Abstract
Objectives: We aimed to identify the potential HOXB4/HOXC4 downstream effectors and elucidate their regulatory mechanism in the expansion of hematopoietic stem cell (HSC).
Methods: The microarray data GSE24379 were downloaded from Gene Expression Omnibus database, including 12 human CD34+ hematopoietic cells with irradiated EGFP-, HOXB4-, or HOXC4-transduced MS-5 cells, respectively. Then common differentially expressed genes (DEGs) in HOXB4- and HOXC4-treated hematopoietic cells (HOXB4&HOXC4.DEGs) were screened out. Protein–protein interaction (PPI) network was constructed and functional modules analysis was performed. Pathway enrichment analysis was performed using the Database for Annotation Visualization and Integrated Discovery. Besides, transcription regulatory network (TRN) was constructed to screen transcription factors (TFs) corresponding to HOXB4&HOXC4.DEGs.
Results: A total of 408 HOXB4&HOXC4.DEGs (373 up- and 35 down-regulated) in hematopoietic cells were identified. Tumor protein p53 (TP53) had the highest degrees in PPI network. Cyclin B1 (CCNB1) was a hub node in Cluster 1. V-myc avian myelocytomatosis viral oncogene homolog (MYC) and MYC-associated factor X (MAX) were important TFs with higher degrees. Meanwhile, MYC, TP53, and CCNB1 were significantly enriched in cell cycle.
Conclusion: MYC, MAX, TP53, and CCNB1 may be crucial HOXB4/HOXC4 downstream molecules potentially involved in HSCs expansion, and HOXB4 and HOXC4 homeoprotein could display positive effects on expansion of human HSCs via regulating these genes.
Introduction
The multipotent hematopoietic stem cells (HSCs), the common ancestor of all types of blood cells, possess the ability of both self-renewal and differentiation according to body needs.Citation1,Citation2 Expansion of HSCs underlie the basis of gene therapy and various genetic diseases of blood cells have shown to be successfully treated with HSC transplantation (HSCT).Citation3 Given the propensity of HSCs to remain quiescent, gene therapy applications and the translational use of them in transplantation biology remain a great challenge.Citation4 Until now, the mechanisms that control self-renewal and hematopoietic differentiation are still ambiguous, a better understanding of the biological nature of HSCs and molecular mechanisms underlying HSC expansion will have significant impact on transplantation and current gene therapy strategies.
Recently, numerous studies have implicated that a variety of transcription factors (TFs) are important regulatory components of HSC expansion, especially Hox homeobox gene family.Citation5,Citation6 HOXB4 appears to be a strong positive regulator of HSCs self-renewal, and overexpression of HOXB4 significantly promotes HSCs regeneration and expansion.Citation7–Citation9 A degradation-resistant HOXB4 variant is also shown to markedly improve ex vivo expansion of adult HSCs.Citation10 It has also been suggested that HOXB4 can induce stable gene expression changes in transplanted HSCs that are often involved in balanced self-renewal and differentiation divisions.Citation11 Moreover, a previous study also confirms that HOXB4 confers definitive lymphoid-myeloid engraftment potential on HSCs derived from yolk sac hematopoietic progenitors or embryonic stem cell.Citation12 Besides, genetic alterations of HSC caused by retrovirus-mediated constitutive expression of human HOXB4 is likely to be hazardous for therapeutic applications.Citation13 In addition to HOXB4, HOXC4, a paralog of the HOXB4, has been shown to be able to induce an in vitro expansion of clonogenic and early progenitors after retroviral transduction of HOXC4 into human CD34+ cells.Citation14 Also, the work of Auvray et al. demonstrated that HOXC4 homeoprotein efficiently expanded human HSCs and triggered similar molecular alterations as HOXB4, in which microarray GSE24379 was used.Citation13 Progresses have been achieved in understanding the biological nature of HSCs; however, the molecular mechanisms of HOXB4 or HOXC4-mediated benign HSCs expansion are mostly unknown, and the present knowledge seems to be insufficient.
In the present study, we used microarray data GSE24379 to explore potential effectors of HOXB4 and HOXC4 in human CD34+ hematopoietic cells following exposure to these factors by biological informatics approach. Since HOXB4 and HOXC4 displayed important molecular analogies and had similar temporal and spatial expression patterns during embryogenesis, common differentially expressed genes (DEGs) with the same expression pattern in HOXB4- and HOXC4-treated CD34+ cells (HOXB4&HOXC4.DEGs) were screened out. Additionally, functional enrichment analysis were performed, and transcription regulatory network (TRN) were constructed for exploring TFs corresponding to these DEGs. We aimed to give a systematic perspective to elucidate the key mechanism and identify the potential HOX downstream effectors involved in HSCs expansion.
Materials and methods
Microarray data
The gene expression profiling of GSE24379 deposited by Auvray et al.Citation13 was downloaded from Gene Expression Omnibus database (http://www.ncbi.nlm.nih.gov/geo/). A total of 12 CD34+ hematopoietic cell samples were used for the development of this microarray data. Human CD34+ hematopoietic cells co-cultured for 24 hours with irradiated GFP-, HOXB4-, or HOXC4-transduced MS-5 cells respectively (CD34+/MS-5/GFP, CD34+/MS-5/HOXB4, or CD34+/MS-5/HOXC4). Every two treatments were randomly assigned into the same group and consequently three groups were obtained, as follows: CD34+/MS-5/HOXB4 vs. CD34+/MS-5/HOXC4, CD34+/MS-5/HOXB4 vs. CD34+/MS-5/GFP, and CD34+/MS-5/HOXC4 vs. CD34+/MS-5/GFP (n = 4 per group; total = 12). In addition, aminoallyl-dUTP of samples were incorporated for indirect labeling with Cy3 and Cy5, three competitive hybridizations in this array design were performed in duplicate using a dye-swap strategy. The raw data and annotation files were downloaded based on the platform of GPL4803 (CEA – DSV/DRR/SGF: Human 25 k oligo v1.0) for subsequent analysis.
Data preprocessing and DEGs screening
All the array data were preprocessed using LOESSCitation15 normalization. We then identified DEGs in CD34+ cells co-cultured with MS-5/HOXB4 and co-cultured MS-5/HOXC4 using limmaCitation16 in R package, respectively. The significant P-value was adjusted by Benjamini and Hochberg (BH) method.Citation17 Then the adjusted P-value < 0.05 and |log fold change (FC)| ≥ 0.5 were considered as the cutoff value.
In addition, the common DEGs in HOXB4- and HOXC4-treated CD34+ cells with the same expression pattern (high expression or low expression) were considered as HOXB4&HOXC4.DEGs. These HOXB4&HOXC4.DEGs were used for subsequent analysis.
Pathway enrichment analysis
Kyoto Encyclopedia of Genes and Genomes (KEGG)Citation18 is a pathway-related database for classification of related gene sets into their respective pathways. The BiocCartaCitation19 website is an interactive online resource which mainly divides into three categories: gene function, proteomic pathway, and reagent exchange. Database for Annotation Visualization and Integrated Discovery (DAVID)Citation20 is a tool for providing functional annotation behind large-scale genomic or transcriptomic data.
In this study, KEGG and BiocCarta pathway enrichment analysis for HOXB4&HOXC4.DEGs were performed by DAVID online analytical tools. The P-value < 0.05 was set as the threshold value.
Protein–protein interaction (PPI) network construction and functional modules analysis
Search Tool for the Retrieval of Interacting Genes (STRING)Citation21 database provides comprehensive information of both predicted and experimental interactions of proteins. The PPI pairs in STRING database were displayed with a combined score. The HOXB4&HOXC4.DEGs were mapped into PPIs with combined score ≥ 0.7 as the significant cutoff value. Then PPI network was then built using CytoscapeCitation22 software. Besides, the significant modules of PPI network with score cutoff = 0.2 were screened out using Molecular Complex Detection (MCODE)Citation23 plugin of Cytoscape. Then cluster analysis for assessing the function modules of PPI network was performed by Biological Networks Gene Ontology (BiNGO)Citation24 plugin of Cytoscape. The P-value was adjusted by false discovery rate (FDR), and FDR < 0.05 was defined as significant.
TRN construction
ENCyclopedia of DNA Elements (ENCODE)Citation25 database provides all functional elements in the human genome sequence and TF binding sites. In our study, we first extracted all the information of human TF binding sites based on the information of ENCODE database. Then TF binding site existed in at least two independent samples was identified and used for subsequent analysis. According to the annotation information of transcription region, we further identified the TFs located in promoter region, a region of 1.5 kb around transcriptional start sites (TSS) with 1 kb upstream and 0.5 kb downstream. Then the corresponding target genes regulated by these TFs were obtained. With the information of TFs-target genes pairs, the TRN of DEGs was constructed.
Results
DEGs screening
In CD34+/MS-5/HOXB4 vs. CD34+/MS-5/HOXC4 groups, only 28 DEGs (14 up- and 14 down-regulated) were identified. However, we identified a total of 547 DEGs (478 up- and 69 down-regulated) in CD34+/MS-5/HOXB4 vs. CD34+/MS-5/GFP group and 718 DEGs (642 up- and 76 down-regulated) in CD34+/MS-5/HOXC4 vs. CD34+/MS-5/GFP group. The results showed that the numbers of up-regulated genes were significantly more than down-regulated genes no matter in HOXB4-treated or HOXC4-treated CD34+ cells.
Besides, as shown in Fig. , totally 408 overlaps (HOXB4&HOXC4.DEGs) were identified. Among them, 373 DEGs, such as Heat Shock 70 kDa Protein 1A (HSPA1A), Arrestin, Beta 1 (ARRB1), ATM Serine/Threonine Kinase (ATM), Chromodomain Helicase DNA Binding Protein 4 (CHD4), Cullin 4A (CUL4A), and Prosaposin (PSAP) were up-regulated (Fig. A) and 35 DEGs, like HMG-Box Transcription Factor 1 (HBP1), Myocyte Enhancer Factor 2C (MEF2C), Dachshund Family Transcription Factor 1 (DACH1) and Cyclin-Dependent Kinase Inhibitor 1C (P57, Kip2) (CDKN1C), were down-regulated (Fig. B). These HOXB4&HOXC4.DEGs were considered as key HOXB4/HOXC4 downstream molecules used for further analysis.
Pathway enrichment analysis
Based on the information of KEGGCitation18 database and the BiocCartaCitation19 website, five KEGG pathways and five Biocarta pathways were enriched by 408 HOXB4&HOXC4.DEGs respectively with P-value <0.05 (Table ). The enriched KEGG pathways were Cell cycle, Spliceosome, DNA replication, Citrate cycle (TCA cycle), and Progesterone-mediated oocyte maturation (Table A). The enriched Biocarta pathways were Regulation of eIF2, Cyclins and Cell Cycle Regulation, G1/S Check Point, CDK Regulation of DNA Replication, and ATM Signaling Pathway (Table B).
Table 1 The KEGG and Biocarta pathways enriched by 408 common DEGs in HOXB4/HOXC4-treated cells (HOXB4&HOXC4.DEGs)
PPI network construction and functional modules analysis of network
Based on the information of STRINGCitation21 database, the HOXB4&HOXC4.DEGs were mapped into PPIs with combined score ≥ 0.7 as the significant cutoff value. Then PPI network constructed by HOXB4&HOXC4.DEGs contained 214 nodes and 664 edges (Fig. A). Thereinto, 201 nodes (red) were up-regulated genes and 13 nodes (green) were down-regulated gene. Based on nodes degrees, the top 10 nodes were tumor protein p53 (TP53), cyclin B1 (CCNB1), cell division cycle 6 (CDC6), cyclin A2 (CCNA2), RAD51 recombinase (RAD51), cyclin-dependent kinase 2 (CDK2), minichromosome maintenance complex component 4 (MCM4), cell division cycle 20 (CDC20), v-myc avian myelocytomatosis viral oncogene homolog (MYC), and minichromosome maintenance complex component 7 (MCM7). All these DEGs were up-regulated.
Figure 2 PPI network of HOXB4&HOXC4.DEGs (A) and two significant modules: Cluster 1 (B) and Cluster 2 (C). HOXB4&HOXC4.DEGs represent common DEGs in HOXB4/HOXC4-treated hematopoietic cells. Red nodes are up-regulated genes and green nodes are down-regulated ones. The lines stand for the interaction between genes. Nodes size represents their node degree.
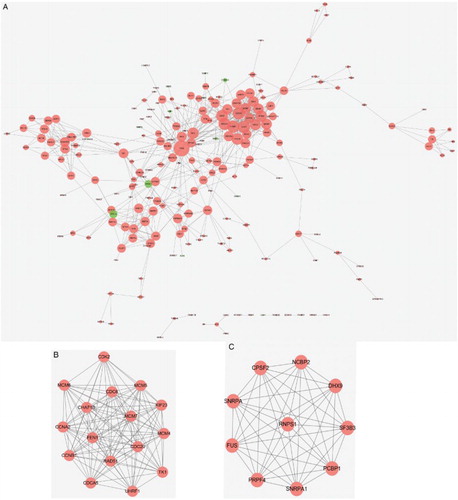
Additionally, using MCODE plugin of Cytoscape, a total of nine functional modules were identified with FDR <0.05 (Table ). The score of Cluster 1 and Cluster 2 were more than 10. Cluster 1 contains 16 nodes and 115 edges (Fig. B), and Cluster 2 contains 10 nodes and 45 edges (Fig. C). The hub nodes in Cluster 1 were CCNB1, CDC6, and CCNA2, while the hub nodes in Cluster 2 were nuclear cap binding protein subunit 2, 20 kDa (NCBP2), splicing factor 3b, subunit 3, 130 kDa (SF3B3) and fused in sarcoma (FUS). All these DEGs were also up-regulated genes.
Table 2 Total nine modules of PPI network classified by MCODE
TRN construction of HOXB4&HOXC4.DEGs
As shown in Fig. , TRN constructed by HOXB4&HOXC4.DEGs contained 329 edges and 236 nodes based on the information of ENCODECitation25 database. According to the annotation information of transcription region, the TFs located in promoter region, a region of 1.5 kb around TSS with 1 kb upstream and 0.5 kb downstream, 223 up-regulated nodes and 13 down-regulated ones were identified. Thereinto, five nodes were TFs (4 up- and 1 down-regulated). Up-regulated TFs were MYC, MYC-associated factor X (MAX), histone deacetylase 2 (HDAC2), and tripartite motif containing 28. The only down-regulated TF was nuclear receptor subfamily 3, group C, member 1 (NR3C1).
Figure 3 TRN of HOXB4&HOXC4.DEGs. HOXB4&HOXC4.DEGs represent common DEGs in HOXB4/HOXC4-treated hematopoietic cells. Red nodes represent up-regulated genes and green nodes are down-regulated ones. Square nodes represent TFs and circular nodes were not TFs. The lines stand for the interaction between genes.
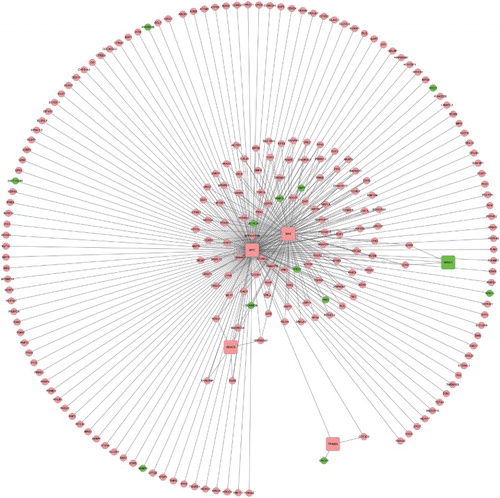
Discussion
Expansion of HSCs represents an important objective for gene therapy strategies.Citation13 In the current study, we used bioinformatics approach to explore the potential molecular mechanism involved in HOXB4/HOXC4-treated HSCs expansion. Consistent with the work of Auvray et al.Citation13 in which microarray GSE24379 was used, we also identified same up-regulated DEGs, such as HSPA1A, ARRB1, ATM, CHD4, CUL4A, and PSAP, and down-regulated DEGs, like HBP1, MEF2C, DACH1, and CDKN1C. Moreover, as a result of CD34+ cell exposure to HOXB4/C4 using different cell models, HSPA1A and HBP1 were also identified to be differentially expressed by Schiedlmeier et al.,Citation26 ARRB1and MEF2C were also identified by Palmqvist, Pineault, et al.,Citation27 CDKN1C and DACH1 were also identified by Forsberg et al.,Citation28 and ATM, CHD4, CUL4A, and PSAP were also identified by Kent et al.Citation29 In contrast to these previous findings, our results also identified other different DEGs. Among them, MYC and MAX were important TFs involved in HSCs expansion, and MYC interacted with MAX, TP53, and CCNB1 directly in TRN network. Meanwhile, MYC, TP53, and CCNB1 were significantly enriched in cell cycle and were regarded to be strongly associated with HOX homeoprotein in HSCs expansion.
MYC and MAX are members of basic helix-loop-helix leucine zipper TFs.Citation30 c-MYC is shown to control the balance between HSCs self-renewal and differentiation via regulating the interaction between HSCs and their niche including N-cadherin and a number of adhesion receptors.Citation31 The work of Laurenti et al. also confirmed that MYC activity (c-MYC and N-MYC) regulated crucial aspects of HSC function, such as proliferation and differentiation, and survival.Citation32 Moreover, c-MYC can promote self-renewal of HSCs as a downstream critical effector molecule of HOXB4.Citation33 In addition, increasing evidences suggest that the functions of the MYC/MAX/MAD network play roles in cell proliferation, differentiation, and death.Citation30 Auvray et al. have shown that c-MYC and its partner MAX are up-regulated in HSC exposed to HOXB4 or to HOXC4.Citation13 Because HOXB4/HOXC4 protein transfer is operative for expanding HSCs,Citation13 thereby, we speculate that MYC and MAX may be key common downstream effectors of HOXB4/HOXC4 and may play a crucial role in controlling the balance between HSCs self-renewal and differentiation.
Furthermore, MYC interacted with TP53 and CCNB1 directly in TRN network. TP53 was also identified from PPI network with the highest degrees compared to others. Numerous studies have identified critical roles of TP53 in HSCs behavior, such as self-renewal, differentiation, apoptosis, and aging.Citation34,Citation35 A study of Mysm1-deficient mice have shown that p53 is essential for HSC function and lymphopoiesis.Citation36 Moreover, TP53 plays an important role in steady-state hematopoiesis and is essential for the enhanced HSC quiescence seen in the absence of MEF.Citation37 Besides, TP53 is involved in stress hematopoiesis and regulates its target genes, variably inducing cell cycle arrest, apoptosis, or senescence, in response to diverse stresses.Citation38 During normal and stress hematopoiesis, the pathways that HOXB4 regulates HSC self-renewal also can be affected by TP53.Citation8,Citation39 Therefore, our results are in line with previous findings and suggest that TP53 may play a crucial role in hematopoiesis and thus regulate HOXB4/HOXC4-mediated HSCs expansion. In addition, CCNB1 was also identified as a hub node in Cluster 1 in our study. CCNB1 is likely to be privileged mediators of HSC differentiation-associated proliferation during the various phases of cytokine-mediated mobilization.Citation40 CCNB1 is also found to be strongly associated with the fate of HSCs and progenitor cell.Citation40 Thus, we speculate that CCNB1 may play crucial roles in the proliferation of HSCs following by exposure to HOXB4 or HOXC4.
Strikingly, MYC, TP53, and CCNB1 were significantly enriched in cell cycle. MYC protein is confirmed to induce cell cycle progression and apoptosis via dimerization with MAX.Citation41 TP53 participates in multiple cell cycle checkpoints and controls HSCs self-renewal.Citation42,Citation43 CCNB1 is a regulatory protein which is essential for the control of the cell cycle at the G2/M (mitosis) transition.Citation44 Furthermore, previous study demonstrated that HSCs proliferation is likely determined by the function and/or regulation of components and regulators of the cell cycle machinery.Citation40,Citation45 Appropriate cell cycle control is required for maintaining normal hematopoiesis, especially at the early stage of HSCs/progenitor cells.Citation46 Therefore, we further speculate that these proteins may play important roles in HSCs expansion via cell cycle.
Only 1 dataset with relatively small sample size was the limitation of our study. Moreover, there was no experimental validation, like shRNA approach, to validate the roles of key DEGs in HSCs expansion. Further study with more datasets and experiments are still needed to verify the findings of our study. Besides, chromatin immunoprecipitation followed by sequencing (ChIP–seq) is increasingly being used for mapping protein–DNA interactions in-vivo on a genome scale.Citation47 If ChIP–seq is performed to extract direct target genes of HOXB4/HOXC4 from DEGs lists, the obtained results will be more convincing.
In conclusion, MYC, MAX, TP53, and CCNB1 may be crucial HOXB4/HOXC4 downstream molecules potentially involved in HSCs expansion, and HOXB4 and HOXC4 homeoprotein may display positive effects on expansion of human HSCs by targeting these proteins. MYC may play important roles in controlling the balance between HSCs self-renewal and differentiation following by exposure to HOXB4 or HOXC4 via dimerization with MAX. TP53 may play important roles in regulating hematopoiesis and thus is involved in HOXB4- or HOXC4-mediated HSCs expansion. CCNB1 may play crucial roles in the proliferation of HOXB4/HOXC4-treated HSCs. Additionally, all these molecules may play important roles in HOXB4/HOXC4-mediated HSCs expansion via cell cycle. Our findings would aid in a better understanding of the molecular mechanism of HSCs expansion and provide broader perspective for the development of transplantation and gene therapy strategies.
Disclaimer statements
Contributors Chunlei Xin and Chunting Zhao conceived and designed the study. Xiangcong Yin and Shaoling Wu were responsible for data collection and analysis. Zhan Su was responsible for data interpreting. Chunlei Xin wrote the article. Chunting Zhao guaranteed the integrity of the data on this article.
Funding This work was supported by Natural Science Foundation of Shandong (ZR2009CM056).
Conflict of interest All authors declare that they have no conflict of interests to state.
Ethics approval These data were downloaded from common data base. So, the ethics approval is unnecessary for our study.
References
- Gunsilius E, Gastl G, Petzer A. Hematopoietic stem cells. Biomed Pharmacother. 2001;55(4):186–94. doi: 10.1016/S0753-3322(01)00051-8
- Seita J, Weissman IL. Hematopoietic stem cell: self-renewal versus differentiation. Wiley Interdiscip Rev Syst Biol Med. 2010;2(6):640–53. doi: 10.1002/wsbm.86
- Kohn DB, Pai S-Y, Sadelain M. Gene therapy through autologous transplantation of gene-modified hematopoietic stem cells. Biol Blood Marrow Transplant. 2013;19(1):S64–S69. doi: 10.1016/j.bbmt.2012.09.021
- Kunisaki Y, Frenette PS. The secrets of the bone marrow niche: Enigmatic niche brings challenge for HSC expansion. Nat Med. 2012;18(6):864–5. doi: 10.1038/nm.2825
- Alharbi RA, Pettengell R, Pandha HS, Morgan R. The role of HOX genes in normal hematopoiesis and acute leukemia. Leukemia. 2013;27(5):1000–8. doi: 10.1038/leu.2012.356
- Slany RK. Role of the Trithorax (MLL): HOX axis in HSC development, function, and leukemia. In: Bonifer C, Cockerill P, (ed.) Transcriptional and epigenetic mechanisms regulating normal and aberrant blood cell development. New York: Springer; 2014. p. 175–204.
- Antonchuk J, Sauvageau G, Humphries RK. HOXB4-induced expansion of adult hematopoietic stem cells ex vivo. Cell. 2002;109(1):39–45. doi: 10.1016/S0092-8674(02)00697-9
- Krosl J, Austin P, Beslu N, Kroon E, Humphries RK, Sauvageau G. In vitro expansion of hematopoietic stem cells by recombinant TAT-HOXB4 protein. Nat Med. 2003;9(11):1428–32. doi: 10.1038/nm951
- Antonchuk J, Sauvageau G, Humphries RK. HOXB4 overexpression mediates very rapid stem cell regeneration and competitive hematopoietic repopulation. Exp Hematol. 2001;29(9):1125–34. doi: 10.1016/S0301-472X(01)00681-6
- Lee J, Shieh J-H, Zhang J, Liu L, Zhang Y, Eom JY, et al. Improved ex vivo expansion of adult hematopoietic stem cells by overcoming CUL4-mediated degradation of HOXB4. Blood. 2013;121(20):4082–9. doi: 10.1182/blood-2012-09-455204
- Yu H, Neale G, Zhang H, Lee HM, Ma Z, Zhou S, et al. Downregulation of Prdm16 mRNA is a specific antileukemic mechanism during HOXB4-mediated HSC expansion in vivo. Blood. 2014;124(11):1737–47. doi: 10.1182/blood-2013-10-534735
- Kyba M, Perlingeiro RC, Daley GQ. HoxB4 confers definitive lymphoid-myeloid engraftment potential on embryonic stem cell and yolk sac hematopoietic progenitors. Cell. 2002;109(1):29–37. doi: 10.1016/S0092-8674(02)00680-3
- Auvray C, Delahaye A, Pflumio F, Haddad R, Amsellem S, Miri-Nezhad A, et al. HOXC4 homeoprotein efficiently expands human hematopoietic stem cells and triggers similar molecular alterations as HOXB4. Haematologica. 2012;97(2):168–78. doi: 10.3324/haematol.2011.051235
- Daga A, Podesta M, Capra MC, Piaggio G, Frassoni F, Corte G. The retroviral transduction of HOXC4 into human CD34+ cells induces an in vitro expansion of clonogenic and early progenitors. Exp Hematol. 2000;28(5):569–74. doi: 10.1016/S0301-472X(00)00135-1
- Zahurak M, Parmigiani G, Yu W, Scharpf RB, Berman D, Schaeffer E, et al. Pre-processing Agilent microarray data. BMC Bioinformatics. 2007;8(1):142–55. doi: 10.1186/1471-2105-8-142
- Smyth GK. Limma: linear models for microarray data. In: Gentleman R, Carey V, Huber W, Irizarry R, Dudoit S, (eds.) Bioinformatics and computational biology solutions using R and bioconductor. New York: Springer; 2005. p. 397–420.
- Benjamini Y, Hochberg Y. Controlling the false discovery rate: a practical and powerful approach to multiple testing. J R Stat Soc Series B. 1995;57(1):289–300.
- Kanehisa M. The KEGG database. Novartis Found Symp. 2002;247:91–103; discussion -3, 19–28, 244–52. doi: 10.1002/0470857897.ch8
- Nishimura D. BioCarta. Biotech Softw Internet Rep: Comput Softw J Sci. 2001;2(3):117–20.
- Da Wei Huang BTS, Lempicki RA. Systematic and integrative analysis of large gene lists using DAVID bioinformatics resources. Nat Protoc. 2008;4(1):44–57. doi: 10.1038/nprot.2008.211
- Franceschini A, Szklarczyk D, Frankild S, Kuhn M, Simonovic M, Roth A, et al. STRING v9. 1: protein-protein interaction networks, with increased coverage and integration. Nucleic Acids Res. 2013;41(D1):D808–15. doi: 10.1093/nar/gks1094
- Shannon P, Markiel A, Ozier O, Baliga NS, Wang JT, Ramage D, et al. Cytoscape: a software environment for integrated models of biomolecular interaction networks. Genome Res. 2003;13(11):2498–504. doi: 10.1101/gr.1239303
- Bader GD, Hogue CW. An automated method for finding molecular complexes in large protein interaction networks. BMC Bioinformatics. 2003;4(1):1471–98. doi: 10.1186/1471-2105-4-2
- Maere S, Heymans K, Kuiper M. BiNGO: a Cytoscape plugin to assess overrepresentation of gene ontology categories in biological networks. Bioinformatics. 2005;21(16):3448–9. doi: 10.1093/bioinformatics/bti551
- Consortium EP. The ENCODE (ENCyclopedia of DNA elements) project. Science. 2004;306(5696):636–40. doi: 10.1126/science.1105136
- Schiedlmeier B, Santos AC, Ribeiro A, Moncaut N, Lesinski D, Auer H, et al. HOXB4′s road map to stem cell expansion. Proc Natl Acad Sci. 2007;104(43):16952–7. doi: 10.1073/pnas.0703082104
- Palmqvist L, Pineault N, Wasslavik C, Humphries RK. Candidate genes for expansion and transformation of hematopoietic stem cells by NUP98-HOX fusion genes. PLoS One. 2007;2(1):e768–79. doi: 10.1371/journal.pone.0000768
- Forsberg EC, Prohaska SS, Katzman S, Heffner GC, Stuart JM, Weissman IL. Differential expression of novel potential regulators in hematopoietic stem cells. PLoS Genet. 2005;1(3):e281–94. doi: 10.1371/journal.pgen.0010028
- Kent DG, Copley MR, Benz C, Wöhrer S, Dykstra BJ, Ma E, et al. Prospective isolation and molecular characterization of hematopoietic stem cells with durable self-renewal potential. Blood. 2009;113(25):6342–50. doi: 10.1182/blood-2008-12-192054
- Grandori C, Cowley SM, James LP, Eisenman RN. The Myc/Max/Mad network and the transcriptional control of cell behavior. Annu Rev Cell Dev Biol. 2000;16(1):653–99. doi: 10.1146/annurev.cellbio.16.1.653
- Wilson A, Murphy MJ, Oskarsson T, Kaloulis K, Bettess MD, Oser GM, et al. c-Myc controls the balance between hematopoietic stem cell self-renewal and differentiation. Genes Dev. 2004;18(22):2747–63. doi: 10.1101/gad.313104
- Laurenti E, Varnum-Finney B, Wilson A, Ferrero I, Blanco-Bose WE, Ehninger A, et al. Hematopoietic stem cell function and survival depend on c-Myc and N-Myc activity. Cell Stem Cell. 2008;3(6):611–24. doi: 10.1016/j.stem.2008.09.005
- Satoh Y, Matsumura I, Tanaka H, Ezoe S, Sugahara H, Mizuki M, et al. Roles for c-Myc in self-renewal of hematopoietic stem cells. J Biol Chem. 2004;279(24):24986–93. doi: 10.1074/jbc.M400407200
- Dumble M, Moore L, Chambers SM, Geiger H, Van Zant G, Goodell MA, et al. The impact of altered p53 dosage on hematopoietic stem cell dynamics during aging. Blood. 2007;109(4):1736–42. doi: 10.1182/blood-2006-03-010413
- Shounan Y, Dolnikov A, MacKenzie K, Miller M, Chan Y, Symonds G. Retroviral transduction of hematopoietic progenitor cells with mutant p53 promotes survival and proliferation, modifies differentiation potential and inhibits apoptosis. Leukemia. 1996;10(10):1619–28.
- Belle JI, Langlais D, Petrov JC, Pardo M, Jones RG, Gros P, et al. p53 mediates loss of hematopoietic stem cell function and lymphopenia in Mysm1 deficiency. Blood. 2015;125(15):2344–8. doi: 10.1182/blood-2014-05-574111
- Liu Y, Elf SE, Miyata Y, Sashida G, Liu Y, Huang G, et al. p53 regulates hematopoietic stem cell quiescence. Cell Stem Cell. 2009;4(1):37–48. doi: 10.1016/j.stem.2008.11.006
- Liu Y, Elf SE, Asai T, Miyata Y, Liu Y, Sashida G, et al. The p53 tumor suppressor protein is a critical regulator of hematopoietic stem cell behavior. Cell Cycle. 2009;8(19):3120–4. doi: 10.4161/cc.8.19.9627
- Park I-K, Qian D, Kiel M, Becker MW, Pihalja M, Weissman IL, et al. Bmi-1 is required for maintenance of adult self-renewing haematopoietic stem cells. Nature. 2003;423(6937):302–5. doi: 10.1038/nature01587
- Passegué E, Wagers AJ, Giuriato S, Anderson WC, Weissman IL. Global analysis of proliferation and cell cycle gene expression in the regulation of hematopoietic stem and progenitor cell fates. J Exp Med. 2005;202(11):1599–611. doi: 10.1084/jem.20050967
- Amati B, Littlewood T, Evan G, Land H. The c-Myc protein induces cell cycle progression and apoptosis through dimerization with Max. EMBO J. 1993;12(13):5083–7.
- Giono LE, Manfredi JJ. The p53 tumor suppressor participates in multiple cell cycle checkpoints. J Cell Physiol. 2006;209(1):13–20. doi: 10.1002/jcp.20689
- Gonzalez S. miR-33-mediated downregulation of p53 controls hematopoietic stem cell self-renewal. Cell Cycle. 2010;9(16):3277–85.
- Sánchez I, Dynlacht BD, editors. New insights into cyclins, CDKs, and cell cycle control. Seminars in cell & developmental biology. New York: Academic Press; 2005.
- Sherr CJ, Roberts JM. Living with or without cyclins and cyclin-dependent kinases. Genes Dev. 2004;18(22):2699–711. doi: 10.1101/gad.1256504
- Ezoe S, Matsumura I, Satoh Y, Tanaka H, Kanakura Y. Cell cycle regulation in hematopoietic stem/progenitor cells. Cell Cycle. 2004;3(3):314–8. doi: 10.4161/cc.3.3.710
- Jothi R, Cuddapah S, Barski A, Cui K, Zhao K. Genome-wide identification of in vivo protein–DNA binding sites from ChIP-Seq data. Nucleic Acids Res. 2008;36(16):5221–31. doi: 10.1093/nar/gkn488