Abstract
Objective: Myelodysplastic syndromes (MDS) include a heterogeneous group of clonal hematological stem cell disorders characterized by ineffective hematopoiesis, cytopenias. MicroRNAs (miRNAs) are short non-coding RNA molecules that repress gene expression at the post-transcriptional level. In this review, we summarize advanced investigations that underscore deregulated miRNA expression in MDS, and discuss the implications of miRNAs in the molecular pathogenesis of MDS.
Methods: Relevant English-language literatures were searched and retrieved from PubMed using the terms MDS and miRNAs.
Results: The majority of studies have focused on profiling miRNA expression in MDS, only a small number of studies have investigated the exact pathogenic role of miRNAs in MDS.
Discussion: In the hematopoietic system, miRNAs are critical regulators of the differentiation of hematopoietic stem/progenitor cells. Thus, it is not surprising that dysregulation of miRNAs can lead to hematopoietic stem cell anomalies and further cause MDS. Deregulated miRNA expression has been identified in MDS, and it contributes to the pathogenesis and progression of MDS. Chromosomal aberrations, hypermethylation of miRNA promoters, and mutations of miRNA genes may lead to dysregulation of miRNA in MDS. However, the complex regulatory networks between miRNAs and their potential target genes in MDS still need to be explored in further studies.
Conclusions: Although the function of miRNAs is not fully understood, these small non-coding RNAs represent novel pathogenetic and clinical implications in MDS. The studies of miRNAs may guide us towards a better understanding of this disease and shed light on the development of new therapeutic strategies.
Introduction
Myelodysplastic syndromes (MDS) are a group of clonal diseases originating from hematopoietic stem cells (HSCs). Patients with MDS usually present with anemia and other cytopenia, and about a third of patients will develop into acute myeloid leukemia (AML).Citation1 There are seven major subtypes of MDS according to the 2008 WHO classification: refractory cytopenia with unilineage dysplasia (RCUD) (refractory anemia (RA); refractory neutropenia (RN); refractory thrombocytopenia (RT)), RA with ringed sideroblasts (RARS), RA with multilineage dysplasia (RCMD), RA with excess blasts-1 (RAEB-1), RA with excess blasts-2 (RAEB-2), MDS-unclassified (MDS-U), and MDS with isolated deletion chromosome 5q (del(5q)) (5q- syndrome).Citation2 Current known risk factors of MDS include ageing, chemotherapy or radiation treatment, occupational exposure and some genetic syndromes such as Fanconi's anemia.Citation1,Citation3 Various risk factors lead to somatic gene mutations, epigenetic deregulation, microenvironmental and immune changes, all of which result in defects of HSCs and further cause MDS.Citation1 However, to date, the precise mechanisms behind the initiation and evolution of MDS are still not fully understood, and current effective treatment and diagnosis methods of MDS are limited because of the heterogeneity of this disease.
MicroRNAs (miRNAs) are short non-coding RNA molecules that repress expression of genes by inhibiting the transcription or inducing degradation of target mRNA.Citation4 To date, there are about 2603 mature miRNAs in humans (miRBase 21, June 2014), each of them with the potential to regulate hundreds of target genes that encode for proteins.Citation5 By inhibiting translation of target genes, miRNAs regulate numerous biological processes in cells such as proliferation, apoptosis, and differentiation.Citation6 In addition, miRNAs can be either oncogenes or tumor suppressors in the pathogenesis of different cancers,Citation7 which may be due to the fact that more than 50% of miRNA genes are located with cancer-related regions.Citation8 A recent genomic analysis of AML and MDS cell lines found that about 77% (542 out of 706) of miRNAs are located in regions of leukemia-associated cytogenetic changes, and 18% (99 out of 542) of these miRNAs are relevant in myeloid malignancies.Citation9 Moreover, miRNAs are essential regulators of the differentiation and development of HSCs.Citation10Citation11Citation12–Citation13 Therefore, alterations of these non-coding RNA molecules may affect proliferation and differentiation of HSCs, contributing to the pathogenesis of hematological malignancies, including MDS.Citation14,Citation15 In this paper, we review dysregulation of miRNAs in MDS and highlight its pathogenetic role in MDS. In addition, the clinical implications of these small RNAs are discussed.
Profilings of miRNA in MDS
Profilings of miRNAs provide clues to the pathogenesis of MDS
The miRNA profiles of various hematological malignancies have been determined,Citation16Citation17Citation18–Citation19 and have been shown to differ.Citation20 MDS is regarded as a preleukemia disease and a third of MDS patients will develop into AML ultimately.Citation1 Differential miRNA expression profiles have been identified between early and advanced stages of MDS, which implies that these miRNAs may be involved in the pathogenesis of MDS and the transformation of MDS to AML. In one study, expression levels of miR-422a and miR-617 were found to be associated with disease progression (their levels were progressively increased from healthy people to early MDS to advanced MDS and secondary AML).Citation21 Similarly, lower expression levels of miR-181a and miR-222 were observed in early-stage MDS in contrast with later-stage MDS.Citation22 In agreement with this, another study showed that the expression of four miR-181 family members was highly increased in patients with advanced MDS compared to patients with early MDS.Citation23 Importantly, these miRNAs have been proven to be important regulators in hematopoiesis. MiR-181 regulates mouse lymphopoiesis in the early stages,Citation24 whereas it inhibits granulocytic and macrophage-like differentiation in human HSCs.Citation25 MiR-222 blocks erythropoiesis via translational repression of c-KIT.Citation26 In contrast to the miRNAs mentioned above, mir-17-5p and mir-20a were shown to be upregulated in low-risk MDS rather than in high-risk MDS.Citation27 A common potential target of these two miRNAs is E2F1, which is an important regulator in cell proliferation and apoptosis.Citation28 Similarly, miR-34a was consistently overexpressed in early MDS,Citation21 and it induced apoptosis via partly targeting Bcl-2,Citation29 indicating miR-34a may contribute to the increased apoptotic status in early MDS. Overall, all these findings form the basis for further investigations of leukemic transformation in MDS. However, the exact role of these miRNAs in the transformation of MDS to AML still needs to be proven in further in vitro and in vivo experiments.
MiRNA profiling in MDS samples from different sources
Many studies have identified differentially expressed miRNAs in MDS by using high-throughput methods, and further validated the expression of these miRNAs with the use of quantitative reverse-transcriptase PCR (qRT-PCR). In these studies, the most widely used sample is unsorted mononuclear cells (MNCs). By using miRNA microarray and RT-PCR assay, the expression of eight miRNAs was identified to be significantly deregulated in bone marrow (BM)-MNCs of MDS patients (Table ).Citation30 In a whole-genome miRNA profiling study, Borze et al.Citation31 found that levels of miR-720 and miR-21 were increased, whereas miR-671-5p and one human virus miRNA (Epstein–Barr virus miR-BART13) were downregulated in MDS. BM and peripheral blood (PB) are both important samples in the diagnosis of patients with MDS. Pons et al.Citation22 profiled miRNAs expression in both BM and PB MNCs from 25 patients with MDS, and found different miRNA expression profiles in these two different samples (Table ), suggesting that the expression of miRNAs in MDS is tissue specific.
Table 1 MiRNA expression profiling in MDS
Only in three studies were CD34+ HSCs chosen as sample sources. Because MDS is a clonal disorder arising in HSCs,Citation1 these studies may lay the foundations for further investigations to explore the pathogenetic implication of miRNAs in MDS. In one of the studies that selected CD34+ cells as sample source, expression levels of mir-17-5p, mir-20a and let-7a in MDS patients were not distinctly different from those in healthy controls. However, increased miR-17-5p and miR-20a expression is linked with favorable outcome in MDS patients.Citation27 Dostalova Merkerova et al.Citation21 analyzed miRNAs expression profiling in CD34+ cells from 43 MDS patients and nine healthy controls, and found 22 miRNAs differentially expressed in MDS (Table ). The expression of four miRNAs encoded on 5q was analyzed in CD34+ HSCs from seven 5q- syndrome patients and five normal controls. The results showed that the expression of miR-378 and miR-146a was significantly reduced, whereas miR-143 and miR-145 expression levels of 5q- syndrome patients were not obviously different from those of healthy controls.Citation33 However, in PB CD14+ monocytes of 11 patients with del(5q), only miR-378 showed decreased expression.Citation34 This inconsistency may be attributed to the different samples used, the former study used CD34+ cells, the latter used PB CD14+ monocytes.
Mesenchymal stromal cells (MSCs) are a group of small, non-hematopoietic cells residing in the BM. Regarded as the osteoblastic progenitors, they are also key components in the hematopoietic microenvironment.Citation35 Santamaria et al.Citation36 profiled the miRNA expression of MSCs from 21 low-risk MDS with the use of low-density arrays, and found 159 miRNAs that were significantly downregulated in MDS when compared with controls. Plasma is a widely used clinical sample because of its accessibility. Recently, Zuo et al.Citation32 applied a high-throughput assay to detect the specific miRNA profile in plasma that could distinguish patients with MDS from healthy controls (Table ).
Overall, it is difficult to conclude which sample source is the most suitable for the determination of miRNA profiles in MDS. MNC and plasma can be more conveniently used to identify miRNAs that can serve as biomarkes in MDS, whereas HSC is a more suitable sample to decipher the contribution of candidate miRNAs to the molecular pathogenesis of MDS. Taken together, the differences in patient numbers, sample sources, and discovery platforms, may justify the discrepancies among the different studies. It is hoped, however, that further investigations of miRNA expression profiles in MDS may provide new tools for diagnosis and reinforce the theoretical basis for further functional studies of miRNAs.
The pathogenetic role of miRNAs in MDS
With the wide use of molecular biology techniques, a variety of genetic lesions have been found to be implicated in the pathological processes of MDS. The known genetic lesions leading to MDS include amplifications or deletions of genes, functional genetic mutations, and epigenetic deregulation.Citation37 In addition, microenvironmental changes and immune dysregulation also contribute to the development of MDS.Citation1,Citation3 Nevertheless, the exact molecular mechanisms leading to MDS pathogenesis and disease progression still remain to be fully elucidated. Recent studies have attempted to reveal the pathogenetic roles of miRNAs in MDS, which may improve our understanding of this complex disease.
miRNAs associated with the phenotypes of 5q-syndrome
Early in 2002, a commonly deleted region (CDR) in the long arm of chromosome 5 was identified. The CDR contains 40 protein-coding genesCitation38 and three miRNA genes,Citation39 and previous studies have reported that the pathophysiology of 5q- syndrome was linked with haploinsufficiency of protein-coding genes residing in CDR. For example, haploinsufficiency of RPS14 can cause macrocytic anemia, by interfering with differentiation and proliferation of the erythroid lineage via the P53 pathway.Citation40,Citation41 However, haploinsufficiency of protein-coding genes does not explain all clinical phenotypes of the 5q- syndrome. Recent studies have shown that haploinsufficiency of miRNA genes located in CDR also participates in the pathological processes of 5q- syndrome (Fig. ).
Figure 1 Schematic diagram of the major molecular pathogenesis of 5q- syndrome. Haploinsufficiency of RPS14 mainly causes erythroid defect, and haploinsufficiency of miR-145 and miR-146a is related to megakaryocytic dysplasia. Coordinate loss of miR-145 and RPS14 caused a significant increase in the ratio of megakaryocytic to erythroid cells. Taken together, the haploinsufficiency of miRNAs genes and protein-coding genes located in the commonly deleted region cooperate in the pathogenesis of 5q- syndrome.
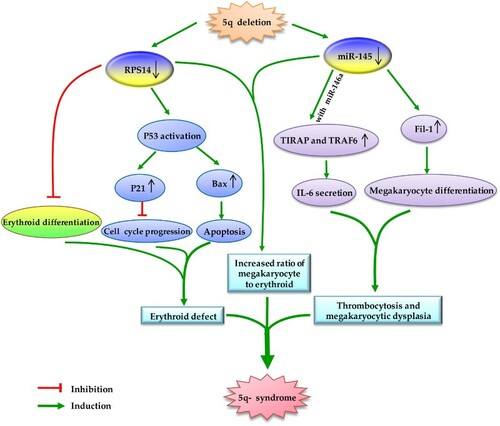
Haploinsufficiency of miR-143, miR-145 (encoded on CDR) and miR-146a (adjacent to the CDR) seems to alter hematopoiesis in a way similar to 5q-syndrome. Mice with decreased levels of miR-145 and miR-146a displayed hematopoietic disorders resembling 5q- syndrome, including hypolobated megakaryocytes and peripheral thrombocytosis. Researchers further found that haploinsufficiency of miR-145 and miR-146a increased megakaryopoiesis via upregulation of TIRAP and TRAF6 (which are regulated by miR-145 and miR-146a, respectively).Citation42 In agreement with this, Kumar et al.Citation43 found that decreased expression of miR-145 resulted in an increase in megakaryocytes by targeting Fli-1, a transcription factor playing an important role in megakaryopoiesis.Citation44 Moreover, combination of miR-145 and RPS14 deletion seemed to cause a greater degree of increase in megakaryocytes than miR-145 deletion alone.Citation43 Interestingly, miR-145 could increase erythroid colony formation in BM progenitors from miR-145-overexpressing recipient mice, whereas it had no effect on erythroid colony formation in human CD34+ cells in vitro.Citation43 These findings imply that the effect of miR-145 on erythropoiesis may differ between mouse and human, or miR-145 may only influence erythropoiesis in vivo. Increased activity of erythroid progenitors is also observed in cord blood CD34+ cells with dysregulated miR-143.Citation45 However, there is no study exploring the role of miR-378 (which is also encoded on CDR) in the initiation and progression of 5q- syndrome. Higher levels of miR-378 were associated with intermediate stages of erythropoiesis,Citation46 and the erythropoietin receptor is a putative target of miR-378,Citation47 suggesting that miR-378 may be related to erythropoiesis. However, in vitro and in vivo evidences needed to decipher the functional role of miR-378 in 5q- syndrome.
MiRNAs act as oncogenes in the pathogenesis and progression of MDS
A few miRNAs have been proven to function as oncogenes in the pathogenesis and progression of MDS. These miRNAs are upregulated and contribute to the pathogenesis and progression of MDS by repressing the expression of tumor suppressors. Lee et al.Citation48 demonstrated that increased expression of miR-210 may participate in the disease progression of MDS by targeting tumor suppressor SHIP-1, loss of which resulted in myeloproliferative diseases in mice.Citation49,Citation50 MiR-10a and miR-10b are known to be upregulated in MDS patients.Citation22,Citation33,Citation51 In addition, expression of miR-10a/b is under the control of transcription factor TWIST-1, and knockdown of miR-10a/b enhances apoptosis in leukemic cells via the p53/NF-κB pathway.Citation51 MiR-22 is upregulated in both adult and pediatric MDS patients,Citation52,Citation53 and its overexpression is inversely correlated with tumor suppressor TET2 expression.Citation52 By using a luciferase reporter assay, Song et al.Citation53 identified that miR-22 directly targeted TET2. In addition, miR-22 transgenic mice displayed increased proliferation of HSCs and impaired differentiation and ultimately developed into MDS and hematological malignancies.Citation53 In another study, overexpression of miR-21 led to obvious reduction in erythroid colony formation in primary CD34+ cells via decreasing SMAD7 expression. Additionally, inhibition of miR-21 stimulated ertythropoiesis in both mice with dyshematopoiesis and primary MDS cells,Citation54 strengthening the pathogenetic role of miR-21 in MDS. Of note, some researchers of the aforementioned studies chose primary AML cells or AML cell lines to explore the roles of miRNAs in the progression of MDS. It is known that culture and transfection of primary MDS cells are still technically challenging, and there are few cell lines resembling human MDS.Citation55 However, it would be preferable to use primary MDS cells or AML cell lines transformed from MDS in these studies.
The specific role of miR-125 family in the pathogenesis of dyshematopoiesis and MDS
Overexpression of miR-125b has been observed in MDS and AML,Citation56,Citation57 and it is able to block differentiation of primary CD34+ cellsCitation58 and promote hematopoietic engraftment of HSCs.Citation59,Citation60 In addition, overexpression of miR-125b leads to leukemia in several mouse models. By upregulating miR-125b expression in murine fetal liver cells, various leukemia-like diseases were generated.Citation61In one in vivo experiment, overexpression of miR-125b caused a myeloproliferative disorder that further developed into myeloid leukemia.Citation59 The development of a highly invasive myeloid leukemia was also observed in another overexpressing-miR-125b mouse model.Citation62 Moreover, upregulation of miR-125b expression is likely to transform myeloid cells by repressing a number of mRNAs, which are critical regulators of myeloid differentiation and apoptosis, such as CBFB and multiple genes involved in the P53 pathway.Citation63
Similar to miR-125b, overexpression of miR-125a can also promote hematopoietic engraftment of HSCs.Citation59,Citation64 A recent paper reported that miR-125a also blocked erythroid differentiation in both MDS and erythroleukemia cell lines.Citation56 As described previously, overexpression of miR-125b is likely to cause leukemia, whereas miR-125a is more likely to cause myeloproliferative diseases in mouse models. Several studies have described that upregulation of miR-125a in mice caused disorders similar to myeloproliferative neoplasm.Citation64,Citation65
Interestingly, these two miRNAs are identified only in enriched HSCs instead of in total BM cells,Citation59 and they are both downregulated upon differentiation.Citation64 Although these two miRNAs are encoded on different chromosomes, they have identical seed sequences and similar effects on HSCs, which indicates that some target genes of these two miRNAs may overlap.
Hypermethylation of miRNA promoter in MDS
As described before, methylation of miRNAs can cause downregulation of some miRNAs in MDS. More importantly, hypermethylation of miRNA promoters is likely to contribute to the pathogenesis and progression of MDS. Hypermethylation of the miR-34b promoter is found in patients with MDS-post AML, and these patients are likely to have acquired the miR-34b hypermethylation status during the progression to AML. In addition, downregulation of miR-34b caused by hypermethylation of the promoter enhances cell proliferation and clonogenic potential in healthy BM and fetal liver cells,Citation66 further suggesting the potential role of miR-34b hypermethylation in the transformation of MDS to AML. In one study, mice transplanted with BM cells overexpressing EVI1 suffered from a MDS-like disease characterized by defective erythropoiesis and megakaryopoiesis, pancytopenia, and severe anemia.Citation67 More importantly, inappropriate EVI1 expression causes methylation and silencing of miR-124, which contributes to deregulation of cell cycle and self-renewal in murine BM cells, ultimately leading to BM failure in mice.Citation68 All these findings unify dysregulation of miRNAs and epigenetic regulation in MDS into a common pathway.
Potential mechanisms causing dysregulation of miRNAs in MDS
Chromosomal abnormalities are present in 20–70% patients with variants of MDS,Citation69 and the most common chromosomal aberrations include del(5q), monosomy 7 (−7) or del(7q), trisomy 8 (+8), and del(20q).Citation3 By using high resolution genome arrays, Starczynowski et al.Citation9 found that many (∼70%) miRNAs mapped to leukemia-associated copy-number alterations, suggesting chromosomal aberrations may be a major contributor to the alterations in miRNA expression. Recent studies have proved that abnormal karyotype can affect miRNA expression patterns in MDS. Twenty miRNAs are downregulated in MDS with +8 compared with cytogenetically normal MDS (Table ).Citation70 MDS with del(5q) also show a unique miRNA expression pattern compared to healthy controls (Table ).Citation33 The plasma levels of 13 miRNAs in MDS with del(20q) were found to be significantly different from those in MDS with a normal karyotype. MiRNA expression profile of MDS patients with −7 is also different from that of patients with cytogenetically normal MDS (Table ).Citation32 Interestingly, aberration of these karyotypes do not distinctly affect the expression of miRNAs located in the affected chromosomal region, which indicates that the mechanisms behind the regulation of miRNAs are complex. Out of four miRNAs that form a cluster on del(5q), expression levels of miR-378 and miR-146a are significantly reduced in BM CD34+ cells from 5q- patients, whereas miR-143 and miR-145 are identified to have almost normal expression levels.Citation33 Thirteen miRNAs encoded on chromosome 7 were not downregulated in RAEB-2 with -7 compared with RAEB-2 with normal karyotype.Citation70 Similarly, plasma levels of two miRNAs (miR-296 and miR-499) that are located on 20q are significantly increased in MDS patients with del (20q).Citation32
Table 2 Upregulated and downregulated miRNAs related with major chromosomal alterations in MDS
Similar to protein-coding genes, expression of miRNA genes can be post-transcriptionally regulated by various factors.Citation71,Citation72 Therefore, transcriptional or post-transcriptional regulators may also alter the expression of miRNAs in MDS. For example, transcription factor TWIST-1 can induce expression of miR-10a/b by binding directly to the promoters of miR-10a/b,Citation73 and elevated levels of miR-10a/b correlate with increased TWIST-1 mRNA level in MDS.Citation51 Another example is miR-34a, which is regulated by the transcription factor encoded by the tumor suppressor gene P53,Citation74 and expression of miR-34a is increased in early-MDS patients.Citation21
Emerging investigations indicate that hypermethylation of miRNA promoters is one of the mechanisms causing downregulation of miRNAs.Citation75,Citation76 Hypermethylation status of a few intragenic miRNAs and their host genes (miR-140/WWP2, miR-378/PPARGC1B and miR-632/ZNF207) was identified, which is consistent with decreased expression of these miRNAs and their host genes in MDS.Citation76 MiR-124 is downregulated in MDS patients, and its downregulation correlates with promoter methylation.Citation77
Acquisition of somatic mutations of miRNA genes is another mechanism by which miRNA function may be altered in MDS. Recently, heterozygous mutations in miR-142, miR-632 were detected in MDS. Of 519 patients with primary MDS or secondary AML, four patients carried miR-142 mutations (0.77%) and one carried miR-632 mutations (0.19%). Moreover, all mutations of miR-142 occurred in the seed region,Citation78 which indicates that these mutations may influence the function of miR-142.
Clinical implications of miRNAs in MDS
The International Prognostic Scoring System (IPSS) relies on the cytogenetics, morphology, and clinical features to group MDS patients into four prognostic groups: low risk, intermediate-1 risk, intermediate-2 risk, and high risk.Citation79 However, the present scoring systems of MDS, including the IPSS, are limited to predicting outcomes of the MDS patient, and do not fully reflect the genetic complexity of this disease.Citation79,Citation80 More molecular signatures are needed to improve prediction accuracy and help for stratification of MDS patients. There are many miRNAs that have potential to be prognostic biomarkers for stratification in MDS, which may also constitute targets for novel clinical approaches of this disease in future.
In 22 patients with MDS, median survival time was longer in patients with low levels of miR-181 family (9.3 years) than in patients with high levels of these miRNAs (3.5 years).Citation23 Zuo et al.Citation81 identified that elevated let-7a in MDS patients was correlated with shorter overall survival (OS) in different IPSS groups (P < 0.001). Median survival time in MDS patients with high level of miR-194-5p was longer than patients with low level of miR-194-5p (72 months vs. 19 months, P = 0.049).Citation82 Similarly, MDS patients with higher-expressing miR-20a and miR-17-5p are at lower risk of death, and OS is better in these patients.Citation27 The median OS and progression free survival of MDS patients with low miR-21 levels compared favorably with those of patients with high miR-21 levels (45.0 vs. 22.6 months and 44.5 vs. 14.0 months, respectively).Citation83 In a retrospective study, Song et al.Citation53 found that upregulated miR-22 indicated poor survival in MDS patients. Recently, a signature of seven miRNAs (let-7a, miR-144, miR-16, miR-25, miR-451, miR-651, miR-655) was validated as an independent predictor of MDS survival with 75% accuracy (P = 0.008) in MDS patients with normal cytogenetics,Citation32 implying that different individual miRNAs could be combined to improve the predictive power of miRNA biomarkers.
Taken together, although these studies try to normalize patients in each study cohort, there are still various confounding factors such as age, blood cell counts, complications, and molecular genetics in these patients. Certainly, the predictive value of these miRNAs still needs to be evaluated in more prospective studies including larger MDS patient cohorts.
In addition to the prognostic implications of miRNAs, an increasing number of studies associate miRNAs with the response to therapy in MDS. A recent study has demonstrated miR-21 as a predictor of response to hypomethylating agentsin MDS. Patients with low miR-21 levels had a higher overall response rate than those with high miR-21 levels (73.2 vs. 41.2%, P = 0.021).Citation83 Treatment with lenalidomide led to upregulation of miR-143 (1.8-fold) and miR-145 (1.9-fold) in CD34+ cells obtained from 29 MDS patients (10 del(5q) and 19 non-del(5q)). The researchers further found that transfusion independence was associated with high miR-145 level in patients with del(5q).Citation45 Another study identified that the miRNAs mapped to 14q32 were differentially expressed in lenalidomide-treated del(5q) patients.Citation84 Thus, these miRNAs may have potential to be used as biomarkers for predicting therapeutic outcomes in MDS patients.
Conclusions and prospects
Although the function of miRNAs is not fully understood, these small non-coding RNAs represent novel pathogenetic implications in MDS. Recent advances have strongly suggested that miRNAs are critical in the pathogenesis of MDS, and that dysregulation of miRNAs and other molecular defects cooperate to cause MDS. In addition, bioinformatic algorithms (miRBase 21, TargetScan 7.0) indicate that one miRNA can have multiple potential target genes, suggesting that one miRNA may target various genes and cause disease by interfering with various signaling pathways. However, the complex regulatory networks between miRNAs and their potential target genes in MDS still need to be explored in further studies. Currently, the outcome of patients with MDS is poor and effective treatment options are limited. Therefore, the possible use of miRNA-based prognostic biomarkers may be an important clinical approach in this disease. It is foreseeable that in the future we can combine different miRNAs to predict outcomes of patients with MDS. Similarly, the combination of miRNA markers with other molecular signatures may also help to better predict outcomes of individual patients.Citation85 Recently, Bhagat et al.Citation54 found that a miR-21 inhibitor could improve anemia of MDS both in vitro and in vivo, suggesting miRNA-targeted therapy for MDS is possible in the near future.
Disclaimer statement
Contributors L.W., and J.C. conceived the manuscript and revised it; X.K. wrote the manuscript and prepared the figure and tables.
Funding This work was supported by the National Natural Science Foundation of China (No. 30971277; No. 81250034), the Natural Science Foundation of Chongqing (CSTC, 2009BB5070), the Health Bureau of Chongqing (2013-2-023), The Foundation of Chongqing Graduate Student Innovative Research (CYS14123) and the Project Foundation of Chongqing Municipal Education Committee (2013).
Conflict of interest The authors have no conflict of interest to disclose.
Ethics approval None.
References
- Adès L, Itzykson R, Fenaux P. Myelodysplastic syndromes. Lancet 2014;383(9936):2239–52. doi: 10.1016/S0140-6736(13)61901-7
- Vardiman JW, Thiele J, Arber DA, Brunning RD, Borowitz MJ, Porwit A, et al. The 2008 revision of the World Health Organization (WHO) classification of myeloid neoplasms and acute leukemia: rationale and important changes. Blood 2009;114(5):937–51. doi: 10.1182/blood-2009-03-209262
- Tefferi A, Vardiman JW. Myelodysplastic syndromes. N Engl J Med. 2009;361(19):1872–85. doi: 10.1056/NEJMra0902908
- Ambros V. microRNAs: tiny regulators with great potential. Cell 2001;107(7):823–26. doi: 10.1016/S0092-8674(01)00616-X
- Lewis BP, Burge CB, Bartel DP. Conserved seed pairing, often flanked by adenosines, indicates that thousands of human genes are microRNA targets. Cell 2005;120(1):15–20. doi: 10.1016/j.cell.2004.12.035
- Kloosterman WP, Plasterk RHA. The diverse functions of microRNAs in animal development and disease. Dev Cell 2006;11(4):441–50. doi: 10.1016/j.devcel.2006.09.009
- Zhang B, Pan X, Cobb GP, Anderson TA. microRNAs as oncogenes and tumor suppressors. Dev Biol. 2007;302(1):1–12. doi: 10.1016/j.ydbio.2006.08.028
- Calin GA, Sevignani C, Dumitru CD, Hyslop T, Noch E, Yendamuri S, et al. Human microRNA genes are frequently located at fragile sites and genomic regions involved in cancers. Proc Natl Acad Sci. 2004;101(9):2999–3004. doi: 10.1073/pnas.0307323101
- Starczynowski DT, Morin R, McPherson A, Lam J, Chari R, Wegrzyn J, et al. Genome-wide identification of human microRNAs located in leukemia-associated genomic alterations. Blood 2011;117(2):595–607. doi: 10.1182/blood-2010-03-277012
- Johnnidis JB, Harris MH, Wheeler RT, Stehling-Sun S, Lam MH, Kirak O, et al. Regulation of progenitor cell proliferation and granulocyte function by microRNA-223. Nature 2008;451(7182):1125–29. doi: 10.1038/nature06607
- Liao R, Sun J, Zhang L, Lou G, Chen M, Zhou D, et al. MicroRNAs play a role in the development of human hematopoietic stem cells. J Cell Biochem. 2008;104(3):805–17. doi: 10.1002/jcb.21668
- Fallah P, Arefian E, Naderi M, Aghaee-Bakhtiari SH, Atashi A, Ahmadi K, et al. miR-146a and miR-150 promote the differentiation of CD133+ cells into T-lymphoid lineage. Mol Biol Rep. 2013;40(8):4713–19. doi: 10.1007/s11033-013-2567-6
- Georgantas RW, Hildreth R, Morisot S, Alder J, Liu C-G, Heimfeld S, et al. CD34+ hematopoietic stem-progenitor cell microRNA expression and function: a circuit diagram of differentiation control. Proc Natl Acad Sci. 2007;104(8):2750–55. doi: 10.1073/pnas.0610983104
- Alemdehy MF, Erkeland SJ. MicroRNAs: key players of normal and malignant myelopoiesis. Curr Opin Hematol. 2012;19(4):261–67. doi: 10.1097/MOH.0b013e328353d4e9
- Lujambio A, Lowe SW. The microcosmos of cancer. Nature 2012;482(7385):347–55. doi: 10.1038/nature10888
- Navarro A, Gaya A, Martinez A, Urbano-Ispizua A, Pons A, Balagué O, et al. MicroRNA expression profiling in classic Hodgkin lymphoma. Blood 2008;111(5):2825–32. doi: 10.1182/blood-2007-06-096784
- Marcucci G, Radmacher MD, Maharry K, Mrózek K, Ruppert AS, Paschka P, et al. MicroRNA expression in cytogenetically normal acute myeloid leukemia. N Engl J Med. 2008;358(18):1919–28. doi: 10.1056/NEJMoa074256
- Rokah OH, Granot G, Ovcharenko A, Modai S, Pasmanik-Chor M, Toren A, et al. Downregulation of miR-31, miR-155, and miR-564 in chronic myeloid leukemia cells. PLoS ONE 2012;7(4):e35501. doi: 10.1371/journal.pone.0035501
- Wang J, Xiang G, Zhang K, Zhou Y. Expression signatures of intragenic miRNAs and their corresponding host genes in myeloid leukemia cells. Biotechnol Lett. 2012;34(11):2007–15. doi: 10.1007/s10529-012-1018-0
- Mi S, Lu J, Sun M, Li Z, Zhang H, Neilly MB, et al. MicroRNA expression signatures accurately discriminate acute lymphoblastic leukemia from acute myeloid leukemia. Proc Natl Acad Sci. 2007;104(50):19971–76. doi: 10.1073/pnas.0709313104
- Dostalova Merkerova M, Krejcik Z, Votavova H, Belickova M, Vasikova A, Cermak J. Distinctive microRNA expression profiles in CD34+ bone marrow cells from patients with myelodysplastic syndrome. Eur J Hum Genet. 2011;19(3):313–9. doi: 10.1038/ejhg.2010.209
- Pons A, Nomdedeu B, Navarro A, Gaya A, Gel B, Diaz T, et al. Hematopoiesis-related microRNA expression in myelodysplastic syndromes. Leukemia Lymphoma 2009;50(11):1854–9. doi: 10.3109/10428190903147645
- Sokol L, Caceres G, Volinia S, Alder H, Nuovo GJ, Liu CG, et al. Identification of a risk dependent microRNA expression signature in myelodysplastic syndromes. Br J Haematol. 2011;153(1):24–32. doi: 10.1111/j.1365-2141.2011.08581.x
- Chen CZ, Li L, Lodish HF, Bartel DP. MicroRNAs modulate hematopoietic lineage differentiation. Science 2004;303(5654):83–6. doi: 10.1126/science.1091903
- Su R, Lin HS, Zhang XH, Yin XL, Ning HM, Liu B, et al. MiR-181 family: regulators of myeloid differentiation and acute myeloid leukemia as well as potential therapeutic targets. Oncogene 2015;34(25):3226–39. doi: 10.1038/onc.2014.274
- Felli N, Fontana L, Pelosi E, Botta R, Bonci D, Facchiano F, et al. MicroRNAs 221 and 222 inhibit normal erythropoiesis and erythroleukemic cell growth via kit receptor down-modulation. Proc Natl Acad Sci. 2005;102(50):18081–6. doi: 10.1073/pnas.0506216102
- Vasilatou D, Papageorgiou SG, Kontsioti F, Kontos CK, Tsiotra P, Mpakou V, et al. Expression analysis of mir-17-5p, mir-20a and let-7a microRNAs and their target proteins in CD34+ bone marrow cells of patients with myelodysplastic syndromes. Leukemia Res. 2013;37(3):251–8. doi: 10.1016/j.leukres.2012.11.011
- Mundle SD, Saberwal G. Evolving intricacies and implications of E2F1 regulation. FASEB J. 2003;17(6):569–74. doi: 10.1096/fj.02-0431rev
- Wang X, Liu P, Zhu H, Xu Y, Ma C, Dai X, et al. miR-34a, a microRNA up-regulated in a double transgenic mouse model of Alzheimer's disease, inhibits bcl2 translation. Brain Res Bull. 2009;80(4–5):268–73. doi: 10.1016/j.brainresbull.2009.08.006
- Erdogan B, Facey C, Qualtieri J, Tedesco J, Rinker E, Isett RB, et al. Diagnostic microRNAs in myelodysplastic syndrome. Exp Hematol. 2011;39(9):915–26. doi: 10.1016/j.exphem.2011.06.002
- Borze I, Scheinin I, Siitonen S, Elonen E, Juvonen E, Knuutila S. miRNA expression profiles in myelodysplastic syndromes reveal Epstein–Barr virus miR-BART13 dysregulation. Leukemia Lymphoma 2011;52(8):1567–73. doi: 10.3109/10428194.2011.568652
- Zuo Z, Maiti S, Hu S, Loghavi S, Calin GA, Garcia-Manero G, et al. Plasma circulating-microRNA profiles are useful for assessing prognosis in patients with cytogenetically normal myelodysplastic syndromes. Modern Pathol. 2015;28(3):373–82. doi: 10.1038/modpathol.2014.108
- Votavova H, Grmanova M, Dostalova Merkerova M, Belickova M, Vasikova A, Neuwirtova R, et al. Differential expression of microRNAs in CD34+ cells of 5q-syndrome. J Hematol Oncol. 2011;4:1. doi: 10.1186/1756-8722-4-1
- Merkerova MD, Krejcik Z, Belickova M, Hrustincova A, Klema J, Stara E, et al. Genome-wide miRNA profiling in myelodysplastic syndrome with del(5q) treated with lenalidomide. Eur J Haematol. 2015;95(1):35–43. doi: 10.1111/ejh.12458
- Flores-Figueroa E, Montesinos JJ, Flores-Guzmán P, Gutiérrez-Espíndola G, Arana-Trejo RM, Castillo-Medina S, et al. Functional analysis of myelodysplastic syndromes-derived mesenchymal stem cells. Leukemia Res. 2008;32(9):1407–16. doi: 10.1016/j.leukres.2008.02.013
- Santamaria C, Muntion S, Roson B, Blanco B, Lopez-Villar O, Carrancio S, et al. Impaired expression of DICER, DROSHA, SBDS and some microRNAs in mesenchymal stromal cells from myelodysplastic syndrome patients. Haematologica 2012;97(8):1218–24. doi: 10.3324/haematol.2011.054437
- Bejar R, Levine R, Ebert BL. Unraveling the Molecular Pathophysiology of Myelodysplastic Syndromes. J Clin Oncol 2011;29(5):504–15. doi: 10.1200/JCO.2010.31.1175
- Boultwood J, Fidler C, Strickson AJ, Watkins F, Gama S, Kearney L, et al. Narrowing and genomic annotation of the commonly deleted region of the 5q- syndrome. Blood 2002;99(12):4638–41. doi: 10.1182/blood.V99.12.4638
- Jadersten M, Karsan A. Clonal evolution in myelodysplastic syndromes with isolated del(5q): the importance of genetic monitoring. Haematologica 2011;96(2):177–80. doi: 10.3324/haematol.2010.038281
- Ebert BL, Pretz J, Bosco J, Chang CY, Tamayo P, Galili N, et al. Identification of RPS14 as a 5q-syndrome gene by RNA interference screen. Nature 2008;451(7176):335–39. doi: 10.1038/nature06494
- Barlow JL, Drynan LF, Hewett DR, Holmes LR, Lorenzo-Abalde S, Lane AL, et al. A p53-dependent mechanism underlies macrocytic anemia in a mouse model of human 5q-syndrome. Nature Med. 2010;16(1):59–66. doi: 10.1038/nm.2063
- Starczynowski DT, Kuchenbauer F, Argiropoulos B, Sung S, Morin R, Muranyi A, et al. Identification of miR-145 and miR-146a as mediators of the 5q-syndrome phenotype. Nature Med. 2010;16(1):49–58. doi: 10.1038/nm.2054
- Kumar MS, Narla A, Nonami A, Mullally A, Dimitrova N, Ball B, et al. Coordinate loss of a microRNA and protein-coding gene cooperate in the pathogenesis of 5q- syndrome. Blood 2011;118(17):4666–73. doi: 10.1182/blood-2010-12-324715
- Neuwirtova R, Fuchs O, Holicka M, Vostry M, Kostecka A, Hajkova H, et al. Transcription factors Fli1 and EKLF in the differentiation of megakaryocytic and erythroid progenitor in 5q-syndrome and in Diamond-Blackfan anemia. Ann Hematol. 2013;92(1):11–8. doi: 10.1007/s00277-012-1568-1
- Venner CP, Woltosz JW, Nevill TJ, Deeg HJ, Caceres G, Platzbecker U, et al. Correlation of clinical response and response duration with miR-145 induction by lenalidomide in CD34+ cells from patients with del(5q) myelodysplastic syndrome. Haematologica 2013;98(3):409–13. doi: 10.3324/haematol.2012.066068
- Bruchova H, Yoon D, Agarwal AM, Mendell J, Prchal JT. Regulated expression of microRNAs in normal and polycythemia vera erythropoiesis. Exp Hematol. 2007;35(11):1657–67. doi: 10.1016/j.exphem.2007.08.021
- Qian J, Lin J, Qian W, Ma JC, Qian SX, Li Y, et al. Overexpression of miR-378 is frequent and may affect treatment outcomes in patients with acute myeloid leukemia. Leukemia Res. 2013;37(7):765–8. doi: 10.1016/j.leukres.2013.03.014
- Lee DW, Futami M, Carroll M, Feng Y, Wang Z, Fernandez M, et al. Loss of SHIP-1 protein expression in high-risk myelodysplastic syndromes is associated with miR-210 and miR-155. Oncogene 2012;31(37):4085–94. doi: 10.1038/onc.2011.579
- Harder KW, Quilici C, Naik E, Inglese M, Kountouri N, Turner A, et al. Perturbed myelo/erythropoiesis in Lyn-deficient mice is similar to that in mice lacking the inhibitory phosphatases SHP-1 and SHIP-1. Blood 2004;104(13):3901–10. doi: 10.1182/blood-2003-12-4396
- Lakhanpal GK, Vecchiarelli-Federico LM, Li YJ, Cui JW, Bailey ML, Spaner DE, et al. The inositol phosphatase SHIP-1 is negatively regulated by Fli-1 and its loss accelerates leukemogenesis. Blood 2010;116(3):428–36. doi: 10.1182/blood-2009-10-250217
- Li X, Xu F, Chang C, Byon J, Papayannopoulou T, Deeg HJ, et al. Transcriptional regulation of miR-10a/b by TWIST-1 in myelodysplastic syndromes. Haematologica 2013;98(3):414–9. doi: 10.3324/haematol.2012.071753
- Coutinho DF, Monte-Mor BC, Vianna DT, Rouxinol ST, Batalha AB, Bueno AP, et al. TET2 expression level and 5-hydroxymethylcytosine are decreased in refractory cytopenia of childhood. Leukemia Res. 2015;39(10):1103–8. doi: 10.1016/j.leukres.2015.07.005
- Song SJ, Ito K, Ala U, Kats L, Webster K, Sun SM, et al. The oncogenic microRNA miR-22 targets the TET2 tumor suppressor to promote hematopoietic stem cell self-renewal and transformation. Cell Stem Cell 2013;13(1):87–101. doi: 10.1016/j.stem.2013.06.003
- Bhagat TD, Zhou L, Sokol L, Kessel R, Caceres G, Gundabolu K, et al. miR-21 mediates hematopoietic suppression in MDS by activating TGF-β signaling. Blood 2013;121(15):2875–81. doi: 10.1182/blood-2011-12-397067
- Drexler HG, Dirks WG, Macleod RA. Many are called MDS cell lines: one is chosen. Leukemia Res. 2009;33(8):1011–6. doi: 10.1016/j.leukres.2009.03.005
- Gañán-Gómez I, Wei Y, Yang H, Pierce S, Bueso-Ramos C, Calin G, et al. Overexpression of miR-125a in myelodysplastic syndrome CD34+ cells modulates NF-κB activation and enhances erythroid differentiation arrest. PLoS ONE 2014;9(4):e93404. doi: 10.1371/journal.pone.0093404
- Jongen-Lavrencic M, Sun SM, Dijkstra MK, Valk PJ, Löwenberg B. MicroRNA expression profiling in relation to the genetic heterogeneity of acute myeloid leukemia. Blood 2008;111(10):5078–85. doi: 10.1182/blood-2008-01-133355
- Bousquet M, Quelen C, Rosati R, Mansat-De Mas V, La Starza R, Bastard C, et al. Myeloid cell differentiation arrest by miR-125b-1 in myelodysplasic syndrome and acute myeloid leukemia with the t(2;11)(p21;q23) translocation. J Exp Med. 2008;205(11):2499–506. doi: 10.1084/jem.20080285
- O'Connell RM, Chaudhuri AA, Rao DS, Gibson WS, Balazs AB, Baltimore D. MicroRNAs enriched in hematopoietic stem cells differentially regulate long-term hematopoietic output. Proc Natl Acad Sci. 2010;107(32):14235–40. doi: 10.1073/pnas.1009798107
- Emmrich S, Rasche M, Schoning J, Reimer C, Keihani S, Maroz A, et al. miR-99a/100∼125b tricistrons regulate hematopoietic stem and progenitor cell homeostasis by shifting the balance between TGFβ and Wnt signaling. Genes Dev. 2014;28(8):858–74. doi: 10.1101/gad.233791.113
- Bousquet M, Harris MH, Zhou B, Lodish HF. MicroRNA miR-125b causes leukemia. Proc Natl Acad Sci. 2010;107(50):21558–63. doi: 10.1073/pnas.1016611107
- Chaudhuri AA, So AY, Mehta A, Minisandram A, Sinha N, Jonsson VD, et al. Oncomir miR-125b regulates hematopoiesis by targeting the gene Lin28A. Proc Natl Acad Sci. 2012;109(11):4233–8. doi: 10.1073/pnas.1200677109
- Bousquet M, Nguyen D, Chen C, Shields L, Lodish HF. MicroRNA-125b transforms myeloid cell lines by repressing multiple mRNA. Haematologica 2012;97(11):1713–21. doi: 10.3324/haematol.2011.061515
- Gerrits A, Walasek MA, Olthof S, Weersing E, Ritsema M, Zwart E, et al. Genetic screen identifies microRNA cluster 99b/let-7e/125a as a regulator of primitive hematopoietic cells. Blood 2012;119(2):377–87. doi: 10.1182/blood-2011-01-331686
- Guo S, Bai H, Megyola CM, Halene S, Krause DS, Scadden DT, et al. Complex oncogene dependence in microRNA-125a–induced myeloproliferative neoplasms. Proc Natl Acad Sci. 2012;109(41):16636–41. doi: 10.1073/pnas.1213196109
- Pigazzi M, Manara E, Bresolin S, Tregnago C, Beghin A, Baron E, et al. MicroRNA-34b promoter hypermethylation induces CREB overexpression and contributes to myeloid transformation. Haematologica 2013;98(4):602–10. doi: 10.3324/haematol.2012.070664
- Buonamici S, Li D, Chi Y, Zhao R, Wang X, Brace L, et al. EVI1 induces myelodysplastic syndrome in mice. J Clin Invest. 2004;114(5):713–19. doi: 10.1172/JCI21716
- Dickstein J, Senyuk V, Premanand K, Laricchia-Robbio L, Xu P, Cattaneo F, et al. Methylation and silencing of miRNA-124 by EVI1 and self-renewal exhaustion of hematopoietic stem cells in murine myelodysplastic syndrome. Proc Natl Acad Sci. 2010;107(21):9783–8. doi: 10.1073/pnas.1004297107
- Pozdnyakova O, Miron PM, Tang G, Walter O, Raza A, Woda B, et al. Cytogenetic abnormalities in a series of 1029 patients with primary myelodysplastic syndromes: a report from the US with a focus on some undefined single chromosomal abnormalities. Cancer 2008;113(12):3331–40. doi: 10.1002/cncr.23977
- Hussein K, Theophile K, Busche G, Schlegelberger B, Gohring G, Kreipe H, et al. Aberrant microRNA expression pattern in myelodysplastic bone marrow cells. Leukemia Res. 2010;34(9):1169–74. doi: 10.1016/j.leukres.2010.04.012
- Davis-Dusenbery BN, Hata A. MicroRNA in cancer: the involvement of aberrant MicroRNA biogenesis regulatory pathways. Genes Cancer 2010;1(11):1100–14. doi: 10.1177/1947601910396213
- Siomi H, Siomi MC. Posttranscriptional regulation of microRNA biogenesis in animals. Mol Cell. 2010;38(3):323–32. doi: 10.1016/j.molcel.2010.03.013
- Ma L, Teruya-Feldstein J, Weinberg RA. Tumour invasion and metastasis initiated by microRNA-10b in breast cancer. Nature 2007;449(7163):682–8. doi: 10.1038/nature06174
- Hermeking H. p53 enters the microRNA world. Cancer Cell 2007;12(5):414–8. doi: 10.1016/j.ccr.2007.10.028
- Deng H, Guo Y, Song H, Xiao B, Sun W, Liu Z, et al. MicroRNA-195 and microRNA-378 mediate tumor growth suppression by epigenetical regulation in gastric cancer. Gene 2013;518(2):351–9. doi: 10.1016/j.gene.2012.12.103
- Erdogan B, Bosompem A, Peng D, Han L, Smith E, Kennedy ME, et al. Methylation of promoters of microRNAs and their host genes in myelodysplastic syndromes. Leukemia Lymphoma 2013;54(12):2720–7. doi: 10.3109/10428194.2013.790542
- Xia Q, Hu J, Meng YS. Abnormal expression of microRNA-124 in patients with leukemia or myelodysplastic syndrome and its significance. Zhongguo shi yan xue ye xue za zhi/Zhongguo bing li sheng li xue hui = J Exp Hematol/Chin Assoc Pathophysiol. 2012;20(2):358–61.
- Thol F, Scherr M, Kirchner A, Shahswar R, Battmer K, Kade S, et al. Clinical and functional implications of microRNA mutations in a cohort of 935 patients with myelodysplastic syndromes and acute myeloid leukemia. Haematologica 2015;100(4):e122–4. doi: 10.3324/haematol.2014.120345
- Greenberg P, Cox C, LeBeau MM, Fenaux P, Morel P, Sanz G, et al. International scoring system for evaluating prognosis in myelodysplastic syndromes. Blood 1997;89(6):2079–88.
- Kantarjian H, O'Brien S, Ravandi F, Cortes J, Shan J, Bennett JM, et al. Proposal for a new risk model in myelodysplastic syndrome that accounts for events not considered in the original International Prognostic Scoring System. Cancer 2008;113(6):1351–61. doi: 10.1002/cncr.23697
- Zuo Z, Calin GA, de Paula HM, Medeiros LJ, Fernandez MH, Shimizu M, et al. Circulating microRNAs let-7a and miR-16 predict progression-free survival and overall survival in patients with myelodysplastic syndrome. Blood 2011;118(2):413–5. doi: 10.1182/blood-2011-01-330704
- Choi JS, Nam MH, Yoon SY, Kang SH. MicroRNA-194-5p could serve as a diagnostic and prognostic biomarker in myelodysplastic syndromes. Leukemia Res. 2015;39(7):763–8. doi: 10.1016/j.leukres.2015.04.013
- Kim Y, Cheong JW, Kim YK, Eom JI, Jeung HK, Kim SJ, et al. Serum microRNA-21 as a potential biomarker for response to hypomethylating agents in myelodysplastic syndromes. PLoS ONE 2014;9(2):e86933. doi: 10.1371/journal.pone.0086933
- Krejcik Z, Belickova M, Hrustincova A, Klema J, Zemanova Z, Michalova K, et al. Aberrant expression of the microRNA cluster in 14q32 is associated with del(5q) myelodysplastic syndrome and lenalidomide treatment. Cancer Genet. 2015;208(4):156–61. doi: 10.1016/j.cancergen.2015.03.003
- Cho WC. Great potential of miRNAs as predictive and prognostic markers for cancer. Expert Rev Mol Diagn. 2012;12:315–18. doi: 10.1586/erm.12.21