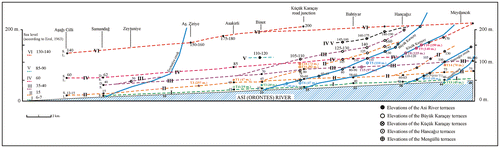
At 140–150 m apsl, the highest terraces in the region sit along the Küçük Karaçay River, where the terraces rest on the Pliocene sediment. Two other groups of fluvial terraces along the Küçük Karaçay lie between 120 and 128 m, and between 92 and 108 m. The 110 m terrace along the Büyük Karaçay and 113 m terrace along the Çekmece River can be correlated with the 108 m terraces along the Küçük Karaçay. Three other groups of fluvial terraces along the Büyük Karaçay sit at 82, 41–47 and 19 m. Along the Asi River’s main channel, the highest terrace deposits occur at 108 and 103 m, while many rest between 90 and 47 m, and the lowest ones lie between 40 and 25 m.
The fluvial terraces’ areal distribution indicates that all the terraces developed along the main Asi River and its tributaries flowing from the northwest. No terrace deposits line on the southeastern graben flank. The thickness of the terrace deposits increases to the southeast. These data also support the ideas that the Antakya Graben has an asymmetric structure, and that tilting and differential incision along the Asi River and its tributaries has controlled the fluvial terrace deposition on the northwestern flank. Moreover, the present asymmetric graben shape developed during the post-Pliocene tectonic evolution of the region, probably during the Pleistocene and Holocene, coeval with the terrace deposition.
5.5. The marine terraces
Along the Mediterranean coast, marine terrace deposits, wave-cut platforms, and notches (Erol, Citation1963) range from 25 cm to 180 m in elevation. Although the detail of these terrace deposits is the subject of another ongoing study, our preliminary results (Blackwell et al., Citation2011, Citation2012, Florentin et al., Citation2013) indicate that coeval marine terraces sit on different elevations on both sides of the Antakya Graben. ESR dating of in situ fossils such as Lithophagus from these deposits indicate an uplift rate of ~1.1 m/ky on the northern graben flank, but 2.1 m/ky on the southern flank of the Antakya Graben (Blackwell et al., Citation2011, Citation2012, Florentin et al., Citation2013). In the north, however, Doğan et al. (Citation2012), recognized MIS 5a terraces at 44 m (apsl) dated at ~72 ka, while the same horizon sat at 59 m in the south. Their results clearly show that the southeastern Antakya Graben uplifted about 15 m relative to the northwestern part since 72 ka. Based on ESR and 230Th/234U dating of MIS 5a terrace deposits, Doğan et al. (Citation2012) calculated an uplift rate of 0.88 ± 0.08 mm/y for the northern part and 1.08 ± 0.10 mm/y for the southern part. Although uplift rate from the current study and those from Doğan et al. (Citation2012) disagree, both data sets support the results from the regional geologic and geomorphological studies presented above, i.e. that the faults within the graben, or at least the Çöğürlü, Gözene, and Altın Faults are active and uplifted the graben differentially and its SE flank.
6. Distribution of the earthquakes
Although the study area and its surroundings were affected by destructive earthquakes during the historical period (Ambraseys, Citation1989; Ambraseys & Jackson, Citation1998; Ben-Menahem, Citation1991; Guidoboni et al., Citation1994; Guidoboni & Comastri, Citation2005; Khair et al., Citation2000; Sbeinati et al., Citation2005), no destructive (i.e. M ≥ 6) earthquake occurred during the instrumental period. Ambraseys (Citation1989) concluded that the earthquakes on 13 August 1822 and 3 April 1872 (M = 7.2) occurred in the Karasu valley, possibly on the Amanos Fault, and in the Amik Basin, respectively. Trench studies provided evidence for 3 historical earthquakes occurred in 859 AD, 1408, and 1872 on the Hacıpaşa Fault (Akyüz et al., Citation2006). Together with the 1996 Cyprus event (Mw = 6.8; Papazachos & Papaioannou, Citation1999), these events show that the three faults forming the ATJ are still active and producing earthquakes. According to the historical records, Seleucia Pieria (near modern Samandağ on the Mediterranean coast) was also intensely affected by some of these earthquakes more than Antakya. Uplifted borings from marine molluscs, at 0.75 m above modern sea level on the Asi Delta, have also been linked to the historical earthquake in AD 551 (Pirazzoli et al., Citation1991). Faulting within the Antakya Graben or its southwestern continuation under Mediterranean Sea likely produced some of these historical earthquakes. Age data provided from the Gözene Fault also support this interpretation.
Earthquakes smaller than M = 5.5 occurred in the region during the instrumental period, mostly on the EAF, DAF, and KOF, as well as on some local or unknown offshore faults. Nonetheless, on 24 June 1989 (ML = 5.1), 22 January 1997 (ML = 5.1 and 5.2), and 17 June 2009 (ML = 5.2), earthquakes occurred on the faults within the Antakya Graben. By inverting the slip vectors for these earthquakes, Över et al. (Citation2002) concluded that the present stress regime acting within the Antakya Graben is NW-SE extension.
7. Tectonic evolution and interpretation
During the terminal Middle Miocene, the final collision between the Arabian and Anatolian Plates marks the beginning of neotectonic period in the Eastern Mediterranean, when their regional evolution was controlled by: (a) continuing northward movement of the Arabian Plate along the DSF; (b) westward extrusion of the Anatolian Plate; and (c) subduction of the African Plate beneath the Anatolian and Aegean Plates.
Before the neotectonic period, Oligocene deformation and erosion followed the deposition of the Latest Cretaceous to Eocene transgressive sediment on the Arabian Platform and overlying ophiolite nappes. During the Early Miocene, a new marine transgression started. In the beginning, sea level rose, then receded until the Messinian, when the Mediterranean Sea, which was isolated of from the Atlantic Ocean when the Straits of Gibraltar closed, accumulated evaporates.
Boulton et al. (Citation2006) thought that the normal faulting associated with the marine sedimentation in the Antakya Graben area during the Middle Miocene resulted from far-field stresses related to continental collision along the Bitlis-Zagros Suture to the north. Moreover, they were reactivated during the Pliocene due to foreland flexure that caused the Antakya Graben to form. Our observations indicate that an angular unconformity separates Miocene and Pliocene sediment (Figure ), which indicates a deformation phase, or tilting of fault blocks, during the beginning of the Pliocene sedimentary deposition. This faulting period can be attributed to the opening of the Antakya Graben.
The Pliocene sediment is confined to the Antakya Graben, and no palaeontological evidence for Early Pliocene sedimentation exists in the area. Although Boulton et al. (Citation2007) attribute the first appearance of Globigerinoides trilobus sacculifer, G. obliquus obliquus, and Globorotalia sticula from the basal part of the Samandağ formation characterize the base of the Pliocene, Şafak (Citation1993a) found the same fossils occurred within the underlying Langhian-Serravalian sequence. Based on a 87Sr/86Sr isotopic ratio age of 5.35 Ma, Boulton et al. (Citation2007) assigned a Pliocene age to the Samandağ formation, but this age corresponds to the latest Messinian, rather than earliest Pliocene (International Chronostratigraphic Chart; www.stratigraphy.org). Given these data and the unconformity separating the Samandağ formation from the underlying units (Figure ), the Samandağ formation is most likely Piacenzian (3.6–2.6 Ma) in age, as Karakuş and Taner (Citation1994) proposed based on mollusc data from five measured sections. Given these data, the Antakya Graben must have opened during the Late Pliocene. Considering the initiation of the rift magmatism in the Karasu Graben about 2.2 Ma (Parlak et al., Citation1998), it can be interpreted that both the Karasu and Antakya Grabens opened coevally, under the control of same tectonic regime, but only the Antakya Graben was connected to the Mediterranean.
The Pliocene marine sediment in the Antakya Graben comprises thick conglomerate horizons, lenses, and channels, especially in the upper sequence. Fed mainly by Eocene and Miocene limestones, these conglomerates indicate that the basal ophiolites were still not sufficiently exhumed to provide the dominant clastic material to the Pliocene sediment. Conglomerate channels cover and cut the fine-grained parts of the formation in places, indicating that a fan-delta and associated submarine channels reached into the graben, where marls, silts, and sandstones were deposited in a marine environment. The increasing thicknesses and conglomerate frequency with height the section, which coarsen upward indicate a regressive period, was followed by tropical subaerial exposure in the latest Pliocene (Karakuş & Taner, Citation1994). All these data imply that the Antakya Graben was a short-lived marine deposition area during the Late Pliocene.
Although Yürür and Chorowicz (Citation1998) and Doğan et al. (Citation2012) reported major faults delimiting the whole northwestern margin of the Antakya Graben, such faults were not evident in our detailed mapping campaign. Clearly, field observations showed that the NE-trending sinistral faults in the northeastern part of the graben do not follow the whole margin and they move to the center of the graben southwestward. In the northeast margin, the Miocene sediment transgressively overlies the Kızıldağ Ophiolite, and that this undisturbed primary contact can be traced nearly all along the southern slope on Kızıldağ Mountain. Boulton and Whittaker (Citation2009) also concluded that northern graben margin is tectonically quiescent, and that previous interpretations for major faults on the northern margin were without foundation.
The geological and geomorphological data outlined above support the interpretation that post-Late Pliocene tectonic movements affected the development of an asymmetric structure in the Antakya Graben:
Parallel drainage along both sides of the graben indicates structural control on the drainage system.
The central drainage indicates that the graben center shifted southeastward.
The tributaries on the graben’s northwestern flank are longer, gentle, and gradual, while tributaries on the southeastern flank are short, steep, and immature.
The thickness of the fluvial terraces that were deposited on the northwestern flank and along the central drainage increase towards the central graben implying: (a) southeastward tilting of the NW flank; (b) rapid uplift of Kızıldağ Mountain; and (c) rapid erosion of the uplifted areas that provided coarse alluvial material to the tributaries that reached the central Asi River.
Pliocene sediment deposited within the graben was mainly derived from Eocene and Miocene units. In the Pliocene sediment, the dominant clasts within the conglomerate horizons are Eocene and Miocene limestones, with <30% ophiolite fragments. The palaeocurrent analyses imply that the sediment source existed to the northwest, where presently ophiolites outcrop. Unlike the Pliocene sediment, the fluvial terraces contain 80–90% ophiolite fragments derived from the same margin. This indicates that the Kızıldağ Ophiolite was mainly exhumed after the Pliocene sedimentary deposition, and just before, or coeval with, the fluvial terrace deposition.
Along the Antakya-Samandağ Road at a basal elevation of 59 m (UTM ZONE-37 238279/4004792), fluvial terraces unconformably overlie thin marine clastic units that have been ESR dated at 107 ± 8 ka (Blackwell et al., Citation2011). They indicate that: (a) During MIS 5d, the sea approached Antakya, or even the Amik Plain; and (b) At least some fluvial terraces are younger than 107 ± 8 ka.
Despite the thick fluvial terraces that occur along their courses, most tributaries on the northwestern flank, and even many parts of the central Asi River, flow on the bedrock, indicating incision of drainage system after the fluvial terrace deposition. This can be achieved in two ways, base-level changes and/or regional uplift.
In the Mediterranean, frequent, rapid sea level changes occurred throughout the Quaternary. After MIS 5a, Mediterranean sea-level fall to a minimum of −130 m during MIS 2. Most fluvial terraces deposited during MIS 5–2 (76–11 ka), because their internal structure implies that they were deposited in a very high energy environment, possibly indicating lowest base-level position. The ESR data above also support this idea. After MIS 2 at 11.6 ka, sea level rose and remained high throughout MIS 1. This implies that the fluvial incision related with the base-level change cannot be younger than MIS 2. Therefore, any incision after MIS 2 had to have been controlled by tectonic uplift rather than base-level changes.
The marine terraces that occur at different elevations along the Mediterranean coast imply differential uplift across the region. In the study area, near Mağaracık, in the northern Asi Delta, the 25 m to 105 m terraces yielded preliminary ESR dates of 67 ± 9 to 113 ± 12 ka, which correlate with MIS 5a–5e, while the 170 m terrace near Tekebaşı (Figure ) overlooking the southern Asi Delta gave a preliminary age of 398 ± 24 ka, correlating with MIS 11 (Blackwell et al., Citation2011). MIS 11 terraces are absent in the northern part. Doğan et al. (Citation2012) suggested that the MIS 5a (72 ka) terraces had been uplifted ~ 15 m more than those in the northern graben. Pirazzoli et al. (Citation1991) described two emerged but much younger shorelines on both sides of the Asi Delta at +2 and +0.8 m. They thought that the upper shoreline could date to 2.5 ± 0.1 ky BP, while the lower shoreline possibly had been uplifted during the earthquake in July 551 AD that reactivated the faults around Hatay. These data also support the interpretation here that differential uplifting occurred in the Antakya Graben during the Quaternary. Uplift on the Mediterranean coast probably still continues, since the Palaeolithic Merdivenli Cave sits at ~50 m apsl (Şenyürek & Bostancı, Citation1958) and the Middle Paleolithic Üçağızlı Cave, at ~20 apsl (Kuhn et al., Citation2009), and the ancient harbor, Seleucia Pierria (Erol & Pirazzoli, Citation2007) now sits above sea level.
Given the data herein, we interpret that the Antakya Graben opened on a transtensional fault system connecting Cyprus Arc to the Amanos and the Hacıpaşa segments of the DSF, when the basin was connected to the Mediterranean, as indicated by the outcrop distribution for the Pliocene sediment. The regressive Pliocene sediment package and the unconformably overlying terrace deposits indicate that marine sedimentation ended in the latest Pliocene. Subsequently, transtensional and then extensional faults along the southeastern graben margin controlled its tectonic and morphological development.
Chronological observations on the kinematic data show that extensional movement in the Antakya Graben postdates the sinistral movement. 14C data and present earthquake activity also implies that the extensional regime is still active in the region. Based on focal mechanism solutions of small to moderate earthquakes occurred in the region, Över et al. (Citation2002) identified a stress field is dominantly extensional with a NE-trending σ3 axis, which agrees with this study’s kinematic data (Figures and ; Table ). Över et al. (Citation2002) attributed this extensional period to a roll-back effect of the Cyprus Arc. Meanwhile, offshore seismic activity between Cyprus and the Antakya Graben is dominantly sinistral (Makris, Stacker, & Kramvis, Citation2000). Based on these data and convex geometry of the normal faults, we interpret that the extensional structures in the Antakya Graben developed during the mature stage of the regional sinistral movement as relaxation structures.
In the light of data presented above and regional plate kinematics (Figure ), we interpret that the İskenderun Block start to escape westward, as proposed previously by Yürür and Chorowicz (Citation1998), during the Late Pliocene (2.2 Ma) as evidenced by rift magmatism in the Karasu Graben. GPS data by Meghraoui et al. (Citation2011) and Mahmoud et al. (Citation2012) showed that differential movements of continental blocks (or fragments) delimited by strike-slip faults is still continuing. Westward movement of the İskenderun Block caused opening of the Karasu Graben in the east and created a transtensional regime along the sinistral CAF. Under this transtensional regime the Antakya Graben opened as NE continuation of the Latakia Basin, but it quickly filled probably due to its narrow structure and fast uplifting graben shoulders.
Figure 19. The GPS velocity field relative to fixed Arabian Plate (GPS data from Alchalbi et al., Citation2010; Meghraoui et al., Citation2011; Reilinger et al., Citation2006). The abbreviations indicate GPS observation campaigns by Reilinger et al. (Citation2006) and Alchalbi et al. (Citation2010). The fault slip rates (mm/y) were deduced from Mahmoud et al. (Citation2012). The top numbers in each rectangle give strike-slip rates, positive being left-lateral. The other numbers in each rectangle give fault-normal slip rates, positive equalling closing. CAF – Cyprus-Antakya Fault. For the other abbreviations, see Figure .
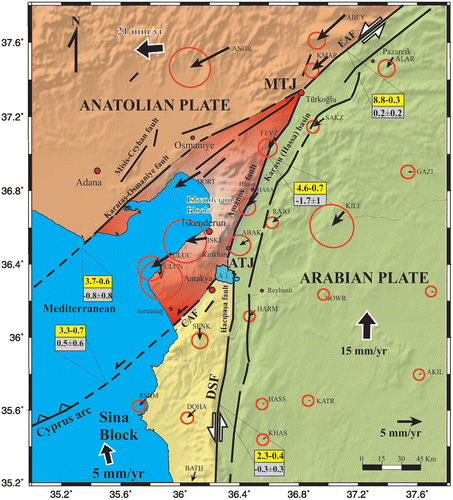
Sinistral faults within the Antakya Graben forming the onshore prolongation of the CAF cut the Pliocene sediment, but are overlain by terrace deposits, indicating a change in the tectonic regime from dominantly sinistral transtension to pure extension after the Latest Pliocene. At this period, weakness zones created by sinistral faults inverted into normal faults at the SE margin, as indicated by overprinted slickenlines, while ones within and northern margin of the graben deactivated. Intrusion of Mediterraean Sea waters into the Antakya Graben towards the Amik Basin about 107 ± 8 ka was possibly facilitated by this extensional tectonic regime.
Normal faults at the SE margin caused tilting of the graben’s floor southeastward, while uplifting northeasten margin fast eroded and the exhuming Kızıldağ Ophiolites provided clasts to the fluvial terraces. Southeastern margin of the graben also uplifted relative to graben center. Morphology, marine terraces, and age data from the Gözene Fault indicate that this tectonic regime is still active in the region.
8. Conclusions
Two (FFF- and FFT-type) triple junctions occur along the northern DSF. The northern one, the MTJ, connects the DSF to the Eastern Anatolian and KOF, while the southern one connects the DSF to the Cyprus Arc via CAF. Westward movement of the İskenderun Block, which is bounded by these triple junctions and the faults connecting them, caused the Karasu Graben to open to the east. This movement also caused transtensional opening of the Antakya Graben south of the İskenderun Block beginning in the Late Pliocene (Figure ). Extensional movements along graben controlled the Quaternary tectonic evolution of the Antakya Graben. Along with the tectonic movements, sea level changes played an important role in the Antakya Graben’s morphologic evolution.
Acknowledgments
The TÜBİTAK 109Y128 and İTÜ BAP 32903 projects funded this work. The Municipality of Hatay provided local facilities during the fieldwork. Rasim Can of State Hydraulic Works (Hatay), Süleyman Ray, Mayor of Kuşalanı Town and Sancar Saraçoğlu of Fina Energy supported field studies. Dr. Murat Nurlu, Head of the Earthquake Department of Disaster and Emergency Management Presidency provided some seismic equipment. ESR dating was partially supported by Williams College, the RFK Science Research Institute, and McMaster Nuclear Reactor. Comments by Dr. Erdin Bozkurt, and two anonymous reviewers helped improving the manuscript. We cordially thank all these institutions, person and the friendly people of Hatay for their help and support.
Notes
1 In the study area, the stratigraphic nomenclature for the Miocene sediment is problematic. Recently, Boulton et al. (Citation2007) tried to revise the stratigraphy in the Hatay region, but, unfortunately, they increased the existing chaos, since they used unpublished local formation names, rather than previously published and regionally correlated names. Boulton et al. (Citation2007) indicated that Atan (Citation1969) published the regional stratigraphic terminology, but Atan (Citation1969) did not study or name any Miocene unit. Boulton et al. (Citation2007) may not have seen Atan (Citation1969), because they do not cite it correctly. Boulton et al. (Citation2007) noted that Şafak (Citation1993a) had divided the Miocene succession into five new biostratigraphic units, but Şafak (Citation1993a) referred to Selçuk (Citation1985) for these formation names. In fact, two reports by Selçuk (Citation1981, Citation1985) first defined all these names. To not increase the confusion further, however, mostly local formation names are used here, since the Miocene units will be described only briefly.
References
- Akyüz, H. S., Altunel, E., Karabacak, V., & Yalçıner, C. Ç. (2006). Historical earthquake activity of the northern part of the Dead Sea Fault Zone, southern Turkey. Tectonophysics, 426, 281–293.
- Alchalbi, A., Daoud, M., Gomez, F., McClusky, S., Reilinger, R., Romeyeh, M. A., … Barazangi, M. (2010). Crustal deformation in northwestern Arabia from GPS measurements in Syria: Slow slip rate along the northern Dead Sea Fault. Geophysical Journal International, 180, 125–135.
- Altunel, E., Meghraoui, M., Karabacak, V., Akyüz, H. S., Ferry, M., Yalçıner, C. Ç., & Munschy, M. (2009). Archaeological sites (Tell and Road) offset by the Dead Sea Fault in the Amik Basin, southern Turkey. Geophysical Journal International, 179, 1313–1329.
- Ambraseys, N. N. (1989). Temporary seismic quiescence: SE Turkey. Geophysical Journal International, 96, 311–331.
- Ambraseys, N. N., & Jackson, J. A. (1998). Faulting associated with historical and recent earthquakes in the eastern Mediterranean region. Geophysical Journal International, 133, 390–406.
- Aslaner, M. (1973). İskenderun ve Kırıkhan bölgesindeki ofiyolitlerin jeolojisi ve petrografisi [Geology and petrography of the ophiolites in the Iskenderun and Kırıkhan region]. (Vol. 150, 71 p.). Ankara: Maden Tetkik Arama Enstitüsü Yayınları.
- Atan, O. E. (1969). Eğribucak-Karacaören (Hassa)-Ceylanlı-Dazevlerî (Kırıkhan) arasındaki Amanos dağlarının jeolojisi [Geology of the Amanos Mountains between Eğribucak-Karacaören (Hassa)-Ceyhanlı-Dazevleri (Kırıkhan)]. (Vol. 139, 85 p.). Ankara: Maden Tetkik Arama Enstitüsü Yayınları.
- Bağcı, U., Parlak, O., & Höck, V. (2005). Whole rock and mineral chemistry of cumulates from the Kızıldağ (Hatay) ophiolite (Turkey): Clues for multiple magma generation during crustal accretion in the southern Neotethyan ocean. Mineralogical Magazine, 69, 53–76.
- Bağcı, U., Parlak, O., & Höck, V. (2008). Geochemistry and tectonic environment of diverse magma generations forming the crustal units of the Kızıldağ (Hatay) ophiolite, southern Turkey. Turkish Journal of Earth Sciences, 17, 43–71.
- Ben-Menahem, A. (1991). Four thousand years of seismicity along the Dead Sea Rift. Journal of Geophysical Research, 96, 20195–20216.
- Blackwell, B. A. B., Florentin, J., Tüysüz, O., Tarı, U., Genç, Ş. C., İmren, C., … Kim, M. (2011). An uplifting idea: ESR dating marine terraces in Hatay province, Turkey. Geological Society of America Abstracts with Programs, 43, 273.
- Blackwell, B. A. B., Florentin, J. A., Tüysüz, O., Tarı, U., Genç, Ş. C., İmren, C., … Blickstein, J. (2012). Slipping up? ESR dating molluscs from marine terraces in Hatay Province, Turkey. Geological Society of America Abstracts with Programs, 44, 296.
- Boulton, S. J., & Robertson, A. H. F. (2007). The Miocene of the Hatay area, S Turkey: Transition from the Arabian passive margin to an underfilled foreland basin related to closure of the Tethys Ocean. Sedimentary Geology, 198, 93–124.
- Boulton, S. J., & Robertson, A. H. F. (2008). The Neogene–Recent Hatay Graben, South Central Turkey: Graben formation in a setting of oblique extension (transtension) related to post-collisional tectonic escape. Geological Magazine, 145, 800–821.
- Boulton, S. J., Robertson, A. H. F., Ellam, R. M., Şafak, Ü., & Ünlügenç, U. C. (2007). Strontium isotopic and micropalaeontological dating used to help redefine the stratigraphy of the neotectonic Hatay Graben, southern Turkey. Turkish Journal of Earth Science, 16, 141–179.
- Boulton, S. J., Robertson, A. H. F., & Ünlügenç, U. C. (2006). Tectonic and sedimentary evolution of the Cenozoic Hatay Graben, southern Turkey: A two-phase, foreland basin then transtensional basin model. In A. H. F. Robertson & D. Mountrakis (Eds.), Tectonic development of the eastern Mediterranean region (pp. 613–634). London: Geological Society of London, Special Publication 260.
- Boulton, S. J., & Whittaker, A. C. (2009). Quantifying the slip rates, spatial distribution and evolution of active normal faults from geomorphic analysis: Field examples from an oblique-extensional graben, southern Turkey. Geomorphology, 104, 299–316.
- Bridgland, D. R., Philip, G., Westaway, R., & White, M. (2003). A long Quaternary terrace sequence in the Orontes River valley, Syria: A record of uplift and of human occupation. Current Science, 84, 1080–1089.
- Bridgland, D. R., Westaway, R., Romieh, M. A., Candy, I., Daoud, M., Demir, T., … Whittaker, J. (2012). The River Orontes in Syria and Turkey: Downstream variation of fluvial archives in different crustal blocks. Geomorphology, 165–166, 25–49.
- Butler, R. W. H., Spencer, S., & Griffiths, H. M. (1997). Transcurrent fault activity on the Dead Sea transform in Lebanon and its implications for plate tectonics and seismic hazard. Journal of the Geological Society London, 154, 757–760.
- Butler, R. W. H., Spencer, S., & Griffiths, H. M. (1998). The structural response to evolving plate kinematics during transpression: Evolution of the Lebanese restraining bend of the Dead Sea transform. In R. E. Holdsworth, R. A. Strachan, & J. F. Dewey (Eds.), Continental transpressional and transtensional tectonics (pp. 81–106). London: Geological Society of London, Special Publication 135.
- CIESM. (2008). The Messinian Salinity Crisis from mega-deposits to microbiology. A concensus report (Report No. 33). In F. Briand (Ed.), CIESM Workshop Monographs, Monaco, 168 p.
- Cox, R. T. (1994). Analysis of drainage basin symmetry as a rapid technique to identify areas of possible Quaternary tilt-block tectonics: An example from the Mississippi Embayment. Geological Society of America Bulletin, 106, 571–581.
- Doğan, U., Koçyiğit, A., Varol, B., Özer, İ., Molodkov, A., & Zöhra, E. (2012). MIS5a and MIS3 relatively high sea-level stands on the Hatay–Samandağ coast, eastern Mediterranean, Turkey. Quaternary International, 262, 65–79.
- Dubertret, L. (1953). Géologie des roches vertes du nord-ouest de la Syrie et du Hatay (Turquie) [Geology of the green rocks in the northwest Syria and Hatay (Turkey)]. Notes et Mémoires sur le Moyen-Orient, 6, 227.
- Enzel, Y., Amit, R., Bruce, J., Harrison, J., & Porat, N. (1994). Morphologic dating of fault scarps and terrace risers in the southern Arava, Israel: Comparison to other age-dating techniques and implications for paleoseismicity. Israel Journal of Earth Science, 43, 91–103.
- Erol, O. (1963). Asi Nehri deltasının jeomorfolojisi ve dördüncü zaman deniz-akarsu sekileri: Die Geomorphologie des Orontes-Deltas und der anschliessenden pleistozänen Strand-und Flussterrassen, Provinz Hatay, Türkei[Geomorphology of the Asi River delta and Pleistocene marive and river terraces] (Vol. 148, 110 p.). Ankaras: Dil ve Tarih-Coğrafya Fakültesi Yayınları.
- Erol, O., & Pirazzoli, P. A. (2007). Seleucia Pieria: An ancient harbour submitted to two successive uplifts. International Journal of Nautical Archaeology, 21, 317–327.
- Faranda, C., Gliozzi, E., Cipollari, P., Grossi, F., Darbaş, G., Gürbüz, K., … Cosentino, D. (2013). Messinian paleoenvironmental changes in the easternmost Mediterranean Basin: Adana Basin, southern Turkey. Turkish Journal of Earth Sciences, 22, 839–863. doi:10.3906/yer-1205-11
- Flerit, F., Armijo, R., King, G., & Meyer, B. (2004). The mechanical interaction between the propagating North Anatolian Fault and the back-arc extension in the Aegean. Earth and Planetary Science Letters, 224, 347–362.
- Florentin, J. A., Blackwell, B. A. B., Tüysüz, O., Tarı, U., Genç, Ş. C., İmren, C., Mo, S., Huang, Y. E. W., BLlickstein, J. I. B., Skinner, A. R., Kim, M. (2013). ESR dating molluscs from marine terraces near Mağaracık and Samandağ, Hatay Province, Turkey. Quaternary Geochronology.
- Garfunkel, Z. (1981). Internal structure of the Dead Sea leaky transform (rift) in relation plate kinematics. Tectonophysics, 80, 81–108.
- Garfunkel, Z., Zak, I., & Ben-Avraham, Z. (1996). The structure of the Dead Sea Basin. Tectonophysics, 266, 155–176.
- Ginat, H., Enzel, Y., & Avni, Y. (1998). Translocated Plio-Pleistocene drainage systems along the Arava fault of the Dead Sea transform. Tectonophysics, 284, 151–160.
- Girdler, R. W. (1990). The Dead Sea transform fault system. Tectonophysics, 180, 1–13.
- Gomez, F., Meghraoui, M., Darkal, A. N., Hijazi, F., Mouty, M., Suleiman, Y., … Barazangi, M. (2003). Holocene faulting and earthquake recurrence along the Serghaya branch of the Dead Sea Fault system in Syria and Lebanon. Geophysical Journal International, 153, 658–674.
- Guidoboni, E., & Comastri, A. (2005). Catalogue of earthquakes and tsunamis in Mediterranean area from the 11th to the 15th century (1037 p.). Instituto Nazionale di Geofisica e Vulconagia Rome.
- Guidoboni, E., Comastri, A., & Traina, G. (1994). Catalogue of ancient earthquakes in the Mediterranean area up to the 10th century (504 p.). Instituto Nazionale di Geofisica Rome.
- Gülen, L., Barka, A., & Toksöz, M. N. (1987). Continental collision and related complex deformation: Maraş triple junction and surrounding structures, SE Turkey. Yerbilimleri, 14, 319–336.
- Hall, J., Aksu, A. E., Calon, T. J., & Yaşar, D. (2005). Varying tectonic control on basin development at an active microplate margin: Latakia basin, eastern Mediterranean. Marine Geology, 221, 15–60.
- Hempton, M. R. (1987). Constraints on Arabian plate motions; an extensional history of the Red Sea. Tectonics, 6, 668–705.
- Inwood, J., Anderson, M. W., Morris, A., & Robertson, A. H. F. (2009). Successive structural events in the Hatay ophiolite of southeast Turkey: Distinguishing oceanic, emplacement and post-emplacement phases of faulting. Tectonophysics, 473, 208–222.
- Johannes, D. (2000). Stereonet (2.46). Shareware software. http://homepage.ruhr-uni-bochum.de/Johannes.P.Duyster/Stereo/Stereo1.html
- Karabacak, V., & Altunel, E. (2013). Evolution of the northern Dead Sea Fault Zone in southern Turkey. Journal of Geodynamics, 65, 282–291.
- Karabacak, V., Altunel, E., Meghraoui, M., & Akyüz, H. S. (2010). Field evidences from northern Dead Sea Fault Zone (south Turkey): New findings for the initiation age and slip rate. Tectonophysics, 480, 172–182.
- Karakuş, K., & Taner, G. (1994). Palaeoecological properties and age of the Samandağ Formation (Antakya Basin) based on mollusca fauna. Geological Bulletin of Turkey, 37, 87–109.
- Karig, D. E., & Kozlu, H. (1990). Late Palaeogene-Neogene evolution of the triple junction region, near Maraş, south central Turkey. Journal of the Geological Society London, 147, 1023–1034.
- Kaya, S., & Kıyılı, R. (2009). Antakya’da ortaçağda meydana gelen doğal afet ve salgın hastalıklara bir bakış. [Contagious diseases and natural disasters that occurred in Atioch in the Medieval Ages]. Mustafa Kemal Üniversitesi Sosyal Bilimler Enstitusu Dergisi, 6, 403–419. Hatay.
- Keller, E. A., & Pinter, N. (2002). Active Tectonics: Earthquakes, uplift and landscape (2nd ed., 362 p.). Upper Saddle River, NJ: Prentice Hall.
- Khair, K., Krakaisis, G. F., & Papadimitriou, E. E. (2000). Seismic zonation of the Dead Sea transform fault area. Annali Di Geofisika, 43, 61–79.
- Klinger, Y., Avouac, J. P., Karaki, N. A., Dorbath, L., Bourles, D., & Reyss, J. L. (2000). Slip rate on the Dead Sea transform fault in northern Araba valley (Jordan). Geophysical Journal International, 142, 755–768.
- Kocaçiftçi, P., & Ünlügenç, U. C. (2008). Antakya ve yakın civarının tektonostratigrafisi. Geosound, 52, 157–177.
- Kuhn, S. L., Stiner, M. C., Güleç, E., Özer, I., Yılmaz, H., Baykara, I., … Suata-Alpaslan, F. (2009). The early Upper Paleolithic occupations at Üçağızlı Cave (Hatay, Turkey). Journal of Human Evolution, 56, 87–113.
- Le Pichon, X., & Angelier, J. (1979). The Hellenic arc and trench system: A key to the neotectonic evolution of the eastern Mediterranean area. Tectonophysics, 60, 1–42.
- Le Pichon, X., Lybéris, N., Angelier, J., & Renard, V. (1982). Strain distribution over the east Mediterranean ridge: A synthesis incorporating new Sea-Beam data. Tectonophysics, 86, 243–274.
- Lybéris, N. (1988). Tectonic evolution of the Gulf of Suez and the Gulf of Aqaba. Tectonophysics, 153, 209–220.
- Lybéris, N., Yürür, T., Chorowicz, J., Kasapoğlu, E., & Gündoğdu, N. (1992). The East Anatolian Fault: An oblique collision belt. Tectonophysics, 204, 1–15.
- Mahmoud, Y., Masson, F., Meghraoui, M., Çakır, Z., Alchalbi, A., Yavaşoğlu, H., … İnan, S. (2012). Kinematic study at the junction of the East Anatolian Fault and the Dead Sea Fault from GPS measurements. Journal of Geodynamics. Retrieved from May 18, 2012. doi:10.1016/j.jog.2012.05.006
- Makris, J., Stacker, J., & Kramvis, S. (2000). Microseismic studies and tectonic implications of Cyprus. In I. Panayides, C. Xenophontos, & J. Malpas (Eds.), Proceedings of the Third International Conference on the Geology of the Eastern Mediterranean (pp. 137–145). Geological Survey Department, Republic of Cyprus.
- Mantovani, E., Viti, M., Babbucci, D., Tamburelli, C., & Albarello, D. (2006). Geodynamic connection between the indentation of Arabia and the Neogene tectonics of the central-eastern Mediterranean region. In Y., Dilek & S., Pavlides (Eds.), Post-collisional tectonics and magmatism in the Mediterranean region and Asia (pp. 15–49). Boulder, CO: Geological Society of America Special Paper. 490.
- Marco, S., Agnon, A., Ellenblum, R., Eidelman, A., Basson, U., & Boas, A. (1997). 817-year-old walls offset sinistrally 2.1 m by the Dead Sea transform, Israel. Journal of Geodynamics, 24, 11–20.
- Masson, F., Mahmood, Y., Çakır, Z., Yavaşoğlu, H., Meghraoui, M., Alchalbi, A., … Ergintav, S. (2010). GPS characterization of the triple junction between Arabia, Africa and Anatolia: New measurements in SE Turkey and in NW Syria. WEGENER 2010 15th General Assembly of Wegener. Programme and Book of Abstracts. September 14-17, 2010, Geodesy Department of Kandilli Observatory and Earthquake Research Institute of Bogazici University, Istanbul, Turkey, 47.
- McClusky, S., Reilinger, R., Mahmoud, S., Ben Sari, D., & Tealeb, A. (2003). GPS constraints on Africa (Nubia) and Arabia plate motions. Geophysical Journal International, 155, 126–138.
- McKenzie, D. (1970). Plate tectonics of the Mediterranean region. Nature, 226, 239–243.
- McKenzie, D. (1972). Active tectonic of the Mediterranean region. The Geophysical Journal of the Royal Astronomical Society, 30, 109–185.
- Meghraoui, M., Çakır, Z., Masson, F., Mahmood, Y., Ergintav, S., Alchalbi, A., … Altunel, E. (2011). Kinematic modelling at the triple junction between the Anatolian, Arabian, African plates (NW Syria and in SE Turkey). Geophysical Research Abstracts, 13 , EGU2011-12599.
- Meghraoui, M., Gomez, F., Sbeinati, R., Woerd, J., Mouty, M., Darkal, A. N., … Barazangi, M. (2003). Evidence for 830 years of seismic quiescence from palaeoseismology, archaeoseismology and historical seismicity along the Dead Sea Fault in Syria. Earth and Planetary Science Letters, 210, 35–52.
- Meulenkamp, J. E., Wortel, M. J. R., van Wamel, W. A., & Hoogerduyn Strating, E. (1988). On the Hellenic subduction zone and the geodynamic evolution of Crete since the late Middle Miocene. Tectonophysics, 146, 203–215.
- Michael, A. J. (1984). Determination of stress from slip data: Faults and folds. Journal of Geophysical Research, 89, 11517–11526.
- Nazik, A. (2004). Planktonic foraminiferal biostratigraphy of the Neogene sequence in the Adana Basin, Turkey, and its correlation with standard biozones. Geological Magazine, 141, 379–387.
- Öner, E. (2008). Alluvial geomorphology and paleogeographical studies on the Asi (Orontes) delta plain. Aegean Geographical Journal, 17(1–2), 1–25.
- Över, S., Kavak, K. Ş., Bellier, O., & Özden, S. (2004). Is the Amik Basin (SE Turkey) a triple-junction area? Analyses of SPOT XS imagery and seismicity. International Journal of Remote Sensing, 25, 193857–193872.
- Över, S., Ünlügenç, U. C., & Bellier, O. (2002). Quaternary stress regime change in the Hatay region (SE Turkey). Geophysical Journal International, 148, 1–14.
- Papazachos, B. C., & Papaioannou, Ch. A. (1999). Lithospheric boundaries and plate motions in the Cyprus area. Tectonophysics, 308, 193–204.
- Parlak, O., Karaoğlan, F., Klötzli, U., Thöni, M., Koller, F., & Bağcı, U. (2009). Isotope geochronology and geochemistry of the Kızıldağ (Hatay) ophiolite, southern Turkey: evidence for crystallization age of oceanic crust and distinct magma genesis in southern Neotethyan ocean. 62nd Geological Kurultai of Turkey, April 13−17, 2009, MTA–Ankara, Türkiye, 838–839.
- Parlak, O., Kop, A., Ünlügenç, U. C., & Demirkol, C. (1998). Geochronology and geochemistry of basaltic rocks in the Karasu graben around Kırıkhan (Hatay), S. Turkey. Turkish Journal of Earth Sciences, 7, 53–61.
- Perinçek, D., & Çemen, İ. (1990). The structural relationship between the East Anatolian and Dead Sea Fault Zones in southeastern Turkey. Tectonophysics, 172, 331–340.
- Pirazzoli, P. A., Laborel, J., Saliège, J. F., Kayan, I., & Person, A. (1991). Holocene raised shorelines on the Hatay coast (Turkey): Palaeoecological and tectonic implications. Marine Geology, 96, 295–311.
- Quennell, A. M. (1958). The structural and geomorphic evolution of the Dead Sea rift. Quarterly Journal of the Geological Society of London, 114, 1–24.
- Quennell, A. M. (1984). The western Arabia rift system. Geological Society of London, Special Publications, 17, 775–788.
- Reilinger, R., McClusky, S., Vernant, P., Lawrence, S., Ergintav, S., Çakmak, R., … Karam, G. (2006). GPS constraints on continental deformation in the Africa-Arabia-Eurasia continental collision zone and implications for the dynamics of plate interactions. Journal of Geophysical Research, 111, B05411, 1–26.
- Rojay, B., Heimann, A., & Toprak, V. (2001). Neotectonic and volcanic characteristics of the Karasu fault segment (Anatolia, Turkey): the transition zone between the Dead Sea transform and the East Anatolian Fault Zone. Geodinamica Acta, 14, 197–212.
- Şafak, Ü. (1993a). Antakya Havzası planktonik foraminifer biyostratigrafisi [Planctonic foraminifera biostratigraphy of the Antakya Basin]. Suat Erk Jeoloji Sempozyumu Bildirileri Proceedings of Suat Erk Symposium, Ankara, 143–156.
- Şafak, Ü. (1993b). Antakya havzası ostrakod biyostratigrafisi [Ostracoda biostratigraphy of the Antakya basin]. Türkiye Jeoloji Bülteni, 36, 115–137.
- Sbeinati, M. R., Darawcheh, R., & Mouty, M. (2005). The historical earthquakes of Syria: An analysis of large and moderate earthquakes from 1365 B.C. to 1900 A.D. Annals of Geophysics, 48, 347–435.
- Schmidt, G. C. (1961). Stratigraphic nomenclature for the Adana region petroleum district VII. Petroleum Administration Bulletin, 6, 47–63.
- Selçuk, H. (1981). Étude géologique de la partie meridional du Hatay, Turquie (PhD thesis) [Geological study of southern Hatay, Turkey] Thesis No. 1977, Université de Genévé, France. 116 p.
- Selçuk, H. (1985). Geology and geodynamic evolution of Kızıldağ-Keldağ-Hatay area (Report No. 7787). Unpublished Internal Report of Mineral Research Institute of Turkey, Ankara.
- Şengör, A. M. C. (1979). Türkiye’nin neotektonik esasları [Neotectonics of Turkey] (Vol. 2, 40 p.). Ankara: Türkiye Jeoloji Kurumu Yayınları Serisi.
- Şenyürek, M., & Bostancı, E. (1958). Hatay vilayetinin Paleolitik Kültürleri [Palaeolithic cultures of Hatay Province]. Belleten (Ankara) XXII, 171–210.
- Steinz, G., & Bartov, Y. (1991). The Miocene-Pleistocene history of the Dead Sea segment of the rift in the light of K-Ar ages of basalts. Israel Journal of Earth Science, 40, 199–208.
- Tatar, O., Piper, J. D. A., Gürsoy, H., Heimann, A., & Koçbulut, F. (2004). Neotectonic deformation in the transition zone between the Dead Sea transform and the East Anatolian Fault Zone, southern Turkey: A palaeomagnetic study of the Karasu Rift volcanism. Tectonophysics, 385, 17–43.
- Tekeli, O., & Erendil, M. (1986). Geology and petrology of the Kızıldağ ophiolite (Hatay). Bulletin of the Mineral Research and Exploration Institute of Turkey, 107, 21–37.
- Ten Dam, A. (1952). Facies and stratigraphy in the southern part of the Neogene Basin of İskenderun. Geological Bulletin of Turkey, 3, 47–64.
- Ternek, Z. (1957). The Lower Miocene (Burdigalian) formations of the Adana Basin, their relations with other formations, and oil possibilities. Bulletin of Mineral Research Institute of Turkey, 49, 60–80.
- Tüysüz, O., Genç, Ş.C., İmren, C., & Tarı, U. (2012). Asi nehri ve Samandağ kıyılarındaki nehir ve deniz taraçaları ile bunların güneydoğu Anadolu’nun neotektoniğindeki yeri. TÜBİTAK ÇAYDAG 109Y128, Open Project Report, 306 p. Retrieved from http://uvt.ulakbim.gov.tr/uvt/index.php?cwid=3&vtadi=TPRJ&ts=1376158191&keyword=T%DCYS%DCZ%2C%20Okan&s_f=3&page=2&detailed=1
- Vidal, N., Alvarez-Marón, J., & Klaeschen, D. (2000). The structure of the Africa–Anatolia plate boundary in the eastern Mediterranean. Tectonics, 19, 723–739.
- Wdowinski, S., Ben-Avraham, Z., Arvidsson, R., & Ekstrom, G. (2006). Seismotectonics of the Cyprian Arc. Geophysical Journal International, 164, 176–181.
- Westaway, R. (2003). Kinematics of the Middle East and eastern Mediterranean updated. Turkish Journal of Earth Sciences, 12, 5–46.
- Westaway, R. (2004). Kinematic consistency between the Dead Sea Fault Zone and the Neogene and Quaternary left-lateral faulting in SE Turkey. Tectonophysics, 391, 203–237.
- Yetiş, C. (1988). Reorganization of the Tertiary stratigraphy in the Adana Basin, southern Turkey. Newsletters in Stratigraphy, 20, 42–58.
- Yetiş, C., Kelling, G., Gökçen, S., & Baroz, F. (1995). A revised stratigraphic framework for Later Cenozoic sequences in the northeastern Mediterranean region. Geologische Rundschau, 84, 794–812.
- Yılmaz, Y., Gürpınar, O., & Yiğitbaş, E. (1988). Tectonic Evolution of the Miocene Basins at the Amanos Mountains and the Maraş Region. Turkish Association of Petroleum Geologists Bulletin, 1, 52–72.
- Yürür, M. T., & Chorowicz, J. (1998). Recent volcanism, tectonics and plate kinematics near the junction of the African, Arabian and Anatolian plates in the eastern Mediterranean. Journal of Volcanology and Geothermal Research, 85, 1–15.