Abstract
Palaeo- and Neo-Tethyan-related magmatic and metamorphic units crop out in Konya region in the south central Anatolia. The Neotethyan assemblage is characterized by mélange and ophiolitic units of Late Cretaceous age. They tectonically overlie the Middle Triassic–Upper Cretaceous neritic to pelagic carbonates of the Tauride platform. The metamorphic sole rocks within the Konya mélange crop out as thin slices beneath the sheared serpentinites and harzburgites. The rock types in the metamorphic sole are amphibolite, epidote-amphibolite, garnet-amphibole schist, plagioclase-amphibole schist, plagioclase-epidote-amphibole schist and quartz-amphibole schist. The geochemistry of the metamorphic sole rocks suggests that they were derived from the alkaline (seamount) and tholeiitic (E-MORB, IAT and boninitic type) magmatic rocks from the upper part of the Neotethyan oceanic crust. Four samples from the amphibolitic rocks yielded 40Ar/39Ar isotopic ages, ranging from 87.04 ± .36 Ma to 84.66 ± .30 Ma. Comparison of geochemistry and geochronology for the amphibolitic rocks suggests that the alkaline amphibolite (seamount-type) cooled below 510 ± 25 °C at 87 Ma whereas the tholeiitic amphibolites at 85 Ma during intraoceanic thrusting/subduction. When all the evidence combined together, the intraoceanic subduction initiated in the vicinity of an off-axis plume or a plume-centered spreading ridge in the Inner Tauride Ocean at 87 Ma. During the later stage of the steady-state subduction, the E-MORB volcanics on the top of the down-going slab and the arc-type basalts (IAT/boninitic) detached from the leading edge of the overriding plate, entered the subduction zone after ~2 my and metamorphosed to amphibolite facies in the Inner Tauride Ocean. Duration of the intraoceanic detachment (~87 Ma) and ophiolite emplacement onto the Tauride-Anatolide Platform (Tavşanlı Zone), followed by subsequent HP/LT metamorphism (~82 Ma) spanned ~5 my in the western part of the Inner Tauride Ocean.
Introduction
Konya region in south central Anatolia forms one of the important locations, where both magmatic and metamorphic units resulted from the Palaeotethyan and Neotethyan evolution can be observed. The remnants of Palaeotethyan crop out in the N–NW part of Konya city and are characterised by pre-Permian tectono-stratigraphic/magmatic units (Eren, Citation1993; Eren, Kurt, Rosselet, & Stampfli, Citation2004; Göncüoğlu et al., Citation2007; Özcan, Göncüoğlü, Turhan, Uysal, & Şentürk, Citation1988; Özcan et al., Citation1990; Robertson & Ustaömer, Citation2009, Citation2011). The Palaeozoic rock assemblage is unconformably covered by Permian and Triassic sedimentary units (Göncüoğlu et al., Citation2007; Özcan et al., Citation1988; Robertson & Ustaömer, Citation2009). These rocks are interpreted as a part of the Afyon–Bolkardağ Zone (Okay, Citation1986) or the Kütahya–Bolkardağ belt (Göncüoğlu, Dirik, & Kozlu, Citation1996–1997), which experienced Alpine HP–LT metamorphism (Candan, Çetinkaplan, Oberhänsli, & Rimmelé, Citation2005; Davis & Whitney, Citation2006; Okay, Citation1986; Pourteau, Candan, & Oberhänsli, Citation2010; Pourteau et al., Citation2013; Sherlock, Kelley, Inger, Harris, & Okay, Citation1999) (Figure ), whereas the Neotethyan units crop out mainly in the southwestern part of the Konya city. They are dominated by mélange and ophiolitic units of Late Cretaceous age (Figure ).
Figure 1. Outline tectonic map of Turkey showing the study area (taken from Robertson & Ustaömer, Citation2009; tectonic zones are from Okay & Tüysüz, Citation1999).
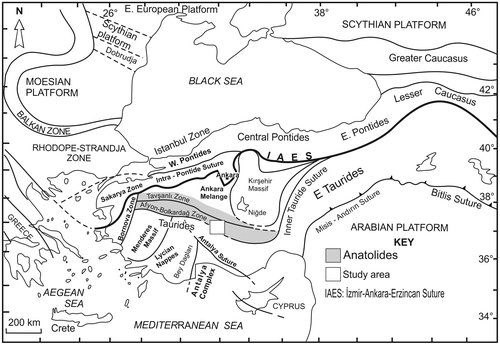
Figure 2. Outline geological map (modified from MTA, Citation2002) showing the Palaeotethyan and Neotethyan units around Konya (taken from Robertson & Ustaömer, Citation2009).
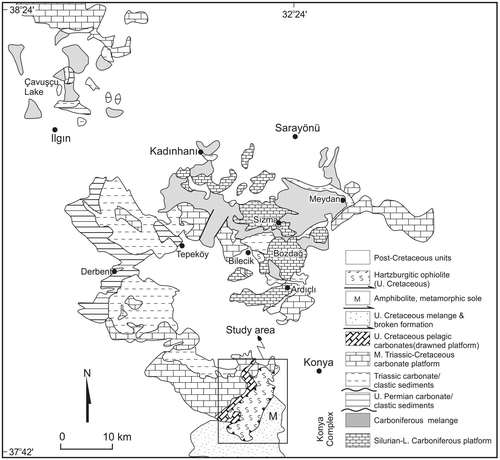
The harzburgite blocks in the mélange to the SW of Konya locally include metamorphic sole at its base. Initiation of subduction and formation of metamorphic soles have been linked to the ophiolite emplacement process (Hacker, Mosenfelder, & Gnos, Citation1996; Jamieson, Citation1980, Citation1986; Malpas, Citation1979; McCaig, Citation1983; Wakabayashi, Ghatak, & Basu, Citation2010; Williams & Smyth, Citation1973). The Tethyan ophiolites are structurally underlain by thin sheets of high-grade metamorphic sole rocks (Çelik, Citation2002; Dilek, Thy, Hacker, & Grundvig, Citation1999; Jamieson, Citation1986; Parlak & Delaloye, Citation1999; Parlak et al., Citation1995, Citation2006; Robertson, Citation2002, 2004; Spray, Citation1984; Vergili & Parlak, Citation2005; Williams & Smyth, Citation1973). Protoliths of the metamorphic soles in Anatolia suggest the presence of both tholeiitic and alkaline magma types from various tectonic settings, such as OIB, MORB and IAB (Çelik, Citation2002; Çelik & Delaloye, Citation2003; Elitok & Drüppel, Citation2008; Lytwyn & Casey, Citation1995; Parlak, Citation1996; Parlak et al., Citation1995, Citation2006; Polat, Casey, & Kerrich, Citation1996; Vergili & Parlak, Citation2005). The 40Ar/39Ar ages of the metamorphic soles from the Tauride ophiolites range from 96 Ma to 88 Ma (Çelik, Delaloye, & Feraud, Citation2006; Dilek et al., Citation1999; Parlak & Delaloye, Citation1999; Parlak et al., Citation2013), whereas the metamorphic sole rocks beneath the ophiolites from the İzmir-Ankara–Erzincan suture zone and its eastward prolongation display variable 40Ar/39Ar ages, ranging from 101 Ma to 93 Ma (Harris, Kelley, & Okay, Citation1994; Önen, Citation2003) in NW Anatolia, 177 Ma to 167 Ma in NE Anatolia (Çelik et al., Citation2011) and 90 Ma in NW of Sevan–Akera suture zone (Hassig et al., 2011).
The timing of HP–LT metamorphism of the leading edge of the Anatolides is well constrained in the Tavşanlı Zone from 82.8 Ma to 79.7 Ma based on Rb–Sr dating of phengite (Sherlock et al., Citation1999). Exhumed leading edge of the Anatolides and emplaced ophiolites were juxtaposed by Late Maastrichtian to Middle Paleocene as these units were unconformably covered by shallow water sediments (Robertson, Parlak, & Ustaömer, Citation2009).
The scope of this paper is (1) to present petrography and geochemistry of the metamorphic sole rocks and hence to investigate possible protoliths of the accreted material to the base of mantle tectonites during intraoceanic thrusting, (2) to give new 40Ar–39Ar age dating of the metamorphic sole rocks and (3) to constrain about the time gap between the intraoceanic subduction and the continental margin subduction during Neotethyan convergence.
Geological setting and petrography
The allochthonous and autochthonous rock assemblages in Konya region are interpreted as part of the Afyon–Bolkardağ Zone (Okay, Citation1986) or the Kütahya–Bolkardağ belt (Göncüoğlu et al., Citation1996–1997). The Palaeotethyan units are dominated by a dismembered, latest Silurian–Early Carboniferous carbonate platform (Bozdağ massif), a Carboniferous mélange made up of sedimentary and igneous blocks in a sedimentary matrix (Halıcı Group or Sızma Group), and an overlying earliest Permian volcanic–sedimentary unit (Eren, Citation1993; Eren et al., Citation2004; Özcan et al., Citation1988, Citation1990; Robertson & Ustaömer, Citation2009, Citation2011) (Figure ). There are number of interpretations about the geodynamics of this assemblage such as (a) an active continental margin bordering the northern margin of Gondwana (Eren & Kurt, Citation2000), (b) an Early Carboniferous back-arc rift along the northern margin of Eurasia (Göncüoğlu et al., Citation2007), (c) a fore-arc basin related to northward subduction beneath Eurasia (Eren et al., Citation2004) and (d) a Carboniferous accretionary complex related to southward subduction beneath Gondwana (Robertson & Ustaömer, Citation2009, Citation2011). The Neotethyan assemblages (mélange and ophiolite) tectonically overlies the Middle Triassic–Upper Cretaceous neritic (Loras limestone) to pelagic (Midos Tepe formation) carbonates of the Tauride platform (Özcan et al., Citation1990) (Figures and ). The Loras limestone is exposed in Loras mountain in the NW of the study area and generally characterised by medium-to-hick-bedded neritic limestone and dolomitic limestone in a lesser extend (Figure (a) and (b)). Based on the fossil evidence, it is Middle Triassic to Cretaceous in age (Özcan et al., Citation1988). To the west of Konya, the Loras limestone is transitionally followed by the Midos Tepe formation that comprises thin-bedded red pelagic limestones, abundant cherty limestone and radiolarian mudstone in the upper level (Figure (c)). This unit is Maastrichtian in age (Özcan et al., Citation1988). The Tauride Platform is tectonically overlain either by mélange or peridotite body (Figure ). The mélange unit has both sedimentary and sheared serpentinite matrix in which large blocks of limestones ranging in age from Carboniferous to Late Cretaceous, radiolarian cherts, mudstone and ophiolitic rocks (ultramafic rocks, volcanics, gabbro) are tectonically dispersed (Figure (e)–(h)). The mélange unit is structurally overlain by an ophiolite body that is characterized by serpentinised harzburgite with economically important hydrothermal magnesite deposits, dunite, pyroxenite and gabbro (Figure (b)–(d)).
Figure 3. Geological map of the Neotethyan units to the southwest of Konya (from Özcan et al., Citation1990). Cross section showing the tectonic relations of the platform and oceanic assemblages in the study area.
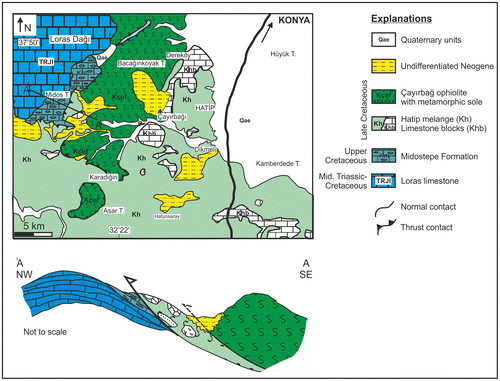
Figure 4. Field views of rock units in the platform and mélange. Loras limestone (a), tectonic relation between Loras limestone and peridotite body (b), pelagic carbonates (Midostepe formation) on the top of the platform (c), serpentinised harzburgite with magnesite veins (d), limestone blocks in the mélange (e), radiolarian cherts and mudstone in the mélange (f), sheared serpentinite in the mélange (g), and volcanogenic sandstone in the mélange (h).

Large harzburgite blocks (Figure (a)) within the mélange locally include an attached metamorphic sole (Daşçı, Citation2007). The metamorphic sole can be observed along a road section between Çayırbağ and Karadiğin villages (Figure ). The metamorphic sole has ~35 m thickness. The contact between the metamorphic sole and the overlying peridotite is defined by a 2–3-m-thick strongly sheared serpentinite above and amphibolite schist below (Figure ). The foliation patterns in the serpentinite and the metamorphic sole are concordant, suggesting that the contact can be interpreted as representing the initial intra-oceanic decoupling surface (Spray, Citation1984) (Figures and (b)). The metamorphic sole include the following rock association: amphibolite, epidote-amphibole schist, garnet-amphibole schist, amphibole schist, plagioclase-amphibole schist, plagioclase-epidote-amphibole schist and quartz-amphibole schist (Figure ). The mineral assemblages in the metamorphic sole rocks are given in Table . The metamorphic sole rocks exhibit granoblastic and nematoblastic textures. They display well-developed foliation due to the preferred orientation of hornblende and plagioclase (Figure (d) and (e)). Amphibolitic metamorphic soles consist of hornblende-dominated dark layers that alternate with light bands, lenses and stringers composed of plagioclase, epidote and minor quartz (Figure (d)–(f)). The amphiboles are the most common phases in the metamorphic sole rocks and represented by euhedral to subhedral hornblendes (65–85%). The plagioclase (15–20%) exhibits strong alteration products including calcite, albite and sericite. The garnets (15–20%) occur as porphyroblasts and are accompanied by hornblende in high-grade rocks (Figure (c)). The actinolite is represented by euhedral to subhedral crystals and very common with pronounced needle-like crystals in greenschist facies rocks. The epidote and zoisite are common in lower amphibolite and greenschist facies rocks of the metamorphic sole. The epidote also occurs as veins. Sphene, rutile and opaque minerals are the accessory phases.
Figure 5. Tectonic relation between the sheared serpentinite and metamorphic sole rocks in Karadiğin village.
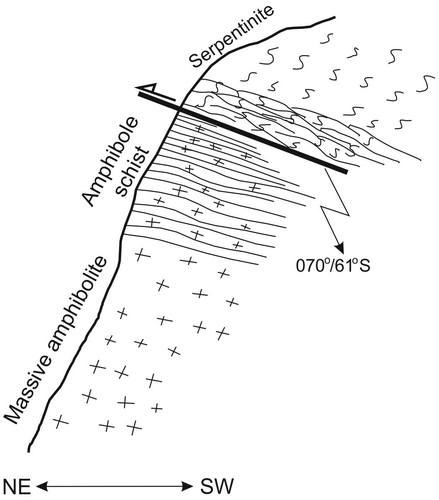
Figure 6. Rock units in the metamorphic sole and overlying peridotite. Serpentinized harzburgite (a), tectonic contact between the metamorphic sole and the serpentinite (b), garnet-bearing amphibolite (c), plagioclase-amphibole schist (d), amphibolite schist (e), and epidote-amphibole schist (f).
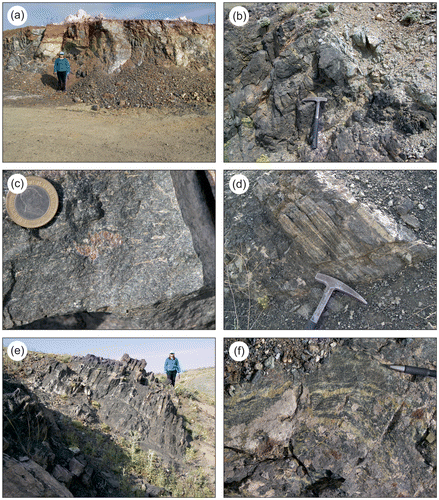
Table 1. Mineral assemblages in the metamorphic sole rocks from the Konya mélange, Turkey.
Analytical Method
A total of 27 samples from the metamorphic sole rocks were analysed for major and trace (including rare earths) elements in Acme Analytical Laboratories Ltd. in Canada. Major element contents were determined from a LiBO2 fusion by ICP-ES by using five grams of sample pulp. Trace element contents were determined from a LiBO2 fusion by ICP-MS by using five grams of sample pulp. The results of the analyses are presented in Tables and . 40Ar/39Ar geochronology was carried out on handpicked hornblende grains from amphibolites of the metamorphic sole at The Auburn Noble Isotope Mass Analysis Laboratory (ANIMAL).
Table 2. Major and trace element analyses of the metamorphic sole rocks (Group I & II) from the Konya mélange, Turkey.
Table 3. Major and trace element analyses of the metamorphic sole rocks (Group III & IV) from the Konya mélange, Turkey.
Four amphibolite samples were collected for 40Ar/39Ar geochronology from four different outcrops of metamorphic sole. The samples were selected on the basis of structural setting and petrography.
Selected four amphibolite samples were crushed and sieved (250–180), and hornblende grains were handpicked under the binocular microscope to be free from visible alteration, inclusion or other phases. The selected samples were washed with de-ionized water in ultrasonic cleaner. Around 300 amphibole grains were individually wrapped in domestic Al-foil and placed in Al-irradiation disk with monitor FC-2 (age = 28.02 Ma., Renne et al., 1998) along with CaF2 flux monitor. All samples and standards were irradiated in McMaster nuclear reactor facility in Hamilton, Ontario, Canada. The laboratory is equipped with a low-volume, high-sensitivity 10-cm radius sector mass spectrometer and automated sample extraction system (Based 50 W Synrad CO2 laser) for the analysis of samples. The analyses were conducted by incremental heating analysis (IH) of 80 hornblende grains from each sample. All of the statistical 40Ar/39Ar ages in this study are quoted at the 95% confidence level, whereas errors in individual measurements are quoted as one standard deviation (1σ). Data reduction and assessment of statistical ages were done using Microsoft Excel and Isoplot (Ludwig, Citation2003). The full data-set for this study is available in the data repository (Table ).
Table 4. 40Ar/39Ar analytical data for the measured sample.
Geochemistry
The major and trace (including rare earths) element contents of the metamorphic sole rocks from the Konya mélange are presented in Tables and . Most of the metabasic rocks (amphibolite) show varying degrees of alteration from greenschist to amphibolite facies in the studied samples. Even mild alteration may cause selected element mobility, especially involving the large ion lithophile (LIL) elements (e.g. Na, K, Rb, Sr and Ba) (Thompson, Citation1991). To test element mobility on the studied samples, Sr and Ba are plotted against Zr in Figure . These elements display non-systematic scattered distribution, reflecting their mobility. Therefore, characterization and discrimination of the metamorphic sole rocks (amphibolites) have been done on the basis of trace elements generally considered as relatively stable (immobile) during alteration, such as the high field strength (HFS) elements and rare earth elements (Floyd & Winchester, Citation1978; Pearce & Cann, Citation1973; Smith & Smith, Citation1976).
Figure 7. Zr vs. Sr (a) and Ba (b) diagrams for the metamorphic sole rocks, showing the chemical alteration effects.
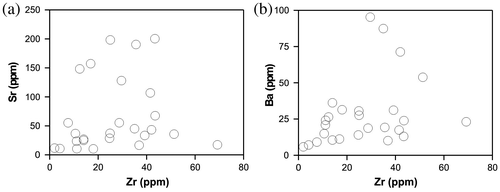
Overall, the geochemistry of the metamorphic sole rocks from the Konya mélange displays a wide range of chemical variation, which can be grouped into four main magma series: Group I (alkaline serie), Group II (main tholeiitic serie), Group III (enriched tholeiitic serie) and Group IV (low-Ti tholeiitic serie). All the geochemical groups show that the amphibolitic metamorphic sole rocks were derived from an igneous protolith based on the Zr/Ti vs. Ni contents (Figure (a)). On the basis of stable incompatible element ratios (Nb/Y vs Zi/Ti) (after Pierce, Citation1996), the amphibolitic sole rocks represent exclusively metamorphosed tholeiitic basalts, except one sample (A-8) in meta-alkali basalt field (Figure (b)). Different petrogenetic groups are well defined by selected major and trace elements in Figure . The FeO*/MgO vs. Zr diagram displays a Fe-enrichment trend for the tholeiitic and alkaline groups, resulted largely by mafic fractionation during their genesis (Figure (a)). The variations of Y and Ti against Zr show linear positive correlations for the tholeiitic meta-basic rocks (Group II to IV) and differ from the alkaline one (Group I) (Figure (b) and (c)). The TiO2 vs. V diagram in Figure (d) differentiate Group I alkaline serie (Ti/V = 67.49), Group II main tholeiitic serie (Ti/V = 11.38–31.42), Group III enriched tholeiitic serie (Ti/V = 24.04–37.13) and Group IV low-Ti tholeiitic serie (Ti/V = 7.82–12.86).
Figure 8. (a) Zr/TiO2–Ni diagram, demonstrating igneous origin of the metamorphic sole rocks (after Winchester, Park, & Holland, Citation1980). (b) Zr–Ti vs. Nb–Y rock classification diagram for the metamorphic sole rocks (after Pierce, Citation1996).
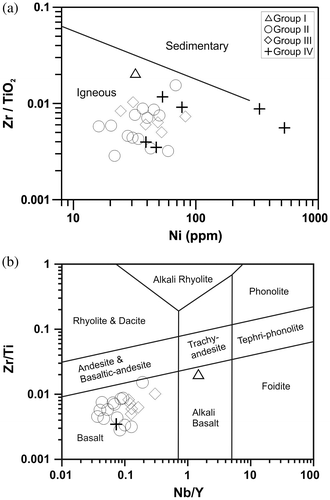
Ratio/ratio plots of incompatible elements were used in Figure to characterize mantle source regions for the protoliths of the metamorphic sole rocks from the Konya mélange. Comparative trace element ratios for different geochemical groups of studied rocks were listed with those of MORB’s, ocean island basalts (OIB) and Vanuatu arc lavas in Table . The Y/Nb, Y/Ta, Zr/Nb and Ti/Nb ratios of the metamorphic sole rocks suggest that Group I (alkaline serie) is similar to ocean island basalt (OIB) and Group III (enriched tholeiitic serie) is comparable to enriched mid-ocean ridge basalt (E-MORB), whereas Group II (main tholeiitic serie) and Group IV (low-Ti tholeiitic serie) are more akin to normal mid-ocean ridge basalt (N-MORB) (Figure (a) and (b)). The Ce/Sm vs. Sm/Yb ratios are plotted in Figure (c). The high Sm/Yb and Ce/Sm ratios of the alkaline amphibolite sample (Group I) suggest that it was derived by melting of an OIB-like enriched mantle source, whereas the low Sm/Yb and Ce/Sm ratios of the main tholeiitic serie amphibolites (Group II) and low-Ti tholeiitic serie (Group IV) suggest derivation from a more depleted MORB-like mantle source. The enriched tholeiitic serie amphibolites (Group III) are mainly plotted in the middle, suggesting a more enriched mantle source for their protliths. In the same diagram, it is clearly seen that Group II and Group IV amphibolitic rocks show close similarity to the tholeiitic amphibolites beneath the Tauride ophiolites, whereas Group I amphibolite is more akin to the alkaline amphibolites beneath the Tauride ophiolites (Figure (c)). The tholeiitic amphibolites of Group III differ from the metamorphic sole rocks of the Tauride ophiolites.
Figure 10. (a) Y/Nb vs. Y/Ta, (b) Zr/Nb vs. Ti/Nb and (c) Sm/Yb vs. Ce/Sm diagrams, indicating different magma sources for the protoliths of the metamorphic sole rocks. Field of metamorphic soles from the Tauride ophiolites are from Parlak et al. (Citation1995, Citation2006), Lytwyn and Casey (Citation1995), Çelik and Delaloye (Citation2003), Vergili and Parlak (Citation2005).
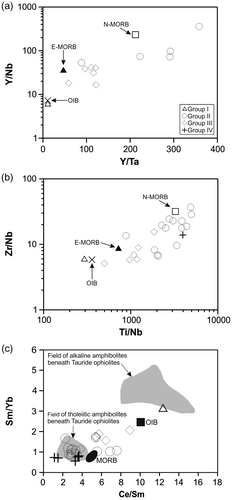
Table 5. Comparative trace element ratios for the metamorphic sole rocks (Group I to IV) with those of N-MORB, E-MORB, OIB (from Sun and McDonough (Citation1989)) and IAT (Vanuatu Arc).
Chondrite-normalized REE patterns for the four magma series from the metamorphic sole rocks of the Konya mélange, together with data from N-MORB, OIB and E-MORB are presented in Figure . The REE concentrations vary from 12 to 159 X condritic for Group I (alkaline amphibolite) rocks. It displays greatest light rare earth element (LREE) enrichment (LaN/YbN = 12.94), showing close similarity to ocean island basaltic rocks (Figure (a)). The REE concentrations vary from 6 to 41 X condritic for Group II (main tholeiitic amphibolites) rocks. They are generally typified by flat normalized patterns with average (LaN/YbN = 1.34), although some have minor LREE depletions and enrichments (Figure (b)). The REE concentrations vary from 10 to 58 X condritic for Group III (enriched tholeiitic amphibolites) rocks. They are more enriched (average LaN/YbN = 2.84) and display variable degrees of enrichment. These rocks are more akin to E-MORB in Figure (c). The REE concentrations vary from .4 to 8 X condritic for Group IV (low-Ti tholeiitic amphibolites) rocks. They are represented by flat REE patterns (average LaN/YbN = 1.45) and very depleted compared to N-MORB (Figure (d)).
Figure 11. Chondrite-normalized REE diagrams for the metamorphic sole rocks (normalizing values are from Sun and McDonough (Citation1989)).
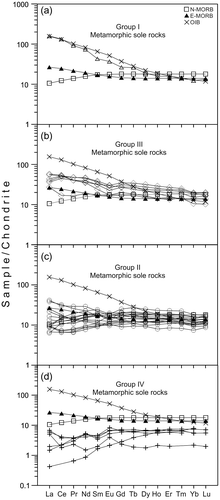
The N-MORB normalized multi-element diagrams of the amphibolitic metamorphic sole rocks from the Konya mélange are presented in Figure , with E-MORB and OIB for comparison. The alkaline amphibolite sample (Group I) in Figure a displays a strong progressive enrichment pattern and has more in common with typical enriched ocean island basalt (OIB) composition (Sun & McDonough, Citation1989). The low-Ti tholeiitic amphibolites (Group IV) show variable, but typically highly depleted patterns compared to N-MORB (Figure (d)). The multi-element diagram of the main tholeiitic amphibolites (Group II) exhibits such features as (a) enrichment in LIL (i.e. Rb, Ba, Th and K), (b) strong negative Nb anomaly, (c) depletion in P and Zr, reflecting effects of zircon and apatite fractionation and (d) flat HFS elements compared to N-MORB (Figure (b)). The enriched tholeiitic amphibolites (Group III) exhibit progressive enrichment patterns and depletion in Sr, P, Zr, Hf and Ti (Figure (c)), showing close similarity to E-MORB (Sun & McDonough, Citation1989). Negative Sr anomalies represent plagioclase fractionation.
Figure 12. N-MORB normalized spider diagrams for the metamorphic sole rocks (normalizing values are from Sun and McDonough (Citation1989)).
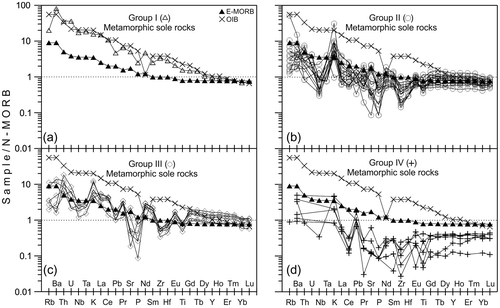
Ar/Ar Geochronology
Four new 40Ar/39Ar ages have been obtained from amphibolites of metamorphic sole. The 40Ar/39Ar age of each sample is considered to reflect the time of metamorphism in the respective blocks with some variation due to slightly different histories. Hornblendes from the metamorphic rocks yield robust 40Ar/39Ar plateau ages (Figure ). The oldest age was obtained from sample A8, resulted in an age of 87.04 ± .36 Ma (Figure (a)). The sample A8 yielded a concordant age as defined by 16 adjacent steps including 97.8% of the total released 39Ar (Figure (a)). Samples A12 and A2 yielded a concordant age of 85.26 ± .19 Ma and 85.62 ± .34 Ma, respectively (Figure (b) and (c)). The age of sample A12 is defined by steps 3 through 21 including 99.78% of total released 39Ar (Figure (c)). The sample A2 yielded a concordant age of 85.62 ± .34 Ma as defined by 20 steps and 100% of the released 39Ar (Figure (b)). The youngest hornblende age (A13) was obtained as 40Ar/39Ar age of 84.66 ± .30 Ma by 97.8% of the 39Ar, steps 1 through 16 (Figure (d)). This sample is slightly, but not significantly younger than other hornblende ages. Calculated Ca/K ratio for each hornblende sample shows that during analyses this ratio was very stable for each sample.
Discussion
Due to the opening of the South Atlantic Ocean (Dewey, Citation1988; Smith, Hurley, & Briden, Citation1981) in Early–Late Cretaceous, rifting ceased and convergence between Afro-Arabian and Eurasia plates began. This compressional tectonic regime caused the initiation of intra-oceanic subduction in the well-established oceanic basins, namely the İzmir-Ankara-Erzincan, the Inner Tauride and the Southern Neotethys. A north-dipping subduction at the beginning of the Late Cretaceous created supra-subduction zone ophiolite bodies in the eastern Mediterranean region (Bağcı, Parlak, & Höck, Citation2005, Citation2006, Citation2008, 2009; Beyarslan & Bingöl, Citation2000; Parlak, Delaloye, & Bíngöl, Citation1996, Parlak, Höck, & Delaloye, Citation2000, Parlak et al., Citation2013; Pearce, Harris, & Tindle, Citation1984; Robertson, Citation2002; Rızaoğlu, Parlak, Höck, & İşler, Citation2006; Robertson, Parlak, & Ustaömer, Citation2013; Yalınız, Floyd, & Göncüoğlü, Citation1996, Citation2000).The intra-oceanic subduction led to emplacement of several ophiolitic nappes with accreted metamorphic soles and mélanges generally from north to south onto continental margins (Al-Riyami, Robertson, Dixon, & Xenophontos, Citation2002; Andrew & Robertson, Citation2002; Dilek & Whitney, Citation1997; Okay et al., Citation2006; Önen & Hall, Citation1993; Parlak & Robertson, Citation2004; Rice, Robertson, & Ustaömer, Citation2006; Rice, Robertson, Ustaömer, İnan, & Taslı, Citation2009; Robertson et al., Citation2009, Citation2013; Rojay, Citation2013; Yılmaz, Citation1993; Yılmaz, Yiğitbaş, & Genç, Citation1993).
It has been widely accepted that initiation of subduction and formation of metamorphic soles have been linked to the ophiolite emplacement process (Hacker et al., Citation1996; Jamieson, Citation1980, Citation1986; Malpas, Citation1979; McCaig, Citation1983; Williams & Smyth, Citation1973). Metamorphic soles are thought to form at the inception of oceanic subduction beneath the hot sub-ophiolitic mantle of the hanging wall (Jamieson, Citation1986; Malpas, Citation1979; Spray, Citation1984; Williams & Smyth, Citation1973). As the metamorphic soles record hot ophiolite emplacement over cold oceanic crust and associated sediments, it is important that the protoliths of the metamorphic soles provide evidence of the nature and composition of former ocean basins (Robertson, Citation2004).
The amphibolitic rocks of the metamorphic sole from the Konya mélange were derived from the metamorphism of the alkaline and tholeiitic ocean floor basalts. The basaltic rocks and the associated sediments were welded and accreted to the base of ophiolite which formed the hanging wall plate of the subduction zone. The geochemical features of the alkaline amphibolite suggest that it was derived from an enriched mantle source and does not exhibit a subduction-zone component (Figures –). Therefore, it is geochemically similar to seamount-type alkaline basalts. Mesozoic seamount-type alkaline basalts were documented extensively along the Neotethyan sutures in Anatolia (e.g. Çelik & Delaloye, Citation2003; Dilek et al., Citation1999; Elitok & Drüppel, Citation2008; Floyd, Citation1993; Lytwyn & Casey, Citation1995; Parlak, Citation2000; Parlak et al., Citation1995, Citation2006; Polat et al., Citation1996; Rojay, Yalınız, & Atıner, Citation2001; Vergili & Parlak, Citation2005), in the Sevan–Akera suture zone in Lesser Caucasus (Rolland et al., Citation2009) and in the Masirah island ophiolite, Oman (Meyer, Mercolli, & Immenhauser, Citation1996). Three different tholeiitic amphibolite rocks from the metamorphic sole are defined such as the main tholeiite, the enriched tholeiite and the low-Ti tholeiite suites. The main tholeiite amphibolitic (Group II) rocks display depletion of Nb (Ta), moderate enrichment of LIL elements and parallel to slightly depleted HFS element patterns compared to N-MORB (Figure ). The negative Nb (Ta) and positive Th anomalies obtained from these rocks indicate a subduction-related tectonic setting for the protoliths. This suggests that island-arc tholeiitic basalts were welded to the base of the over-riding sub-ophiolitic mantle during intraoceanic subduction. Such tholeiitic basaltic volcanic rocks and their metamorphosed equivalents were extensively found in supra-subduction zone-type (SSZ) ophiolites and their subophiolitic metamorphic soles in Turkey (Bağcı & Parlak, Citation2009; Bağcı et al., Citation2008; Çelik, Citation2007; Çelik & Delaloye, Citation2003, Citation2006; Çelik et al., Citation2011; Dilek & Furnes, Citation2009; Dilek & Thy, Citation2009; Dilek et al., Citation1999; Elitok & Drüppel, Citation2008; Parlak, Citation1996; Parlak et al., Citation1995, Citation2006; Parlak et al., Citation2000; Rızaoğlu et al., Citation2006; Vergili & Parlak, Citation2005; Yalınız et al., Citation1996, Citation2000). The enriched tholeiitic amphibolite (Group III) rocks are more akin to E-MORB in terms of rare earth element (REE) and multi-element patterns as well as incompatible element ratios (Figures and ; Table ). This suggests that enriched mid-ocean ridge basaltic rocks were accreted to the base of the overriding sub-ophiolitic mantle during intraoceanic subduction. E-MORB protolith is not common from the metamorphic soles of Turkish ophiolites, except the Pozantı-Karsantı ophiolite (Lytwyn & Casey, Citation1995; Polat et al., Citation1996). The highly depleted nature of the low-Ti tholeiitic amphibolites (Group IV) in terms of TiO2 (.06–.53 wt %) and incompatible trace elements (including REEs) as well as the high MgO (13–21 wt %) contents are quite distinctive compared to other groups (Figures and ; Table ). Hence, Group IV amphibolites can be compatible with the metamorphosed equivalents of the boninitic volcanic rocks in a fore-arc tectonic setting which are documented in many of the eastern Mediterranean ophiolites (Bağcı & Parlak, Citation2009; Bağcı et al., Citation2008; Beccaluva & Serri, Citation1988; Malpas & Langdon, Citation1984; Saccani & Photiades, Citation2004, Citation2005).
The sub-ophiolitic metamorphic sole rocks from the Konya mélange yielded well-defined plateau ages such as 87.04 ± .36 Ma for alkaline seamount-type amphibolite (A8) and 85.62 ± .34 Ma to 84.66 ± .3 Ma for the IAT-type amphibolites (A2, 12 and 13). This suggests that the metamorphic sole rocks cooled below 510 ± 25 °C (Harrison, Citation1981) at 87 to 85 Ma during intraoceanic thrusting/subduction. Several alternative models to explain formation of metamorphic sole and ophiolite obduction have been proposed (Agard et al., 2007, 2014; Robertson, Citation2004; Vaughan & Scarrow, Citation2003). Comparison of protoliths of the metamorphic sole rocks and the 40Ar/39Ar isotopic ages from the Konya mélange suggests that the intra-oceanic subduction initiated in the vicinity of an off-axis plume or a plume-centred spreading ridge in the Inner Tauride Ocean, represented by OIB-type basalts (87.04 ± .36 Ma) because the alkaline amphibolite yielded the older isotopic age than the tholeiitic amphibolites (85.62 ± .34 Ma to 84.66 ± .3 Ma). Similar interpretation has been proposed by Rolland et al. (Citation2009) and Hässig et al. (Citation2011) for the obduction of the Lesser Caucasus Armenian ophiolites along the Sevan-Akera suture zone. During the later stage of the steady-state subduction, a supra-subduction zone (SSZ)-type ophiolite was formed with IAT- and boninitic-type volcanics in the upper part of the overriding plate. The E-MORB volcanics on the top of the down-going slab and the sliced- IAT-/boninitic-type volcanics from the upper plate entered the subduction zone after ~2 my and metamorphosed to amphibolite facies in the Inner Tauride Ocean.
High-precision 40Ar/39Ar ages for the metamorphic sole rocks of the Tauride belt yielded 93.6 ± .8 to 90.7 ± .5 for the Lycian ophiolite, 91.5 ± 1.9 to 90.9 ± 1.3 Ma for the Beyşehir ophiolite, 92.4 ± 1.3 to 90.4 ± .9 Ma for the Pozantı–Karsantı ophiolite, 96.0 ± .7 to 91.0 ± .8 Ma for the Mersin ophiolite and 89.65 ± .97 to 87.49 ± .48 Ma for the Divriği ophiolite (Çelik et al., Citation2006; Dilek et al., Citation1999; Parlak & Delaloye, Citation1999; Parlak et al., Citation2013) and 87.04 ± .36 to 84.66 ± .30 Ma for the Konya mélange. The scatter of the cooling ages for the metamorphic soles is ~11 my although they exhibit very narrow age range for the individual ophiolite body. The age scatter may well suggest that (a) the intraoceanic subduction/thrusting did not start at the same time for all oceanic lithospheric slices that are separated by transform faults before their emplacement onto the Tauride platform or (b) oblique subduction caused this age discrepancy along the Tauride belt ophiolites. Figure presents 40Ar/39Ar ages for the metamorphic soles of the Neotethyan ophiolites in the eastern Mediterranean ophiolites. It is clear that the cooling ages of the metamorphic soles beneath ophiolites from the different suture zones do not show any consistency. The metamorphic sole ages from the İzmir-Ankara-Erzincan and Sevan-Akera suture zones display large discrepancy (90 to 177 Ma), whereas the metamorphic sole ages from the Tauride belt are more consistent and tightened between 96 Ma and 85 Ma (Figure ). If we consider that the formation of metamorphic soles is linked to the ophiolite emplacement process (Hacker et al., Citation1996; Jamieson, Citation1980, Citation1986; Malpas, Citation1979; McCaig, Citation1983; Williams & Smyth, Citation1973), then it is difficult to conclude about simultaneous emplacement processes along several oceanic basins in Anatolia during closure events.
Figure 14. 40Ar/39Ar ages from the metamorphic soles beneath the eastern Mediterranean ophiolites. Data are from 1- Dilek et al. (Citation1999), 2- Parlak and Delaloye (Citation1999), 3- Çelik et al. (Citation2006), 4- Chan, Malpas, Xenophotos, and Lo (Citation2007), 5- Önen (Citation2003), 6- Harris et al. (Citation1994), 7- Dimo-Lahitte, Monié, and Vergély (Citation2001), 8- Okay et al. (Citation1996), 9- Roddick, Cameron, and Smith (Citation1979), 10- Beccaletto and Jenny (Citation2004), 11- Spray and Roddick (Citation1980), 12- Çelik et al. (Citation2011), 13- Parlak et al. (Citation2013), 14- Hassig et al. (2013), 15- This study. Abbreviations, AO: Antalya Ophiolite; BHO: Beyşehir–Hoyran Ophiolite; DO: Divriği Ophiolite; EO: Eldivan ophiolite; GO: Göksun Ophiolite; IO: İspendere Ophiolite; KO: Kınık Ophiolite; KmO: Kömürhan Ophiolite; LO: Lesvos Ophiolite; MO: Mersin Ophiolite; ORO: Orhaneli Ophiolite; OsO: Osmaniye Ophiolite; PO: Pınarbaşı Ophiolite; PKO: Pozantı-Karsantı Ophiolite; SO: Sevan Ophiolite; KM: Konya mélange; mu: muscovite; hb: hornblende; bi: biotite. (Map modified after Dilek and Flower (Citation2003) and Çelik et al. (Citation2011)).
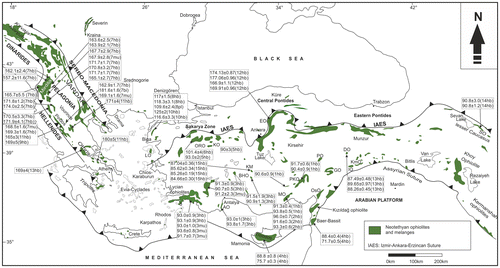
The HP/LT Anatolides are generally subdivided into the Tavşanlı Zone in the north and the Afyon Zone (also known as the Afyon-Bolkar Dağ Zone) further south (Okay & Tüysüz, Citation1999). The Tavşanlı Zone experienced higher grade blueschist facies metamorphism than the Afyon Zone (Candan et al., Citation2005; Davis & Whitney, Citation2006; Okay, Citation1986; Pourteau et al., Citation2010, Citation2013; Sherlock et al., Citation1999). The peak metamorphism in the Tavşanlı Zone is dated by Rb–Sr method on white micas (phengite) and yielded 78.5 ± 1.6 to 82.8 ± 1.7 Ma (Sherlock et al., Citation1999), indicating the passive margin subduction of the Tauride-Anatolide Platform during ophiolite emplacement in NW Turkey. As the initiation of subduction and formation of metamorphic soles have been linked to the ophiolite emplacement process, duration between intraoceanic thrusting and ophiolite emplacement onto the Tauride-Anatolide Platform, followed by subsequent HP–LT metamorphism could be constrained by using the metamorphic sole ages and ages of HP–LT metamorphism. This suggests that the initiation of oceanic lithosphere detachment and final emplacement onto the Tauride-Anatolide Platform spanned ~5 my. Parlak et al. (Citation2013) stated about 8–10 my for the same tectonic process in the eastern part of the Inner Tauride Ocean. This may indicate that duration between inception of intraoceanic subduction and final emplacement onto the continental margin largely depends on how far subduction zone is away from passive continental margin.
Conclusions
The main findings of the present study are as follows.
(1) | The metamorphic sole rocks within the Konya mélange crop out as thin slice beneath the sheared serpentinites and harzburgites. The rock types in the metamorphic sole are amphibolite, epidote-amphibolite, garnet-amphibole schist, plagioclase-amphibole schist, plagioclase-epidote-amphibole schist and quartz-amphibole schist. | ||||
(2) | Metamorphic sole preserves remnants of alkaline (seamount) and tholeiitic (E-MORB, IAT- and boninitic-type) magmatic rocks from the upper part of the oceanic crust. | ||||
(3) | The alkaline amphibolite (seamount-type) cooled below 510 ± 25 °C at 87 Ma, whereas the tholeiitic amphibolites at 85 Ma during intraoceanic thrusting/subduction. | ||||
(4) | The intraoceanic subduction initiated in the vicinity of an off-axis plume or a plume centered spreading ridge in the Inner Tauride Ocean at 87 Ma. During the later stage of the steady-state subduction, the E-MORB volcanics on the top of the down-going slab and the arc-type basalts (IAT/boninitic) detached from the leading edge of the overriding plate entered the subduction zone after ~2 my and metamorphosed to amphibolite facies within the Inner Tauride Ocean. | ||||
(5) | Duration of the intraoceanic detachment (~87 Ma) and ophiolite emplacement onto the Tauride-Anatolide Platform, followed by subsequent HP–LT metamorphism (~82 Ma) spanned ~5 my in the western part of the Inner Tauride Ocean. |
Acknowledgements
This work is a part of MSc study of first author who acknowledges financial support from the Çukurova University Research Foundation (Project No: MMF2005YL31). Erdin Bozkurt, Yann Rolland and Yener Eyüboğlu are thanked for their valuable comments that improved the quality of the paper.
References
- Agard, P., Jolivet, L., Vrielynck, B., Burov, E., & Monié, P. (2007). Plate acceleration: The obduction trigger? Earth and Planetary Science Letters, 258, 428–441.10.1016/j.epsl.2007.04.002
- Agard, P., Zuo, X., Funiciello, F., Bellahsen, N., Faccenna, C., & Savva, D. (2014). Obduction: Why, how and where. Clues from analog models. Earth and Planetary Science Letters, 393, 132–145.
- Al-Riyami, K., Robertson, A. H. F., Dixon, J., & Xenophontos, C. (2002). Origin and emplacement of the Late Cretaceous Baer-Bassit ophiolite and its metamorphic sole in NW Syria. Lithos, 65, 225–260.10.1016/S0024-4937(02)00167-6
- Andrew, T., & Robertson, A. H. F. (2002). The Beysehir-Hoyran-Hadim Nappes: Genesis and emplacement of Mesozoic marginal and oceanic units of the northern Neotethys in southern Turkey. Journal of the Geological Society, London, 159, 529–543.10.1144/0016-764901-157
- Bağcı, U., & Parlak, O. (2009). Petrology of the Tekirova (Antalya) ophiolite (Southern Turkey): Evidence for diverse magma generations and their tectonic implications during Neotethyan-subduction. International Journal of Earth Sciences, 98, 387–405.10.1007/s00531-007-0242-7
- Bağcı, U., Parlak, O., & Höck, V. (2008). Geochemistry and tectonic environment of diverse magma generations forming the crustal units of the Kızıldağ (Hatay) ophiolite southern Turkey. Turkish Journal of Earth Sciences, 17, 43–71.
- Bağcı, U., Parlak, O., & Höck, V. (2005). Whole-rock and mineral chemistry of cumulates from the Kızıldağ (Hatay) ophiolite (Turkey): Clues for multiple magma generation during crustal accretion in the southern Neotethyan Ocean. Mineralogical Magazine, 69, 53–76.10.1180/0026461056910234
- Bağcı, U., Parlak, O., & Höck, V. (2006). Geochemical character and tectonic environment of ultramafic to mafic cumulate rocks from the Tekirova (Antalya) ophiolite (southern Turkey). Geological Journal, 41, 193–219.10.1002/(ISSN)1099-1034
- Beccaluva, L., & Serri, G. (1988). Boninitic and low-Ti subduction-related lavas from intraoceanic arc-backarc systems and low-Ti ophiolites: A reappraisal of their petrogenesis and original tectonic setting. Tectonophysics, 146, 291–315.10.1016/0040-1951(88)90097-2
- Beccaletto, L., & Jenny, C. (2004). Geology and correlation of the Ezine Zone: A Rhodope fragment in NW Turkey? Turkish Journal of Earth Sciences, 13, 145–176.
- Beyarslan, M., & Bingöl, A. F. (2000). Petrology of a supra-subduction zone ophiolite (Elazığ, Turkey). Canadian Journal of Earth Sciences, 37, 1411–1424.10.1139/e00-041
- Candan, O., Çetinkaplan, M., Oberhänsli, R., Rimmelé, G., & Akal, C. (2005). Alpine high-P/low-T metamorphism of the Afyon Zone and implications for the metamorphic evolution of Western Anatolia, Turkey. Lithos, 84, 102–124.10.1016/j.lithos.2005.02.005
- Chan, G. H. N., Malpas, J., Xenophotos, C., & Lo, C. H. (2007). Timing of subduction zone metamorphism during the formation and emplacement of Troodos and Baër-Bassit ophiolites: Insights from 40Ar–39Ar geochronology. Geological Magazine, 144, 797–810.
- Çelik, Ö. F., & Delaloye, M. (2003). Origin of metamorphic soles and their post-kinematic mafic dyke swarms in the Antalya and Lycian ophiolites, SW Turkey. Geological Journal, 38, 235–256.
- Çelik, Ö. F., & Delaloye, M. (2006). Characteristics of ophiolite-related metamorphic rocks in the Beyşehir ophiolitic mélange (Central Taurides, Turkey), deduced from whole rock and mineral chemistry. Journal of Asian Earth Sciences, 26, 461–476.
- Çelik, Ö. F., Delaloye, M., & Feraud, G. (2006). Precise Ar-Ar ages from the metamorphic sole rocks of the Tauride Belt Ophiolites, southern Turkey: Implications for the rapid cooling history. Geological Magazine, 143, 213–227.
- Çelik, Ö. F., Marzoli, A., Marschik, R., Chiaradia, M., Neubauer, F., & Öz, İ. (2011). Early–Middle Jurassic intra-oceanic subduction in the İzmir-Ankara-Erzincan Ocean, Northern Turkey. Tectonophysics, 509, 120–134.
- Çelik, Ö. F. (2002). Geochemical, Petrological and Geochronological Observations on the Metamorphic Rocks of the Tauride Belt Ophiolites (S. Turkey) ( PhD thesis). Geneva University, Terre and Environment p. 39.
- Çelik, Ö. F. (2007). Metamorphic sole rocks and their mafic dykes in the eastern Tauride belt ophiolites (southern Turkey): Implications for OIB-type magma generation following slab break-off. Geological Magazine, 144, 849–866.
- Daşçı, H. T. (2007). Origin of Amphibolites within the Koyna Melange ( MSc thesis). Çukurova University. ( In Turkish with English abstract) Adana, Turkey.
- Davis, P. B., & Whitney, D. L. (2006). Petrogenesis of lawsonite and epidote eclogite and blueschist, Sivrihisar Massif, Turkey. Journal of Metamorphic Geology, 24, 823–849.
- Dewey, J. F. (1988). Lithospheric stress, deformation and tectonic cycles: The disruption of Pangea and the closure of Neo-Tethys. In M. G. Audley-Charles & A. Hallam (Eds.), Gondwana and Tethys (Vol. 37, pp. 23–40). Geological Society, London, Special Publications.
- Dilek, Y., & Furnes, H. (2009). Structure and geochemistry of Tethyan ophiolites and their petrogenesis in subduction rollback systems. Lithos, 113, 1–20.10.1016/j.lithos.2009.04.022
- Dilek, Y., & Thy, P. (2009). Island arc tholeiite to boninitic melt evolution of the Cretaceous Kızıldağ (Turkey) ophiolite: Model for multi-stage early arc-forearc magmatism in Tethyan subduction factories. Lithos, 113, 68–87.10.1016/j.lithos.2009.05.044
- Dilek, Y., & Flower, M. F. J. (2003). Arc-trench rollback and forearc accretion: 2. A model template for ophiolites in Albania, Cyprus, and Oman. In Y. Dilek & P. T. Robinson (Eds.), Ophiolites in Earth History (Vol. 218, pp. 43–68). Geological Society, London, Special Publication.
- Dilek, Y., & Whitney, D. L. (1997). Counterclockwise P-T-t trajectory from the metamorphic sole of a Neo-Tethyan ophiolite (Turkey). Tectonophysics, 280, 295–310.10.1016/S0040-1951(97)00038-3
- Dilek, Y., Thy, P., Hacker, B., & Grundvig, S. (1999). Structure and petrology of Tauride ophiolites and mafic dike intrusions (Turkey): Implications for the Neotethyan Ocean. Geological Society of America Bulletin, 111, 1192–1216.10.1130/0016-7606(1999)111<1192:SAPOTO>2.3.CO;2
- Dimo-Lahitte, A., Monié, P., & Vergély, P. (2001). Metamorphic soles from the Albanian ophiolites, petrology, 40Ar/39Ar geochronology, and geodynamic evolution. Tectonics, 20, 78–96.10.1029/2000TC900024
- Elitok, Ö., & Drüppel, K. (2008). Geochemistry and tectonic significance of metamorphic sole rocks beneath the Beyşehir-Hoyran ophiolite (SW-Turkey). Lithos, 100, 322–353.10.1016/j.lithos.2007.06.022
- Eren, Y., & Kurt, H. (2000). The stratigraphical, geochemical and geodynamical modelling of the northeast margin of Menderes-Taurus Block. Journal of the Faculty of Engineering and Architecture, Selcuk University, Konya, 15, 25–41.
- Eren, Y., Kurt, H., Rosselet, F., & Stampfli, G. M. (2004). Palaeozoic deformation and magmatism in the northern area of the Anatolide block (Konya), witness of the Palaeotethys active margin. Eclogae Geologicae Helvetiae, 97, 293–306.10.1007/s00015-003-1131-8
- Eren, Y. (1993). Konya Kuzeybatısında Bozdağlar masifinin otokton ve örtü birimlerinin stratigrafisi (Startigraphy of autochthonous cover units of the Bozdağlar massif, NW Konya). Geological Bulletin of Turkey, 36, 7–23.
- Floyd, P. A., & Winchester, J. A. (1978). Identification and discrimination of altered and metamorphosed volcanic rocks using immobile elements. Chemical Geology, 21, 291–306.10.1016/0009-2541(78)90050-5
- Floyd, P. A. (1993). Geochemical discrimination and petrogenesis of alkalic basalt sequences in part of the Ankara mélange, central Turkey. Journal of the Geological Society, London, 150, 541–550.10.1144/gsjgs.150.3.0541
- Göncüoğlu, M. C., Dirik, K., & Kozlu, H. (1996–1997). Pre-Alpine and Alpine terranes in Turkey: Explanatory notes to the terrane map of Turkey. Annalles Geologiques des Pays Hellenique, 37, 1–3.
- Göncüoğlu, M. C., Çapkınoğlu, S., Gürsu, S., Noble, P., Turhan, N., Tekin, U. K., … Göncüoğlu, Y. (2007). The Mississippian in the Central and Eastern Taurides (Turkey): Constraints on the tectonic setting of the Tauride-Anatolide Platform. Geologica Carpathica, 58, 427–442.
- Hacker, B. R., Mosenfelder, J. L., & Gnos, E. (1996). Rapid emplacement of the Oman ophiolite: Thermal and geochronologic constraints. Tectonics, 15, 1230–1247.10.1029/96TC01973
- Harrison, T. M. (1981). Diffusion of 40Ar in hornblende. Contributions to Mineralogy and Petrology, 78, 324–331.
- Harris, N. B.W., Kelley, S. P., & Okay, A. İ. (1994). Post-collision magmatism and tectonics in northwest Anatolia. Contributions to Mineralogy and Petrology, 117, 241–252.10.1007/BF00310866
- Hässig, M., Rolland, Y., Sosson, M., & Galoyan, G. (2011). New P-T-t data on the metamorphic sole of the Amasia ophiolites and implications for the geodynamic process, NW of the Sevan-Akera suture zone, Lesser Caucasus (Armenia). Geophysical Research Abstract, 13, EGU2011-7462-1.
- Jamieson, R. A. (1980). Formation of rnetamorphic aureoles beneath ophiolites – Evidence from the St. Anthony complex, Newfoundland. Geology, 8, 150–154.10.1130/0091-7613(1980)8<150:FORABO>2.0.CO;2
- Jamieson, R. A. (1986). P-T paths from high temperature shear zones beneath ophiolites. Journal of Metamorphic Geology, 4, 3–22.10.1111/jmg.1986.4.issue-1
- Lytwyn, J. N., & Casey, J. F. (1995). The geochemistry of postkinematic mafic dike swarms and subophiolitic metabasites, Pozanti-Karsanti ophiolite, Turkey: Evidence for ridge subduction. Geological Society of America Bulletin, 107, 830–850.10.1130/0016-7606(1995)107<0830:TGOPMD>2.3.CO;2
- Ludwig, K. R. (2003). User’s manual for Isoplot 3.0: A geochronological toolkit for Microsoft Excel. Berkely, CA: Berkeley Geochronology Center.
- Malpas, J., & Langdon, G. (1984). Petrology of upper pillow lava suite, Troodos ophiolite, Cyprus. In I. G. Gass, S. J. Lippard & A. W. Shelton (Eds.), Ophiolites and Oceanic Lithosphere (Vol. 13, pp. 155–167). Geological Society, London, Special Publications.
- Malpas, J. (1979). Two contrasting trondhjemite associations from transported ophiolites in Western Newfoundland, initial report. In F. Barker (Ed.), Trondhjemites, Dacites, and Related Rocks (pp. 465–487). Amsterdam: Elsevier.10.1016/B978-0-444-41765-7.50020-4
- McCaig, A. M. (1983). P-T conditions during emplacement of the Bay of Islands ophiolite complex. Earth and Planetary Science Letters, 63, 459–473.10.1016/0012-821X(83)90118-8
- Meyer, J., Mercolli, I., & Immenhauser, A. (1996). Off-ridge alkaline magmatism and seamount volcanoes in the Masirah island ophiolite, Oman. Tectonophysics, 267, 187–208.10.1016/S0040-1951(96)00094-7
- MTA. (2002). Geological Map of Turkey, 1:500,000. Ankara: Maden Tektik ve Arama Genel Müdürlüğü (General Directorate of Mineral Research and Exploration).
- Okay, Aİ., Tüysüz, O., Satır, M., Özkan-Altıner, S., Altıner, D., Sherlock, S., & Eren, R. H. (2006). Cretaceous and Triassic subduction-accretion, high-pressure-low-temperature metamorphism, and continental growth in the Central Pontides, Turkey. Geological Society of America Bulletin, 118, 1247–1269.10.1130/B25938.1
- Okay, A. İ., & Tüysüz, O. (1999). Tethyan sutures of northern Turkey. In B. Durand, L. Lolivet, F. Horvath, & M. Serrane (Eds.), The Mediterranean Basins: Tertiary extension within the Alpine orogeny (Vol. 156, pp. 475–515). Geological Society, London, Special Publications.
- Okay, A. I., Satır, M., Maluski, H., Siyako, M., Monie, P., Metzger, R., & Akyüz, S. (1996). Paleo- and Neo-Tethyan events in northwest Turkey: Geological and geochronological constraints. In A. Yin & M. Harrison (Eds.), Tectonics of Asia (pp. 420–441). Cambridge, UK: Cambridge University Press.
- Okay, A. İ. (1986). High-pressure/low-temperature metamorphic rocks of Turkey. Geological Society of America Memoir, 164, 333–347.10.1130/MEM164
- Önen, P., & Hall, R. (1993). Ophiolites and related metamorphic rocks from the Kütahya region, northwest Turkey. Geological Journal, 28, 399–412.
- Önen, P. (2003). Neotethyan ophiolitic rocks of the Anatolides of NW Turkey and comparison with Tauride ophiolites. Journal of Geological Society, London, 160, 947–962.
- Özcan, A., Göncüoğlu, M. C., Turhan, N., Uysal, S., & Şentürk, K. (1988). Late Palaeozoic evolution of the Kütahaya-Bolkardağ Belt. METU Journal of Pure and Applied Science, 21, 211–220.
- Özcan, A., Göncüoğlu, M. C., Turhan, N., Şentürk, K., Uysal, Ş., & Işık, A. (1990). Konya-Kadınhanı-Ilgın Dolayının Temel Jeolojisi [Geology of Konya-Kadınhanı-Ilgın Region] ( Report No. 9535). Ankara: General Directorate of Mineral Research and Exploration (MTA).
- Parlak, O. (1996). Geochemistry and geochronology of the Mersin Ophiolite within the Eastern Mediterranean tectonic frame ( PhD thesis). University of Geneva, Terre and Environnement, p. 6.
- Parlak, O. (2000). Geochemistry and significance of mafic dyke swarms in the Pozantı-Karsantı ophiolite (southern Turkey). Turkish Journal of Earth Sciences, 24, 29–38.
- Parlak, O., & Delaloye, M. (1999). Precise 40Ar/39Ar ages from the metamorphic sole of the Mersin ophiolite (southern Turkey). Tectonophysics, 301, 145–158.10.1016/S0040-1951(98)00222-4
- Parlak, O., & Robertson, A. H. F. (2004). The ophiolite-related Mersin Melange, southern Turkey: Its role in the tectonicsedimentary setting of Tethys in the Eastern Mediterranean region. Geological Magazine, 141, 257–286.10.1017/S0016756804009094
- Parlak, O., Höck, V., & Delaloye, M. (2000). Suprasubduction zone origin of the Pozantı-Karsantı ophiolite (southern Turkey) deduced from whole-rock and mineral chemistry of the gabbroic cumulates. In E. Bozkurt, J. A. Winchester, & J. D. Piper (Eds.), Tectonics and magmatism in Turkey and the surrounding area (Vol. 173, pp. 219–234). Geological Society, London, Special Publications.
- Parlak, O., Delaloye, M., & Bingöl, E. (1995). Origin of subophiolitic metamorphic rocks beneath the Mersin ophiolite, southern Turkey. Ofioliti, 20, 97–110.
- Parlak, O., Yılmaz, H., & Boztuğ, D. (2006). Geochemistry and tectonic setting of the metamorphic sole rocks and isolated dykes from the Divriği ophiolite (Sivas, Turkey): Evidence for melt generation within an asthenospheric window prior to ophiolite emplacement. Turkish Journal of Earth Sciences, 15, 25–45.
- Parlak, O., Delaloye, M., & Bíngöl, E. (1996). Mineral chemistry of ultramafic and mafic cumulates as an indicator of the arc-related origin of the Mersin ophiolite (southern Turkey). Geologische Rundschau, 85, 647–661.10.1007/BF02440102
- Parlak, O., Karaoğlan, F., Rızaoğlu, T., Klötzli, U., Koller, F., & Billor, Z. (2013). U-Pb and 40Ar–39Ar geochronology of the ophiolites and granitoids from the Tauride belt: Implications for the evolution of the Inner Tauride suture. Journal of Geodynamics, 65, 22–37.10.1016/j.jog.2012.06.012
- Pearce, J. A., & Cann, J. R. (1973). Tectonic setting of basic volcanic rocks determined using trace element analyses. Earth Planetary Science Letters, 19, 290–300.10.1016/0012-821X(73)90129-5
- Pearce, J. A., Harris, N. B. W., & Tindle, A. G. (1984). Trace element discrimination diagrams for the tectonic interpretation of granitic rocks. Journal of Petrology, 25, 956–983.10.1093/petrology/25.4.956
- Pierce, P. (1996). A users guide to basalt discrimination diagrams. In D. A. Wyman (Ed.), Trace element geochemistry of volcanic rocks: Applications for massive sulphide exploration (pp. 79–81). Geological Association of Canada: Geohemistry Short Course Notes.10.1061/(ASCE)1084-0680(1996)1:2(79)
- Peate, D. W., Pearce, J. A., Hawkesworth, C. J., Colley, H., Edwards, C. M. H., & Hirose, K. (1997). Geochemical variations in vanuatu arc lavas: The role of subducted material and a variable mantle wedge composition. Journal of Petrology, 38, 1331–1358.10.1093/petroj/38.10.1331
- Polat, A., Casey, J. F., & Kerrich, R. (1996). Geochemical characteristics of accreted material beneath the Pozantı-Karsantı ophiolite, Turkey: Intra-oceanic detachment, assembly and obduction. Tectonophysics, 263, 249–276.10.1016/S0040-1951(96)00026-1
- Pourteau, A., Candan, O., & Oberhänsli, R. (2010). High-pressure metasediments in central Turkey: Constraints on the Neotethyan closure history. Tectonics, 29. (TC5004, doi:10.1029/2009TC002650)
- Pourteau, A., Sudo, M., Candan, O., Lanari, P., Vidal, O., & Oberhänsli, R. (2013). Neotethys closure history of Anatolia: Insight from 40Ar–39Ar geochronology and P–T estimation in high-pressure metasediments. Journal of Metamorphic Geology, 31, 585–606.
- Renne, P. R., Swisher, C. C., Deino, A. L., Karner, D. B., Owens, T. L., & DePaolo, D. L. (1998). Intercalibration of standards, absolute ages and uncertainties in 40Ar/39Ar dating. Chemical Geology, 145, 117–152.
- Rızaoğlu, T., Parlak, O., Höck, V., & İşler, F. (2006). Nature and significance of Late Cretaceous ophiolitic rocks and its relation to the Baskil granitoid in Elazığ region, SE Turkey. In A. H. F. Robertson & D. Mountrakis (Eds.), Tectonic development of the Eastern Mediterranean region (Vol. 260, pp. 327–350). Geological Society, London, Special Publications.
- Rice, S., Robertson, A. H. F., & Ustaömer, T. (2006). Late Cretaceous–Early Cenozoic tectonic evolution of the Eurasian active margin in the central and Eastern Pontides, Northern Turkey. In A. H. F. Robertson & D. Mountrakis (Eds.), Tectonic development of the Eastern Mediterranean Region (Vol. 260, pp. 413–445). Geological Society, London, Special Publications.
- Rice, S., Robertson, A. H. F., Ustaömer, T., Inan, N., & Taslı, K. (2009). Late Cretaceous–Early Eocene tectonic development of the Tethyan suture zone in the Erzincan area, Eastern Pontides, Turkey. Geological Magazine, 146, 567–590.10.1017/S0016756809006360
- Robertson, A. H. F. (2004). Development of concepts concerning the genesis and emplacement of Tethyan ophiolites in the Eastern Mediterranean and Oman regions. Earth Science Reviews, 66, 331–387.
- Robertson, A. H. F., & Ustaömer, T. (2009). Formation of the Late Palaeozoic Konya Complex and comparable units in southern Turkey by subduction-accretion processes: Implications for the tectonic development of Tethys in the Eastern Mediterranean region. Tectonophysics, 473, 113–148.10.1016/j.tecto.2008.10.027
- Robertson, A. H. F., & Ustaömer, T. (2011). Role of tectonic-sedimentary mélange and Permian–Triassic cover units, central southern Turkey in Tethyan continental margin evolution. Journal of Asian Earth Sciences, 40, 98–120.10.1016/j.jseaes.2010.09.001
- Robertson, A. H. F., Parlak, O., & Ustaömer, T. (2009). mélange genesis and ophiolite emplacement related to subduction of the northern margin of the Tauride-Anatolide continent, central and western Turkey. In D. J. J. Van Hinsbergen, M. A. Edwards, & R. Govers (Eds.), Collision and collapse at the Africa-Arabia-Eurasia subduction zone (Vol. 311, pp. 9–66). Geological Society, London, Special Publications.
- Robertson, A. H. F., Parlak, O., & Ustaömer, T. (2013). Late Palaeozoic–Early Cenozoic tectonic development of Southern Turkey and the easternmost Mediterranean region: Evidence from the inter-relations of continental and oceanic units. In A. H. F. Robertson, O. Parlak, & U. C. Ünlügenç (Eds.), Geological development of the Anatolian Continent (Vol. 372, pp. 9–48). Geological Society, London, Special Publications.
- Robertson, A. H. F. (2002). Overview of the genesis and emplacement of Mesozoic ophiolites in the Eastern Mediterranean Tethyan region. Lithos, 65, 1–67.10.1016/S0024-4937(02)00160-3
- Roddick, J. F., Cameron, W. E., & Smith, A. G. (1979). Permo–Triassic and Jurassic 40Ar–39Ar ages from Greek ophiolites and associated rocks. Nature, 279, 788–790.10.1038/279788a0
- Rojay, B., Yalınız, M. K., & Atıner, D. (2001). Tectonic implications of some cretaceous pillow basalts from the northern Anatolian ophiolitic mélange (Central Anatolia-Turkey) to the evolution of Neotethys. Turkish Journal of Earth Sciences, 10, 93–102.
- Rojay, B. (2013). Tectonic evolution of the Cretaceous Ankara Ophiolitic Mélange during the Late Cretaceous to pre-Miocene interval in Central Anatolia, Turkey. Journal of Geodynamics, 65, 66–81.10.1016/j.jog.2012.06.006
- Rolland, Y., Galoyan, G., Bosch, D., Sosson, M., Corsini, M., Fornari, M., & Verati, C. (2009). Jurassic back-arc and Cretaceous hot-spot series In the Armenian ophiolites — Implications for the obduction process. Lithos, 112, 163–187.10.1016/j.lithos.2009.02.006
- Saccani, E., & Photiades, A. (2004). Mid-ocean ridge and supra-subduction affinities in the Pindos ophiolites (Greece): Implications for magma genesis in a forearc setting. Lithos, 73, 229–253.10.1016/j.lithos.2003.12.002
- Saccani, E., & Photiades, A. (2005). Petrogenesis and tectonomagmatic significance of volcanic and subvolcanic rocks in the Albanide-Hellenide ophiolitic mélanges. The Island Arc, 14, 494–516.10.1111/iar.2005.14.issue-4
- Sherlock, S., Kelley, S. P., Inger, S., Harris, N., & Okay, A. İ. (1999). 40Ar-39Ar and Rb-Sr geochronology of high-pressure metamorphism and exhumation history of the Tavsanli Zone, NW Turkey. Contributions to Mineralogy and Petrology, 137, 46–58.10.1007/PL00013777
- Smith, R. E., & Smith, S. E. (1976). Comments on the use of Ti, Zr, Y, Sr, K, P and Nb in classification of basaltic magmas. Earth and Planetary Science Letters, 32, 114–120.10.1016/0012-821X(76)90049-2
- Smith, A. G., Hurley, A. M., & Briden, J. C. (1981). Phanerozoic Palaeocontinental maps. Cambridge: Cambridge University Press.
- Spray, J. G. (1984). Possible causes and consequences of upper mantle decoupling and ophiolite displacement. In I. G. Gass, S. J. Lippard, & A. W. Shelton (Eds.), Ophiolites and oceanic lithosphere (Vol. 13, pp. 255–268). Geological Society, London, Special Publications.
- Spray, J. G., & Roddick, J. C. (1980). Petrology and 40Ar/39Ar geochronology of some Hellenic sub-ophiolite metamorphic rocks. Contributions to Mineralogy and Petrology, 72, 43–55.10.1007/BF00375567
- Sun, S. S., & McDonough, W. F. (1989). Chemical and isotopic systematics of oceanic basalts: Implications for mantle composition and processes. In A. D. Saunders, & M. J. Norry (Eds.), Magmatism in the Ocean Basins (Vol. 42, pp. 313–347). Geological Society, London, Special Publications.
- Thompson, G. (1991). Metamorphic and hydrothermal processes: Basalt-seawater interactions. In P. A. Floyd (Ed.), Oceanic basalts (pp. 148–173). Glasgow: Blackie.
- Vaughan, A. P. M., & Scarrow, J. H. (2003). Ophiolite obduction pulses as a proxy indicator of superplume events? Earth and Planetary Science Letters, 213, 407–416.10.1016/S0012-821X(03)00330-3
- Vergili, Ö., & Parlak, O. (2005). Geochemistry and tectonic setting of metamorphic sole rocks and mafic dikes from the Pınarbaşı (Kayseri) ophiolite, Central Anatolia. Ofioliti, 30, 37–52.
- Wakabayashi, J., Ghatak, A., & Basu, A. R. (2010). Suprasubduction-zone ophiolite generation, emplacement, and initiation of subduction: A perspective from geochemistry, metamorphism, geochronology, and regional geology. Geological Society of America Bulletin, 122, 1548–1568.10.1130/B30017.1
- Williams, H., & Smyth, W. R. (1973). Metamorphic aureoles beneath ophiolite suites and alpine peridotites; tectonic implications with west Newfoundland examples. American Journal of Science, 273, 594–621.10.2475/ajs.273.7.594
- Winchester, J. A., Park, R. G., & Holland, J. G. (1980). The geochemistry of Lewisian semipelitic schists from the Gairloch District, Wester Ross. Scottish Journal of Geology, 16, 165–179.10.1144/sjg16020165
- Yalınız, K. M., Floyd, P., & Göncüoğlü, M. C. (1996). Supra-subduction zone Ophiolites of Central Anatolia: Geochemical evidence from the Sarikaraman Ophiolite, Aksaray, Turkey. Mineralogical Magazine, 60, 697–710.10.1180/minmag
- Yalınız, K. M., Floyd, P., & Göncüoğlu, M. C. (2000). Geochemistry of volcanic rocks from the Çiçekdağ Ophiolite, Central Anatolia, Turkey, and the their inferred tectonic setting within the northern branch of the Neotethyan Ocean. In E. Bozkurt, J. A. Winchester, & J. D. A. Piper (Eds.), Tectonics and mangamism in Turkey and the surrounding area (Vol. 173, pp. 203–218). London: Geological Society. Special Publications.
- Yılmaz, Y. (1993). New evidence and model on the evolution of the southeast Anatolian orogen. Geological Society of America Bulletin, 105, 251–271.10.1130/0016-7606(1993)105<0251:NEAMOT>2.3.CO;2
- Yılmaz, Y., Yiğitbaş, E., & Genç, Ş. C. (1993). Ophiolitic and metamorphic assemblages of southeast Anatolia and their significance in the geological evolution of the orogenic belt. Tectonics, 12, 1280–1297.10.1029/93TC00597