ABSTRACT
Spatially continuous rock assemblages that share similar environmental evolution or structural features can be classified as a single tectonic unit. This approach enables to link dispersed units or massifs with each other and sometimes can be subjective, depending on the classification criteria. The relationship and the nature of the contact between the Strandja Massif and the İstanbul Zone have been controversial due to the Cainozoic cover. Amalgamation of these units was claimed as early as the Aptian-Albian.
Lower Triassic sedimentary rocks, which are overlain by the Carboniferous flysch with a N-verging thrust fault are exposed NW of the İstanbul Zone. This study reveals the spatial relationship between the Strandja Massif and the İstanbul Zone deduced from the U-Pb dating and Lu-Hf isotopes of the detrital zircons from these Lower Triassic clastics. Our results show that the early Triassic basin was fed from a provenance that included arc-related Upper Carboniferous-Lower Permian magmatic rocks which is much more likely to be the Strandja Massif than the İstanbul Zone. The second outcome of this study is that a unit that previously assigned to Palaeozoic turned out to be Triassic, which brings the Strandja Massif farther to the east, into the northern İstanbul Zone.
1. Introduction
The Rhodope-Pontide Fragment (Şengör & Yilmaz, Citation1981) is the northernmost part of the Alpides in Anatolia. It stretches from the eastern Carpathians in the west (Burchfiel, Citation1980) to the Caucasus in the east (Şengör & Yilmaz, Citation1981), embracing the Moesian Platform form the west and extending along the southern Black Sea coast. It is separated from the southern tectonic units in Turkey by the northern branch of the Neo-Tethyan suture (the İzmir-Ankara-Erzincan suture, Ketin, Citation1966; Şengör & Yilmaz, Citation1981). According to Şengör and Yilmaz (Citation1981) it essentially consists of four tectonic units that amalgamated before the late Cretaceous, from west to east; the Rhodope Massif, the Strandja Massif, the İstanbul Zone and the Eastern Pontides. The main difference in its definition is that Ketin includes the Sakarya Continent into his Pontides whereas Şengör and Yilmaz (Citation1981) separates the Sakarya Continent from the İstanbul Zone by the Intra-Pontide suture. Okay, Şengör, and Görür (Citation1994) considered the Eastern Pontides as the eastern continuation of the Sakarya Zone, an interpretation not here followed. Within the Rhodope-Pontide Fragment the Strandja Massif and the İstanbul Zone form the northern part of Ketin’s (Citation1966) Western Pontides.
The Strandja Massif has a crystalline basement and a cover metamorphosed under epidote-amphibolite to greenschist facies (Aydın, Citation1982; Gočev, Citation1979, Citation1991; Ketin, Citation1982; Natal’in, Sunal, Satir, & Toraman, Citation2012; Okay, Satır, Tüysüz, Akyüz, & Chen, Citation2001; Pamir & Baykal, Citation1947; Sunal, Natal’in, Satir, & Toraman, Citation2006; Sunal, Satir, Natal’in, Topuz, & Vonderschmidt, Citation2011). The basement consists of pre-Cambrian K-Feldspar orthogneisses (Natal’in, Sunal, Gün, Wang, & Zhiqing, Citation2016; Şahin, Aysal, Güngör, Peytcheva, & Neubauer, Citation2014) nonconformably overlain by Ordovician (?) quartzites (Natal’in et al., Citation2016). The age of bt-schists in Kırklareli region was constrained between Devonian-late Carboniferous (Sunal, Satir, Natal’in, & Toraman, Citation2008). Furthermore in Kıyıköy region a large variety of rock associations was interpreted as Carboniferous accretionary prism (Natal’in et al., Citation2016; Şengör & Özgül, Citation2010). All of the basement rocks described here were intruded by Late Carboniferous and Permian granitoids (orthogneisses and metagranitoids) (Aydın, Citation1974; Aydın, Citation1982; Natal’in et al., Citation2016, Citation2012; Okay et al., Citation2001; Sunal et al., Citation2006). These units are unconformably overlain by a Triassic to Jurassic metasedimentary cover (Aydın Citation1974; Aydın, Citation1982; Çağlayan, Şengün, & Yurtsever, Citation1988; Natal’in et al., Citation2016, Citation2012; Okay et al., Citation2001; Sunal et al., Citation2006). Both the basement with intrusions and its lower Mesozoic sedimentary cover were penetratively deformed and accompanied by late Jurassic to early Cretaceous regional metamorphism (Natal’in, Satır, Sunal, & Toraman, Citation2005b; Natal’in, Sunal, & Toraman, Citation2005a). The rocks of the Strandja Massif underwent phases of multiple deformation; a regional late Palaeozoic high grade metamorphism (Natal’in et al., Citation2012; Okay et al., Citation2001) and an overprinting Jurassic-Cretaceous epidote-amphibolite to greenschist facies metamorphism, both being related to the closure of the Paleo-Tethys (Natal’in et al., Citation2012; Şengör, Yılmaz, & Sungurlu, Citation1984). The late Carboniferous orthogneisses of the Strandja Massif had already been metamorphosed together with the basement sediments before the emplacement of the early Permian Kırklareli granites and gained an early foliation that was later overprinted by the regional Mesozoic foliation (Natal’in et al., Citation2012).
Natal’in et al. (Citation2016) divided the Strandja Massif into two regions; the western Strandja Massif, called the Kırklareli region and the eastern Strandja Massif, termed the Kıyıköy region. The oldest rocks in the Kırklareli region are the Palaeozoic sediments (Devonian (?)- late Carboniferous, (Sunal et al., Citation2008), Natal’in et al., Citation2012) intruded by extensive late Carboniferous arc related plutons (Natal’in et al., Citation2016, Citation2012, Citation2005a; Sunal et al., Citation2006). The Permo-Carboniferous interval is represented by schists with amphibolites intruded by the Permian Kırklareli pluton. Detrital zircon ages have shown that the sedimentation started during the Ordovician and lasted at least till the Carboniferous (?) (Natal’in et al., Citation2012; Sunal et al., Citation2006) and then intruded by the late Carboniferous arc related magmatics (Natal’in et al., Citation2016). Natal’in et al. (Citation2016) claimed that the Paleozoic sediments exposed in the Kıyıköy region is similar to the İstanbul Zone in terms of their lithologies.
Şengör and Özgül (Citation2010) and, following them, Natal’in et al. (Citation2016) also stated that there are extensive schist and phyllitte alternation including mafic rocks (former pyroxenites) as blocks and meta-chert inter-layers, which can be interpreted rocks of an accretionary prism. Detrital zircon ages from metasedimentary rocks revealed Ordovician (433 and 446 Ma), Devonian and Carboniferous (350 Ma) magmatic activities in the source areas (Natal’in et al., Citation2012) indicating the Strandja Massif was a part of a long-lived arc from Ordovician to Permian. They linked this arc with the late Palaeozoic–early Mesozoic Silk Road arc. Late Palaeozoic (late Carboniferous) subduction-accretion in the Strandja Massif is synchronous with orogeny in the Balkans.
Although, there are some differences in the geological history of western and eastern Strandja Massifs, both of them are metamorphosed during the late Jurassic -early Cretaceous interval and created penetrative foliation via N-verging thrust tectonics (Aydın, Citation1982; Gočev, Citation1976, Citation1979; Natal’in et al., Citation2016, Citation2012; Okay et al., Citation2001; Şengör, Citation1984; Şengör et al., Citation1984; Sunal et al., Citation2011).
The basement of the İstanbul Zone consists of Neoproterozoic (here Pan-African) metamorphic rocks (Chen et al., Citation2002). This basement is overlain by a continuous, well-developed Atlantic-type sedimentary sequence extending from the Lower Ordovician to the Upper Devonian. The basin, which was tectonically stable from the Ordovician to the end of the Devonian, became tectonically active and turned into a site of turbiditic flysch deposition during the Early Carboniferous. The Carboniferous flysch has long been interpreted to mark the progress of a collision (Görür et al., Citation1997; Şengör & Özgül, Citation2010). The Palaeozoic sequence is unconformably overlain by late Permian and younger sedimentary strata (Özgül, Citation2012). The geology of the İstanbul Zone resembles that of the Hercynides with its abundant Lower Carboniferous flysch deposits passing into Upper Carboniferous molasses carrying with a sharp unconformity upon its sediments.
The reported isotopic age data from the eastern part of İstanbul Zone, revealed Ediacaran magmatic activity related to subduction (Ustaömer, Mundil, & Renne, Citation2005). Detrital zircon ages from Lower Ordovician quartzite of Aydos Formation showed a large age span with dominance of Neoproterozoic zircon ages (around 540, 570, 600–640 and 700–800 Ma: all Pan-African) (Ustaömer, Ustaömer, Gerdes, & Zulauf, Citation2011). Okay, Zack, Okay, and Barth (Citation2011) published zircon ages from the Lower Carboniferous Trakya Formation showing predominantly bimodal age distribution from Late Devonian to Early Carboniferous (390 to 335 Ma) and Cambrian–Neoproterozoic (640 to 520 Ma). The rutile ages from the same study yielded late Devonian–early Carboniferous ages, which Okay et al. (Citation2011) reported them as derivativesfrom amphibolite-facies metamorphic rocks. The Permian Sancaktepe Granite (~254 Ma) has been interpreted as a product of post-collisional tectonics (Bürküt, Citation1976; Okay et al., Citation2001; Yilmaz, Citation1977; Yılmaz Şahin, Aysal, & Güngör, Citation2010), but it is more likely a product of the ongoing Cimmeride subduction; it can be correlated with the Kırklareli Metagranite. Similar but slightly older Permian granitoid (Kürek) is reported from the eastern part of the İstanbul Zone (Okay et al., Citation2013).
The relationship between the İstanbul Zone and the Strandja Massif and their contact have long been a matter of discussion. Early publications suspected an overthrust contact between these units (Şengör, Citation1984; Şengör et al., Citation1984) and interpreted amalgamation through a suture zone (Yilmaz, Citation1977) whereas Okay et al. (Citation1994) considered a right lateral strike-slip fault between them and brought the İstanbul Zone during the opening of the Black Sea from the north, where the Moesian platform is exposed. The contact between them concealed under the sediments of the Trace basin somewhere in the west of İstanbul, in the Çatalca region. The nature of the contact thus remains uncertain.
Northwest of Bosphorus, in a very limited area, a Triassic sequence crops out (; Kaya & Lys, Citation1979-1980). The base of this sequence consists of Lower Triassic massive basaltic lava flows that are overlain by Upper Triassic (Scythian) white to light grey, thickly-bedded Çiftalan Sandstone, which was investigated in this study. The overlying late Scythian-Anisian Köseler Limestone passes into Bakırlıkıran Formation, represented by sandstone and shale.
Figure 1. Map of the Strandja Massif and the İstanbul Zone and relations with surrounding units (Compiled from Türkecan & Yurtsever, Citation2002; Özgül, Citation2011).
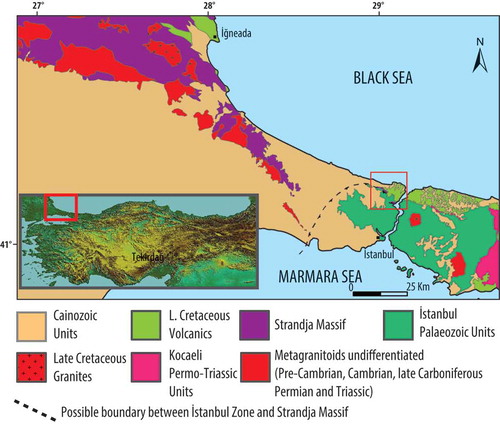
Triassic units in northwestern İstanbul and Kocaeli regions correlate with each other () (Kaya & Lys, Citation1979-1980; Lom, Ülgen, Sakınç, & Şengör, Citation2016).
Figure 2. Generalized strtatigraphic section of Triassic units of the İstanbul Zone. (Lom et al., Citation2016).
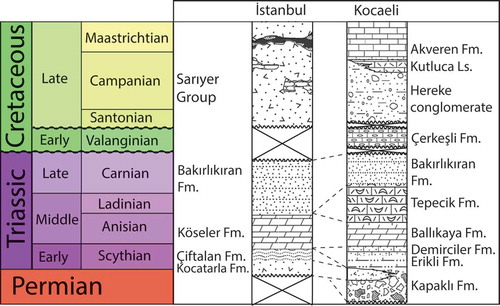
The Triassic units exposed in the northwestern part of İstanbul are thrust by Carboniferous flysch of the İstanbul Zone (Gedik, Timur, Duru, & Pehlivan, Citation2005; Kaya & Lys, Citation1979-1980; Özgül, Citation2011). Age of thrusting remains unclear: it could be medial or late Mesozoic related to the final closure of the Palaeo-Tethys here.
In this study we examined the ages of the detrital zircons obtained from the Triassic rocks in northwest İstanbul and correlated them with the known age data reported from the İstanbul Zone and the Strandja Massif. According to our provenance analyses of the Triassic rocks we came to a conclusion that they were fed from the Strandja Massif rather than the İstanbul Zone. Thus, the Triassic rocks can be attributed to the Strandja Massif and the buried contact between these two units in the NW part of İstanbul must be thrust fault as it is in the exposed area (). The second result of the present study is the necessity of reexamination of some clastic rocks of the İstanbul Zone attributed to Palaeozoic from which there have so far been no palaeontological data. We here show that one such allegedly Silurian unit turned out to be at the oldest post-Carboniferous!
Figure 3. The geological map of the northwestern Bosphorus (Modified after Özgül, Citation2011).
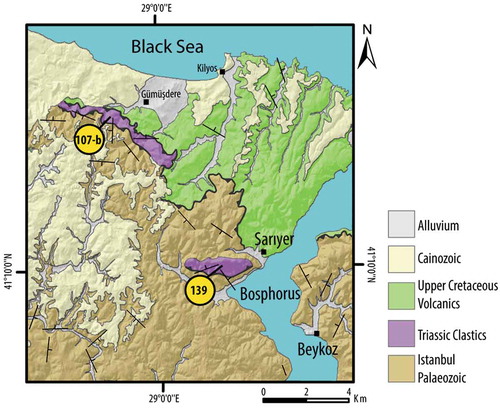
2. Analytical techniques
Standard mineral separation techniques, which include grinding, sieving, Frantz isodynamic separator and heavy liquids were used for zircon extraction from rock samples. Extracted zircons were handpicked under binocular microscope and mounted. Due to low number of the extracted zircon grains all of the 161 zircons between 63–180 µm are used for dating. Cathodoluminescence (CL) images of the polished zircons were taken in Hacettepe University by a Zeiss Evo 50 EP scanning electron microscope.
Uranium, thorium and lead isotope analyzes were carried out by laser ablation – inductively coupled plasma – mass spectrometry (LA-ICP-MS) at the Goethe University of Frankfurt (GUF), using a slightly modified method, as previously described in Gerdes and Zeh (Citation2006, Citation2009)) and Zeh and Gerdes (Citation2012). A ThermoScientific Element 2 sector field ICP-MS was coupled to a Resolution S-155 (Resonetics) 193 nm ArF Excimer laser (CompexPro 102, Coherent) equipped with two-volume ablation cell (Laurin Technic, Australia). Laser spots of 20–30 µm were fired with 5.5 Hz at a fluence of about 1.5 J cm−2. Raw data were corrected offline for background signal, common Pb, laser induced elemental fractionation, instrumental mass discrimination, and time-dependent elemental fractionation of Pb/U using an in-house MS Excel© spreadsheet program (Gerdes & Zeh, Citation2006, Citation2009). Laser-induced elemental fractionation and instrumental mass discrimination were corrected by normalization to the reference zircon GJ-1 (0.0982 ± 0.0003; ID-TIMS GUF value). Repeated analyses of the reference zircon Plesovice, BB-16 and 91,500 (Santos et al., Citation2017; Sláma et al., Citation2008; Wiedenbeck et al., Citation1995) during the same analytical session yielded an accuracy of better 1% and a reproducibility of <2% (2 SD). All uncertainties are reported at the 2sigma level. The analytical results are presented in Table 1 (supplementary material). Concordia diagrams are prepared by using ISOPLOT spreadsheet (Ludwig, Citation2003) and AgeDisplay (Sircombe, Citation2004) were used to prepare the probability density distribution (PDD) and the histograms.
Hafnium isotope measurements carried by using Thermo-Finningan Neptune multi collector ICP-MS coupled to the same laser system as for U-Pb analysis following the method described in Gerdes and Zeh (Citation2006, Citation2009)). Spots of 40 µm in diameter were drilled with a repetition rate of 5.5 Hz and an energy density of 5 J/cm2 during 50s of data acquisition. All data were adjusted relative to the JMC475 of 176Hf/177Hf ratio = 0.282160 and quoted uncertainties are quadratic additions of the within run precision of each analysis and the reproducibility of the JMC475 (2SD = 0.0028%, n = 8). Accuracy of the Hf isotope measurements were verified by repeated analyses of reference zircon GJ-1 and Temora, which yielded a 176Hf/177Hf of 0.282008 ± 0.000019 (2 SD, n = 62) and 0.282685 ± 0.000022 (n = 14), respectively. This is in perfect agreement with the LA-MC-ICPMS long-term average of GJ-1 (0.282010 ± 0.000025; n > 800) and Temora (0.282483 ± 0.000023, n > 250) reference zircon at GUF.
The initial 176Hf/177Hf values are expressed as εHf(t), which is calculated using a decay constant value of 1.867 × 10 − 11 year−1, CHUR after Bouvier, Vervoort, and Patchett (Citation2008; 176Hf/177HfCHUR,today = 0.282785 and 176Lu/177Hf CHUR,today = 0.0336) and the apparent U-Pb ages obtained for the respective domains (see supplementary material Table 2). For the calculation of Hf two stage model ages (TDM) in billion years the measured 176Lu/177Hf of each spot (first stage = age of zircon), a value of 0.0113 for the average continental crust, and a depleted mantle 176Lu/177LuDM = 0.0384 and 176Hf/177Hf DM = 0.28315 (average MORB; Chauvel, Lewin, Carpentier, Arndt, & Marini, Citation2008) were used. International Stratigraphic Chart of the International Commision on Stratigarphy is used as the timescale (Cohen, Finney, Gibbard, & Fan, Citation2013).
3. Results
3.1. Description of samples
Two samples were collected from the western side of the Bosphorusşo; the sample 107-b was taken from near the Black Sea coast, close to Gümüşdere village and the second one, the sample 139, was taken from NW coast of the Bosphorus, near Sarıyer village (). The sample 107-b was collected from below the thrust fault boundary of the Lower Triassic Çiftalan sandstone (Kaya & Lys, Citation1979-1980) with Carboniferous flysch of the Trakya Formation (). The sample is white to light grey sandstone (subarkose) with predominantly quartz, lesser plagioclase, alkali feldspar and minor lithic grains. Sum of 132 zircon grains were extracted from this sample.
The sample 139 is yellow to light brown, greenish-grey, fine-grained sandstone and siltstone alternation of so-called Upper Ordovician-Lower Silurian Gözdağ Member of the Yayalar Formation (Özgül, Citation2012). Only 30 zircons could be extracted from this sample.
3.2. Zircon external and internal structures
shows cathodoluminescence (CL) images of the selected zircons representing the peak ages in the probability distribution diagrams illustrated in . Zircon grains from the sample 107-b are colourless, transparent and often idiomorphic. Their sizes range from 70 and 150 µm. Grains have aspect ratios between 1:1.5 and 1:4. The dominant structures in the grains are well developed oscillatory magmatic zoning, which gets sometime blurred. Other grains show resorption and CL bright overgrowth rims (e.g. grains A425, A419 and A437) implying an overprinting metamorphic event.
Figure 4. CL images of the selected zircon grains from the sample 107-b (A) and the sample 139 (B). Red dotted circles indicate the locations of laser spots. Associated ages are shown below the images indicate peak ages illustrated in ). Size of the spots are ~30 µm.
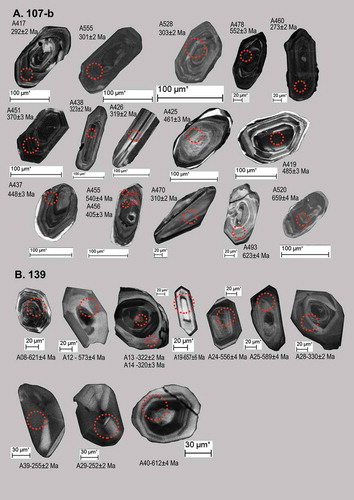
Figure 5. Probability density distribution diagrams and histograms of the detrital zircon ages obtained from the samples 107-b and 139.
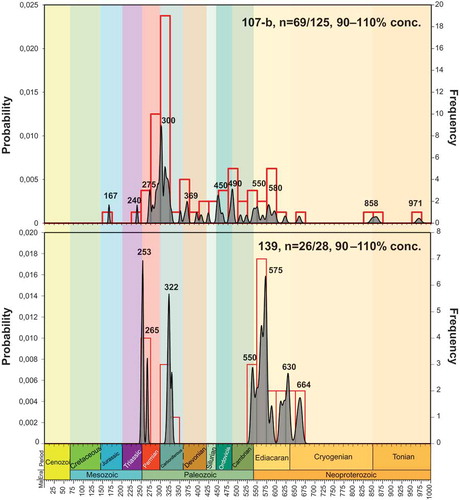
Zircon grains obtained from the sample 139 are also colourless, transparent and mostly idiomorphic but their sizes are smaller (between 70 µm and 100 µm). Many grains are slightly rounded and their aspect ratios range from 1:1.2 and 1:2 While the majority of the crystals show normal oscillatory zoning some grains display in addition sector zoning (e.g. A12, A39, A29), either. Compared to sample 107-b zircon grains, the oscillatory zoning is wider (e.g. A13, 28 and 40).
Both samples are poor in terms of inheritance. Core structure is rare and roundness is not well expressed, which requires short transport and less reworking. Dating spots have been defined in places where zircons have oscillatory magmatic zoning (). During the identification of the laser spots, we try to avoid placing them into mixed domains. Therefore, we believe that all ages obtained represent real geological events. Especially magmatic character of the late Carboniferous ages spots is obvious in the CL images of the zircons from sample 107-b ().
3.3. Detrital zircon U-Pb ages
3.3.1. The sample 107-b
132 zircons were dated from the sample 107-b and 76 of them are 90% – 110% concordant (see electronical supplementary materials Table 1). 167 ± 3 Ma is obtained as youngest concordant age and 2142 ± 16 Ma is the oldest one. For a better visuality, 7 ages older than 1000 Ma of the concordant ones are excluded from the PDD diagrams and histograms in . Excluded ages are; 1246 ± 17, 1469 ± 10, 1740 ± 18, 1950 ± 17, 2123 ± 9 and 2142 ± 16 Ma. Majority of the ages are grouped in Upper Carboniferous with 19 grains, which constitute 28% of total ages obtained from this sample (). Rest of the ages are; Permian, 12 ages (17%), Ediacaran, 10 ages (15%), Ordovician, 7 ages (10%), Cambrian, 6 ages (9%), Devonian, 5 ages (7%), Lower Carboniferous, 3 ages (4%), Cryogenian, 2 ages (3%), Tonian, 2 ages (3%), Triassic and Jurassic, 2 ages (3%), and a Silurian age. The prominent age peak in the PDD diagram () is 300 Ma.
3.3.2. The sample 139
30 zircons were dated from this sample and 28 of them fall into the 90%-110% concordance interval (see electronical supplementary materials Table 1). The youngest and the oldest concordant ages are 252 ± 2 Ma and 1912 ± 6 Ma, respectively. Another age older than 1.0 Ga is 1357 ± 12 Ma. The most abundant age group that belongs to Ediacarian is represented by 14 grains (56%). Other age groups are; Permian, 3 ages (12%), Upper Carboniferous, 2 ages (8%), Lower Carboniferous, 2 ages (8%), Cambrian, 2 ages (8%), Jurassic and Triassic, one age (4%) and one Cryogenian age (4%) (). The PDD diagram and the histogram of the sample 139 is shown in . The main age peaks in this sample are 253 Ma and 575 Ma.
3.4. Hf isotopes
3.4.1. The sample 107-b
Seventy-five of the 76 dated zircons from the sample 107-b analyzed for Lu-Hf isotopes, data is represented in supplementary materials Table 2. illustrates εHf (t) vs. age graph. For a better visuality only ages younger than 1 Ga are plotted. In general, εHf(t) values are between −25.7 and +9.3 (69% negative) and the model ages are between 0.92 Ga (Tonian) and 3.67 Ga (Eoarchean). Thirty-four analyses are enclosed between 270–329 Ma (Middle Permian- Lower Carboniferous). The εHf(t) values of such zircons are grouped around the CHUR (CHondritic Uniform Reservoir) and vary between −6.1 and +2.8 (67% of them have negative values). Hf model ages range from 1.0 Ga (Tonian) to 1.5 Ga (Calymmian). Five of the zircons lie between 352–405 Ma (Lower Carboniferous-Lower Devonian) and their εHf(t) values are highly scattered ranging from +0.5 to −16. Four of them are negative. Oldest model age in this group is 2.1 Ga with −16 εHf(t) value and youngest model age is 1.2 Ga with a 0.5 εHf(t) value. The second largest group of zircon ages (n = 11) lie between 424 Ma (Upper Silurian) and 488 Ma (Furongian). εHf(t) values vary from −9.4 to +1.0 (73% negative) and the model ages fall between 1.2 Ga (Stenian) and 1.8 Ga (Orosirian). The third group is defined by 12 grains yielding ages between 523 Ma and 623 Ma and εHf(t) values between −15.4 and +2.1, corresponding to model ages of 1.2 Ga (Ectasian) to 2.2 Ga (Rhyacian). The remaining data in the graph shows scattered model ages and εHf(t) values. There are 4 zircons with ages between 659 Ma and 969 Ma and εHf(t) values from +9.3 to −25.7. Model ages of these zircons are between 1.9 Ga (Orosirian) – 3.0 Ga (Mesoarchean). Five zircons older than 1 Ga (between 1.25 and 2.14 Ga) are excluded from graph intentionally because of visuality of the graph. The εHf(t) values range from +6.8 to −22.8 corresponding to model ages of 2.0 Ga (Orosirian) and 3.7 Ga (Eoarchean).
Figure 7. εHf(t) – Age graph of the sample 107-b (blue squares) and the sample 139 (orange circles). CHUR is chondritic reservoir from Blichert-Toft and Albarède (Citation1997), which calculated from chondritic Hf composition (176Hf/177Hf = 0.282772). Depleted mantle lines are based on 176Hf/177Hf of 0.28325 (upper line; Chauvel & Blichert-Toft, Citation2001) and 176Hf/177Hf of 0.283164 (lower line; Chauvel et al., Citation2008) Dashed line represents average continental crust evolutionary trend for 176Lu/176Hf of 0.0113 (Rudnick & Gao, Citation2003).
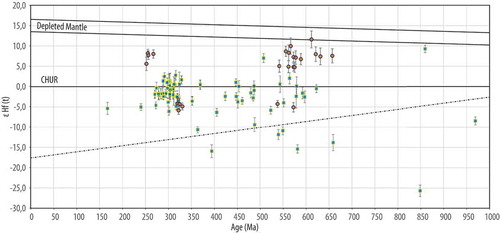
3.4.2. The sample 139
Twenty-five of the dated 26 concordant zircons examined for Lu-Hf isotopes, data is represented in supplementary materials (). In general, εHf(t) values are between −10 and +11.6 and negative values constitute 28% of sum. The model ages are ranging from 0.65 Ga (Cryogenian) to 3.0 Ga (Mesoarchean). One analyses with an age older than 1.0 Ga is excluded from the graph. The rest of the data show three main age groups. The youngest group is represented by 4 zircons distributed between 252 Ma (Upper Permian) and 267 Ma (Middle Permian). They have εHf(t) values between +5.6 and +8.2 and model ages from 0,65 Ga to 0.79 Ga (Cryogenian). The second group is defined by 4 zircons with ages between 320 Ma and 330 Ma (Lower Carboniferous) and εHf(t) values of −4.2 to −5.9 corresponding to model ages of 1.4 Ga (Ectasian) to 1.5 Ga (Calymmian). The third group consists of 16 grains with ages between 537 Ma (Lower Cambrian) and 657 Ma (Cryogenian). Their εHf(t) values vary between −5.1 and +11.6, 13% of them are negative. Their model ages fall between 0.76 Ga (Cryogenian) and 1.7 Ga (Satherian). One zircon giving a 1.9 Ga (Orosirian) crystallization age, has a εHf(t) value of −10 with a model age of 3.0 Ga (Mesoarchean).
4. Discussion
4.1. U-Pb ages and Lu-Hf isotopes of the clastics
The Triassic is an important time interval for both the İstanbul Zone and the Strandja Massif. In the Strandja Massif 2 different Triassic successions defined; Triassic volcanic and sedimentary rocks (Chatalov, Citation1990, Citation1991) and metaclastics with minor volcanics (Çağlayan & Yurtsever, Citation1998; Gočev, Citation1976, Citation1979; Natal’in et al., Citation2016, Citation2012; Okay et al., Citation2001). Furthermore, the İstanbul Zone also displays two different yet correlatable Triassic successions; one in the west, to the NW of İstanbul and the second one in the east, in the Kocaeli region.
First, we would like to discuss the age of the deposition of the rocks (Triassic and Silurian?) studied. The age of the Çiftalan Sandstone, exposed in the İstanbul area, previously reported as Scythian (Kaya & Lys, Citation1979-1980) based on the paleontological data from overlying Anisian limestone. The youngest detrital zircon age obtained from Çiftalan sandstone in this study is 167 ± 3 Ma (Middle Jurassic), but internal structure of this zircon is complex and the age has low concordance value (91%). Therefore, we consider the second youngest age 240 ± 3 Ma (Anisian) as more reliable to establish the maximum depositional age for this unit. Sample 139 was collected from a rock unit, which had been assigned to the Upper Ordovician-Lower Silurian Gözdağ Member of the Yayalar Formation (Özgül, Citation2012 and references herein). However, 8 of the 30 zircon ages gave late Carboniferous and younger ages and the youngest zircon age obtained from this sample is 252 ± 3 Ma. Its contact with the underlying Palaeozoic units is a thrust and there are no overlying units. This unit was most likely deposited during the Triassic but the full possible depositional range can be given as late Carboniferous-Permian to Cretaceous.
4.2. Provenance of the Triassic units, the StrandjaMassif or the İstanbul Zone?
The İstanbul Zone and the Strandja Massif, two possible source areas, differ from each other in terms of their magmatic and metamorphic histories. Presence of Ordovician, Lower Carboniferous magmatic rocks and the later late Jurassic-early Cretaceous metamorphism in the Strandja Massif distinguish it from the İstanbul Zone. Although the Permian magmatism is present in both units, the İstanbul Zone lacks the older ages. In the light of this information, the Strandja Massif is the best candidate to supply Palaeozoic zircons to the Triassic basin of İstanbul. The Triassic basin in İstanbul can be considered as a continuation of Triassic metasedimentary units of the Strandja Massif.
For the comparison, PDD diagrams have been created for the units studied here and the late Ordovician quartz arenites, early Carboniferous flysch and Permian granite intrusions of the İstanbul Zone (Okay et al., Citation2011; Ustaömer et al., Citation2011; Yılmaz Şahin et al., Citation2010) and the pre-Cambrian orthgneisses and the Palaeozoic basement schists of the Strandja Massif (Natal’in et al., Citation2016; Şahin et al., Citation2014; Sunal et al., Citation2006, Citation2008) (). It should be mentioned that we have only 25 ages from the sample 139 and it is difficult to be sure about the satisfactory comparison between it and the sample 107-b. Furthermore, ages obtained from these two samples confirm that although there are similarities in their Carboniferous and Permian ages, their εHf(t) values differ considerably revealing distinct origins (see ).
Figure 8. Probability density distribution diagrams and histograms of the U-Pb ages of the detrital and magmatic zircons, which obtained from the İstanbul Palaeozoic sequence, the Strandja Massif and this study.
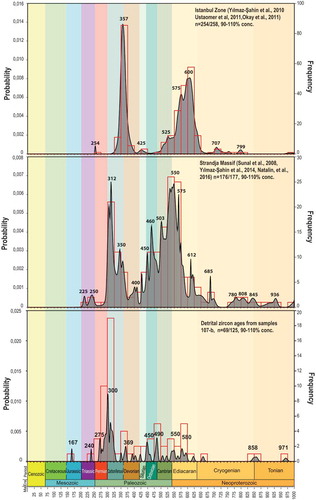
From the provenance perspective, the first similarity between the unit studied here and the Strandja Massif is the presence of a large number of Cambrian ages which represents the Pan-African events. No The Cambrian ages were described from the basement of the İstanbul Zone. There are only ages from detrital zircon grains (6%) (Okay et al., Citation2011; Ustaömer et al., Citation2011). However, Cambrian ages (544 Ma) were reported both from the orthogneisses and the detrital zircons of the Strandja Massif (Natal’in et al., Citation2016; Sunal et al., Citation2008). The Ediacaran ages of the İstanbul Zone has a higher percentage than in the Triassic rocks studied here, which shows that the Ediacaran was not the main source for the Triassic rocks. Besides, Ediacaran rocks were described both from the Strandja Massif (Natal’in et al., Citation2016) and from the basement of the İstanbul Zone (Chen et al., Citation2002).
Detrital zircon ages and their distribution show that Triassic clastics represented by the sample 107-b could not have been fed from the İstanbul Zone because of the presence of Upper Carboniferous representing Hercynian orogenic events and Permian ages. In contrast, it perfectly matches with the Strandja Massif when this interval plus distribution of the ages older than early Carboniferous are considered. shows the percentage of age distributions in specific geological time periods for ages older than the late Carboniferous for the sample 107-b, the Strandja Massif and the İstanbul Zone. Even if the late Carboniferous and Permian ages are excluded from the Triassic clastics of the Çiftalan sandstone, pie charts clearly show close similarity with the Strandja Massif rather than with the İstanbul Zone. Almost continuous age distribution from the Ordovician to the early Permian require a source area or areas with synchronous arc magmatism, which was already proposed for the Strandja Massif by Natal’in et al. (Citation2012). However, there is no direct indication of magmatism between the Ordovician and the late Carboniferous. Only some detrital ages from the Lower Carboniferous unit reveal that there are early Carboniferous zircons supplied to the basin (Okay et al., Citation2011).
Figure 9. Pie charts, which show the percentage of the distribution of the zircon ages in specific age groups.
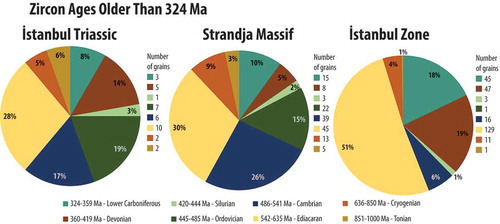
Figure 10. Outcrop represent the tectonic boundary between the İstanbul zone and the Strandja Massif. UTM WGS84 Coordinates: 0666761–4,564,893.
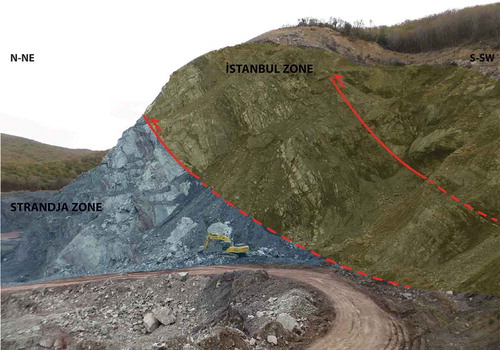
Zircon internal structures for both of the samples indicate that source area of the zircons are magmatic. Zircon crystals are prismatic and reveal good magmatic oscillatory zoning. In addition, high Th/U ratios support a magmatic origin for the zircons. Presence of resorption structures in the rims of the dated Ordovician zircon grains and their absence in the Carboniferous ones indicate that they came from a source that was metamorphosed sometime between the Ordovician and the Carboniferous.
In Şengör et al. (Citation1984), the İstanbul Zone is considered as a northward thrusting nappe over the Strandja Massif. Kaya and Lys (Citation1979-1980) indicate that Carboniferous flysch of İstanbul Zone is thrust over the Triassic rocks and our personal observations also confirm this relationship. illustrates thrusting of the İstanbul Zone onto the Strandja Massif as suggested by Şengör et al. (Citation1984).
Sandstone and siltstone alternation of the alleged Silurian west of Sarıyer is definitely not a part of the İstanbul Palaeozoic sequence. Both the sample 139 and the sample 107-b represent of Upper Carboniferous and Permian rocks. However, as pointed out above, the εHf(t) values of the two samples differ from each other. εHf(t) values of the sample 107-b mainly grouped around the CHUR line in the diagram () indicating that zircons transformed from rocks that are products of a continental margin arc magmatism where mature and juvenile crustal material mixed. In contrast, zircons from the sample 139 mainly show positive εHf(t) values for Permian and Ediacaran intervals. This data show that zircons collected from the sample 139 must be a product of a juvenile crust. Model ages of these two samples also show differences. The sample 139 has younger model ages such as 0,65 Ga, whereas the youngest model age in the sample 107-b is 0,92 Ga. Most probably, source areas of these two units were different.
5. Conclusions
Clastic rocks exposed in the northwest of Bosphorus have been assigned to the Upper Ordovician-Lower Silurian in previous studies. Present study revealed that these clastic rocks have a full range of Permian detrital zircons and the deposition age of this unit must be sometime between the Permian and the late Cretaceous.
Analyzed detrital zircon ages show that provenance of the early Triassic units in the western part of the İstanbul Zone is a continuation of the Strandja Massif.
In this region boundary between the İstanbul Zone and the Strandja Massif is tectonic. The İstanbul Zone was thrust over the Strandja Massif sometime after the Triassic. The age of thrusting remains unclear, but was most likely medial to late Mesozoic.
Before the Triassic the İstanbul Zone was located to the south of the Strandja Massif before its movement to north (present geographical positions).
supplementary.zip
Download Zip (676.1 KB)Acknowledgements
This study is supported by TÜBİTAK research grant No:113Y08. We thank to Evren Çubukçu and personnel of the Electron Microscopy Laboratory in Hacettepe University for help with the taking of cathodoluminescence images. We sincerely thank to Timur Ustaömer for constructive comments on U-Pb dating and Lu-Hf analyses. SCÜ thanks to Zehra Deveci Aral for her support on the evaluation of the data, Richard Albert Ropert and Linda Marko for there helps during isotope analyses and data processing and collaboration at GUF.
Disclosure statement
No potential conflict of interest was reported by the authors.
Supplementary Material
Supplemental data for this article can be accessed here
Additional information
Funding
References
- Aydın, Y., (1974). Etude petrographique et geochimique de la partie centrale du Massif d’Istranca (Turquie). PhD Thesis, Université de Nancy, Nancy.
- Aydın, Y., (1982). Geology of the Yıldız (Istranca) Mountains. PhD Thesis, Technical University, İstanbul.
- Blichert-Toft, J., & Albarède, F. (1997). The Lu-Hf isotope geochemistry of chondrites and the evolution of the mantle-crust system. Earth and Planetary Science Letters, 148(1–2), 243–258.
- Bouvier, A., Vervoort, J., & Patchett, P. (2008). The Lu–Hf and Sm–Nd isotopic composition of CHUR: constraints from unequilibrated chondrites and implications for the bulk composition of terrestrial planets. Earth and Planetary Science Letters, 273, 48–57.
- Burchfiel, B. C. (1980). Eastern European Alpine system and the Carpathian orocline as an example of collision tectonics. Tectonophysics, 63(1–4), 31–61.
- Bürküt, Y. (1976). Kuzeybatı Anadolu’da yeralan plütonların mukayeseli-jenetik etüdü. PhD Thesis, İTÜ Maden Fakültesi, İstanbul [In Turkish]
- Çağlayan, A., Şengün, M., & Yurtsever, A. (1988). Main fault systems shaping the Istranca Massif, Turkey. Journal of Pure and Applied Science, Series A, Geosciences, 21, 145–154.
- Çağlayan, M. A., & Yurtsever, A. (1998). 1:100,000 Ölçekli, Türkiye Jeoloji Haritaları, no. 20, 21, 22, 23, Burgaz-A3, Edirne-B2 ve B3, Burgaz-A4 ve Kırklareli-B4; Kırklareli-B5 ve B6; Kırklareli-C6 Paftaları [Geological Map of Turkey at 1: 100,000 Scale, no. 20, 21, 22, 23, Burgaz-A3, Edirne-B2 ve B3, Burgaz-A4 ve Kırklareli-B4; Kırklareli-B5 ve B6; Kırklareli-C6 Sheets]. Ankara: Mineral Research and Exploration Institute (MTA) of Turkey Publications [in Turkish with English abstract].
- Chatalov, G. (1990). Geology of the Strandja zone in Bulgaria. In Publishing House of the Bulgarian Academy of Sciences, Sofia (Vol. 4, pp. 272).
- Chatalov, G. (1991). Triassic in Bulgaria—A review. Bulletin of the Technical University of Istanbul, 44(1–2), 103–135.
- Chauvel, C., & Blichert-Toft, J. (2001). A hafnium isotope and trace element perspective on melting of the depleted mantle. Earth and Planetary Science Letters, 190(3), 137–151.
- Chauvel, C., Lewin, E., Carpentier, M., Arndt, N. T., & Marini, J. (2008). Role of recycled oceanic basalt and sediment in generating the Hf–Nd mantle array. Nature Geoscience, 1(1), 64–67.
- Chen, F., Siebel, W., Satir, M., Terzioğlu, M., & Saka, K. (2002). Geochronology of the karadere basement (NW Turkey) and implications for the geological evolution of the istanbul zone. International Journal of Earth Sciences, 91(3), 469–481. doi:10.1007/s00531-001-0239-6
- Cohen, K. M., Finney, S. C., Gibbard, P. L., & Fan, J.-X. 2013 ( updated). The ICS International Chronostratigraphic Chart. Episodes, 36, 199–204.
- Gedik, İ., Timur, E., Duru, M., & Pehlivan, Ş. (2005). 1:50000 ölçekli Türkiye Jeoloji Haritaları No: 10 İstanbul - F 22d paftası. In [Geological map of Turkey at 1:50000 scale No: 10 İstanbul – F 22d Sheet]. Ankara: Maden Tetkik ve Arama Genel Müdürlüğü Jeoloji Etütleri Dairesi.
- Gerdes, A., & Zeh, A. (2006). Combined U–Pb and Hf isotope LA-(MC-)ICP-MS analyses of detrital zircons: Comparison with SHRIMP and new constraints for the provenance and age of an Armorican metasediment in Central Germany. Earth and Planetary Science Letters, 249(1–2), 47–61.
- Gerdes, A., & Zeh, A. (2009). Zircon formation versus zircon alteration — New insights from combined U–Pb and Lu–Hf in-situ LA-ICP-MS analyses, and consequences for the interpretation of Archean zircon from the central zone of the limpopo belt. Chemical Geology, 261(3–4), 230–243.
- Gočev, P. (1976). New data on the fault tectonics of Bulgaria and part of the Balkan Peninsula obtained from the ERTS-1 space photographs. Geologica Balcanica, 6(4), 57–76.
- Gočev, P. (1979). The position of Strandja in the alpine structure of the Balkan Peninsula. Review of the Bulgarian Geological Society, 40, 27–76.
- Gočev, P. (1991). The Alpine orogen in the Balkans–A polyphase collisional structure. Geotectonics, Tectonophys. And Geodinamics, 22, 3–44.
- Görür, N., Monod, O., Okay, A. I., Sengör, C., Tüysüz, O., Yiğitbaş, E., … Akkök, R. (1997). Palaeogeographic and tectonic position of the Carboniferous rocks of the western Pontides (Turkey) in the frame of the Variscan belt. Bulletin De La Société Géologique De France, 168, 197–206.
- Kaya, O., & Lys, M. (1979-1980). Triassic on the western side of Bosphorus (Kilyos, İstanbul). a Recent Discovery. Bulletin of the Mineral Research and Exploration Institute of Turkey, Foreign Edition, 93–94, 20–26.
- Ketin, İ. (1966). Tectonic units of Anatolia. Bulletin of the Mineral Research and Exploration, 66, 23–34.
- Ketin, İ. (1982). Genel Jeoloji [General Geology]. İstanbul. İTÜ Maden Fakültesi Yayınları, 2, 216.
- Lom, N., Ülgen, S. C., Sakınç, M., & Şengör, A. M. C. (2016). Geology and stratigraphy of Istanbul region. Geodiversitas, 38(2), 175–195.
- Ludwig, K. R. (2003). Isoplot 3.00: A geochronological toolkit for Microsoft Excel. Berkeley, California: Berkeley Geochronological Center.
- Natal’in, B., Satır, M., Sunal, G., & Toraman, E., (2005b). Structural and metamorphic evolution of the Strandja massif: The Scientific and Technological Research Council of Turkey, Project No: 101Y010. Ankara-Turkey [in Turkish with English abstract, unpublished].
- Natal’in, B., Sunal, G., & Toraman, E. (2005a). The Strandja arc: Anatomy of collision after long-lived arc parallel tectonic transport. In E. V. Sklyarov (Ed.), The strandja arc: Anatomy of collision after long-lived arc parallel tectonic transport (pp. 240–245). Irkutsk: IEC SB RAS.
- Natal’in, B. A., Sunal, G., Gün, E., Wang, B., & Zhiqing, Y. (2016). Precambrian to Early Cretaceous rocks of the Strandja Massif (northwestern Turkey): Evolution of a long lasting magmatic arc. Canadian Journal of Earth Sciences, 53(11), 1312–1335.
- Natal’in, B. A., Sunal, G., Satir, M., & Toraman, E. (2012). Tectonics of the Strandja Massif, NW Turkey: History of a long-lived arc at the northern margin of Palaeo-Tethys. Turkish Journal of Earth Sciences, 21, 755–798.
- Okay, A., Satır, M., Tüysüz, O., Akyüz, S., & Chen, F. (2001). The tectonics of the Strandja Massif: Late-Variscan and mid-Mesozoic deformation and metamorphism in the northern Aegean. International Journal of Earth Sciences, 90(2), 217–233.
- Okay, A. I., Şengör, A. M. C., & Görür, N. (1994). Kinematic history of the opening of the Black Sea and its effect on the surrounding regions. Geology, 22(3), 267–270.
- Okay, A. I., Sunal, G., Sherlock, S., Altıner, D., Tüysüz, O., Kylander-Clark, A. R. C., & Aygül, M. (2013). Early cretaceous sedimentation and orogeny on the active margin of Eurasia: Southern central pontides, Turkey. Tectonics, 32, 1247–1271.
- Okay, N., Zack, T., Okay, A. I., & Barth, M. (2011). Sinistral transport along the Trans-European suture zone: Detrital zircon–Rutile geochronology and sandstone petrography from the carboniferous flysch of the pontides. Geological Magazine, 148(3), 380–403.
- Özgül, N. (2011). İstanbul İl Alanının Jeolojisi [Geology of the İstanbul munucipalty area] (pp. ix+89). İstanbul: İstanbul Büyükşehir Belediyesi, Planlama ve İmar Daire Başkanlığı Zemin ve Deprem İnceleme Müdürlüğü.
- Özgül, N. (2012). Stratigraphy and some structural features of the İstanbul Paleozoic. Turkish Journal of Earth Sciences, 21(6), 817–866.
- Pamir, H., & Baykal, F., (1947). Geological structure of Strandja Massif (Report No. 2257). Ankara.
- Rudnick, R. L., & Gao, S. (2003). Composition of the continental crust. In R. L. Rudnick (Ed.), The crust: Treatise on geochemistry, 3 (pp. 1–64). Oxford: Elsevier-Pergamon.
- Şahin, S. Y., Aysal, N., Güngör, Y., Peytcheva, I., & Neubauer, F. (2014). Geochemistry and U–Pb zircon geochronology of metagranites in Istranca (Strandja) Zone, NW Pontides, Turkey: implications for the geodynamic evolution of Cadomian orogeny. Gondwana Research, 26(2), 755–771.
- Santos, M. M., Lana, C., Scholz, R., Buick, I., Schmitz, M. D., Kamo, S. L., & Gerdes, A. (2017). A new appraisal of Sri Lankan BB zircon as a reference material for LA-ICP-MS U-Pb Geochronology and Lu-Hf Isotope Tracing. Geostandards and Geoanalytical Research, 41(3), 335–358.
- Şengör, A., Yılmaz, Y., & Sungurlu, O. (1984). Tectonics of the Mediterranean Cimmerides: Nature and evolution of the western termination of Palaeo-Tethys. Geological Society, London, Special Publications, 17(1), 77–112.
- Şengör, A. M. C. (1984). The Cimmeride orogenic system and the tectonics of Eurasia. Geological Society of America Special Papers, 195, 1–74.
- Şengör, A. M. C., & Özgül, N. (2010). İstanbul’un Jeolojisi [Geology of İstanbul]. In İstanbul Ansiklopedisi. İstanbul: NTV Yayınları.
- Şengör, A. M. C., & Yilmaz, Y. (1981). Tethyan evolution of Turkey: A plate tectonic approach. Tectonophysics, 75(3–4), 181–190, 193–199, 203–241.
- Sircombe, K. N. (2004). Agedisplay: An EXCEL workbook to evaluate and display univariate geochronological data using binned frequency histograms and probability density distributions. Computers & Geosciences, 30(1), 21–31. doi:10.1016/j.cageo.2003.09.006
- Sláma, J., Košler, J., Condon, D. J., Crowley, J. L., Gerdes, A., Hanchar, J. M., … Whitehouse, M. J. (2008). Plešovice zircon — A new natural reference material for U–Pb and Hf isotopic microanalysis. Chemical Geology, 249(1–2), 1–35.
- Sunal, G., Natal’in, B. A., Satir, M., & Toraman, E. (2006). Paleozoic magmatic events in the Strandja Massif, NW Turkey. Geodinamica Acta, 19(5), 283–300.
- Sunal, G., Satir, M., Natal’in, B., Topuz, G., & Vonderschmidt, O. (2011). Metamorphism and diachronous cooling in a contractional orogen: The Strandja Massif, NW Turkey. Geological Magazine, 148(4), 580–596.
- Sunal, G., Satir, M., Natal’in, B. A., & Toraman, E. (2008). Paleotectonic position of the Strandja Massif and surrounding continental blocks based on zircon Pb-Pb age studies. International Geology Review, 50(6), 519–545.
- Türkecan, A., & Yurtsever, A. (2002). Geological map of Turkey: Istanbul. Ankara: General Directorate of Mineral Research and Exploration.
- Ustaömer, P. A., Mundil, R., & Renne, P. R. (2005). U/Pb and Pb/Pb zircon ages for arc‐related intrusions of the bolu massif (W Pontides, NW Turkey): Evidence for late precambrian (Cadomian) age, Terra Nova, 17(3), 215–223.
- Ustaömer, P. A., Ustaömer, T., Gerdes, A., & Zulauf, G. (2011). Detrital zircon ages from a lower ordovician quartzite of the Istanbul exotic terrane (NW Turkey): Evidence for Amazonian affinity. International Journal of Earth Sciences, 100(1), 23–41.
- Wiedenbeck, M., Allé, P., Corfu, F., Griffin, W. L., Meier, M., Oberli, F., … Spiegel, W. (1995). Three natural zircon standards for u-Th-Pb. Lu-Hf, Trace Elementand REE Analyses Geostand Geoanal Researcher, 19(1), 1–23.
- Yilmaz, I. (1977). The absolute age and genesis of the sancaktepe granite (Kocaeli peninsula). Türkiye Jeoloji Kurumu Bülteni, 20, 17–20.
- Yılmaz Şahin, S., Aysal, N., & Güngör, Y. (2010). Petrogenesis and SHRIMP zircon U-Pb dating of some granitoids within the western pontides, southeastern balkans, NW Turkey, Proceedings Geologica Balcanica, XIX Congress of the Carpathian-Balkan Geological association (pp. 273). Thessaloniki, Greece: Abstracts, Bulgarian Academy Sciences.
- Zeh, A., & Gerdes, A. (2012). U-Pb and Hf isotope record of detrital zircons from gold-bearing sediments of the pietersburg greenstone belt (South Africa) – Is there a common provenance with the witwatersrand basin? Precambrian Research, 204–205, 46–56.